17
Stereotactic Radiosurgery for Ocular Disorders
Roman Liščák and Gabriela Šimonová
Due to its underlying radiobiological principles, radiosurgery is generally used to treat small-volume lesions, and volumes of ocular disorders usually fall within the volume constraints of radiosurgery. The first radiosurgical experience with ophthalmologic indications involved the treatment of patients suffering from uveal melanomas.1–7,21 However, it would appear that the potential of radiosurgery to provide effective treatment for ophthalmologic indications is broader. In addition to uveal melanomas, the current spectrum of ocular indications includes eye metastases, advanced glaucoma, age-related macular degeneration (ARMD), hemangioblastoma, pseudotumors, and vegetative pain.
Radiosurgery of ocular disorders necessitates a close co-operation with ophthalmologists. This multidisciplinary approach is critical for patient selection, follow-up, and treatment.
Technical Issues Specific to Stereotactic Radiosurgery for Ocular Disorders
The Leksell Gamma Knife (Elekta Instruments AB, Stockholm, Sweden) was originally designed to treat deep brain targets, and stereotactic coordinates are derived from the position of the coordinate head frame, which is rigidly fixed to the skull. Proper eye fixation or an eye motion-monitoring system is required because the eye can move during the treatment procedure. Very eccentric target volume location can also cause some inaccuracies in the treatment-planning calculations, including relative dose distribution and absolute dose calculations. Finally, there may be some technical inconveniences in treating patients due to limitations in the range of the coordinate system in this eccentric target location. Despite these difficulties, radiosurgery is an attractive alternative treatment for ophthalmic lesions mainly because of its accurate and precise delivery of high conformal doses to the treatment target.
Eye Fixation
Several different eye immobilization systems and techniques have been described in the literature.1,8–15 Tokuuye et al described a mask fixation technique with a plastic mold gently pressed down over the orbit to restrict ocular movements.14 Zehetmayer et al reported on a suction immobilization technique for radiosurgery of intracranial malignancies using the Gamma Knife.15 Langmann et al used a fixation method of the globe by means of a retrobulbar anesthetic block for eye treatments using the Gamma Knife.1 In the Prague center, the treated eye was fixed under a retrobulbar local anesthesia by suturing two rectus eye muscles to the stereotactic frame; this procedure was done by an ophthalmologist. To ensure adequate dose buildup and homogeneous propagation of radiation between the tissues and the air, a partial tarsorrhaphy was performed, and a plastic cover filled with tissue-equivalent gel was used (Fig. 17.1). This simultaneously acted as protection for the cornea. This eye fixation technique showed very good stability and rigidity in time. Having the patient in a prone versus supine position had no effect on stereotactic target and eye structure localization.11,12
Active eye immobilization techniques, where the patient controls the eye position, for example, by fixating on a light source, have been described for proton/helium ion beam therapy and also for linear accelerator (linac)–based stereotactic radiotherapy of malignant tumors.8,10 For proton/helium ion beam therapy, a surgical intervention is performed to position tantalum clips at the border of the visible tumor under diaphanoscopical control. These clips are used during treatment planning to define the target volume and to verify the position of the tumor during treatment delivery by using x-rays.9 Petersch et al described a noninvasive eye fixation system for the application of linac-based stereotactic radiotherapy in the treatment of uveal melanoma.13 The computer-controlled eye-monitoring system is based on the patient’s fixation on a point of light. When the eye position exceeds the preset geometrical limits to that point of light, the system automatically initiates a cessation of the radiation beam until the eye is repositioned to the proper alignment.
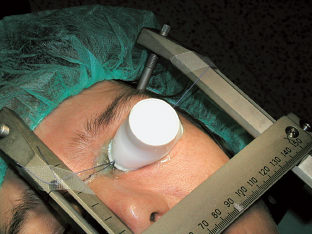
Fig. 17.1 Fixation of the eye.
Accuracy of Stereotactic Imaging
Magnetic resonance imaging (MRI) is the preferred imaging modality for radiosurgery. It offers more detailed and artifact-free information than computed tomography (CT). However, whereas CT is supposed to be free of geometric distortions, MRI may produce some geometric image distortions significant for radiosurgery treatment planning. Generally, MRI geometric distortion is mainly a function of the particular MRI scanner employed, MRI sequence used, image slice orientation, position in the imaged object, and material and geometric parameters of the stereotactic frame.16,17 The issue of image geometric distortion is even more crucial in the case of eye imaging when a target and other structures of interest are in a very frontal location. It is essential to check the extent of geometric distortions for each MRI scanner and sequence employed for ocular imaging.12
Dosimetry and Treatment Planning
Due to very eccentric target volume location, some inaccuracies in the treatment-planning calculations, including relative dose distributions and absolute dose calculations (calculation of treatment time or monitor units), can be expected. This issue is even more prominent for surface structures of the eye (e.g., eye lens, eyelid, and cornea) than for target volumes itself.
Petersch et al reported excellent agreement between actual measurements and the treatment-planning system calculations for uveal melanomas at a depth of 15 to 20 mm.13 However, at small depths (<10 mm), the treatment-planning system cannot model the influence of absorption in the eye motion-monitoring device accurately if the beam passed perpendicularly through it. Consequently, as a means of reducing dosimetric errors, avoidance of frontal beams during radiosurgery to ocular lesions has been suggested.
Detailed dosimetric measurements were also performed at the Prague Na Homolce Hospital. In this case, treatment-planning system calculations of target volume relative dose distributions and absolute dose calculations in different depths were compared with experimental measurements.12 Various treatment plans for different ophthalmic indications (e.g., uveal melanoma, glaucoma, and retinoblastoma) calculated for the Gamma Knife were evaluated. Absolute doses measured by pinpoint ion chamber at depths of 10 mm and deeper showed relatively good agreement with the treatment-planning system calculated data. Absolute doses delivered during head phantom irradiation were systematically lower than calculated ones, usually within 5% or even within 3%. Absolute doses measured by pinpoint ion chamber at depths < 10 mm showed a relatively large deviation compared with the treatment-planning system’s calculated data. In this situation, absolute doses delivered during phantom irradiation were systematically lower by 15 to 20%. To improve on inaccuracies in absolute dose calculations in surface structures, proper tissue-equivalent built-up material attached to the treated eye was recommended (Fig. 17.1). Such material should reduce discrepancies between the planned and the delivered radiation dosimetry. Preliminary measurements demonstrated that calculation errors should not exceed more than 10% even at surface structures when built-up material is used. Relative dose distributions measured by polymer gel dosimetry in the region of the target volumes were in good agreement with the Leksell Gamma Plan (Elekta).
Because of the marginal position of the eye, an extreme gamma angle has to be used to avoid collision of the skull with the collimator helmet of the Gamma Knife model B or C. Patients usually do no tolerate backward bending of the head in a supine position. Therefore, the prone position was used in most cases. The treatment could not be performed for this reason on a small number of patients suffering from rigid kyphosis or Bekhterev disease.
Depending on the patient’s head shape and volume, it may be difficult to adjust properly the frame position to reach all Leksell stereotactic coordinates. Specifically, the Y (posterior-anterior) coordinate can exceed limits engraved on the commercially available Leksell stereotactic frame. Because of this, a special Y coordinate “extender” was developed at Na Homolce Hospital to be able to treat Y coordinates exceeding standard Gamma Knife limits (Fig. 17.2). Fortunately, the problems of collisions and coordinate limits have largely been overcome by the enlarged space of the collimator helmet in the latest model of the Leksell Gamma Knife Perfexion (Elekta).
Specific Disorders
Uveal Melanoma
Natural History and Clinical Presentation
Uveal melanoma represents the most common primary malignant tumor of the eye in adults. There is a peak incidence between 55 and 70 years of age, with a prevalence of 6 or 7 cases per million people per year. The incidence is rare in patients younger than 20 years of age. Approximately 50% of patients with diagnosed melanomas of the choroid or ciliary body will die from this tumor within 15 years.18,19
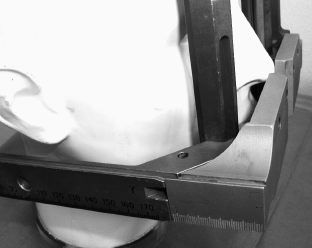
Fig. 17.2 Triangle adapter to expand the stereotactic space Y coordinate.
Uveal melanomas are neoplasms arising from the uveal tract that includes the iris, ciliary body, and choroid. The uveal tract is a vascular structure with no lymphatic drainage. Lymphatic node involvement (preauricular, submandibular, and cervical nodes) can be diagnosed on rare occasions when subconjunctival extension of the primary tumor has also been observed. The major routes of extension are local growth and hematogenous spread. The common sites of hematologic dissemination are the liver, lung, and brain. Liver metastases are diagnosed in two thirds of patients with disease dissemination.
Wide variations in the grading of malignancy can be observed, from relatively benign types with several years of survival without dissemination, to other tumors with fast multiorgan dissemination, which can lead to the patient’s death within a few months. Tumor growth is also influenced by the potential cell doubling time, which varies widely from 60 to 350 days (median ~70 days).20 A longer doubling time as compared with a significant proportion of other tumors can explain the relatively long survival of these patients and also the relatively slow tumor response after irradiation.
Iris melanomas (incidence ~10%) are single or multiple elevated lesions with associated changes of iris color. These types of melanomas are much more benign, smaller in size, readily visible, and diagnosed early. It can be difficult to assess the potential of the malignant cells, even in those with documented growth, because iris nevi may also grow. For this reason, local resection is usually effective whenever it is required.
Choroidal melanomas typically (but with some variation) grow in nodular form and in cases of continuing growth cause many tumors to break through the Bruch membrane (a base membrane-connective tissue complex lying between the retina pigment epithelium and the choriocapillaris). When the melanomas break through the Bruch membrane, they extend into the subsensory retinal space, which gives them a mushroom or collar-button shape. These characteristics, as well as including the detachment of the retina, are typical for uveal melanomas. This type of tumor growth is sometimes accompanied by vitreous hemorrhage with symptoms of sudden visual loss.
Melanomas involving the ciliary body are rare, carry a poor prognosis, and are often diagnosed late. Melanomas can also have diffuse, infiltrating growth to the ciliary body and a pattern called ring melanoma. These tumors are difficult to diagnosis because they are accompanied by relatively little visible tumor mass effect, and they occur in the diagnostically “silent” eye area. Any extrascleral extension of the tumor usually confers a poor prognosis. The diffusely growing melanomas are usually more aggressive, with more malignant cell types.
Melanoma of the ciliary body and choroid have typical clinical features, and the correct diagnosis for a majority of patients can be made by taking a history and performing a complete ocular examination. The ophthalmologic examination includes external ocular examination, indirect ophthalmoscopy, fluorescein angiography, and ultrasonography, which plays a basic role at the time of diagnosis. The ophthalmologic examination also plays a role in follow-up and can be used to obtain precise measurement of the tumor base and height. The height of the tumor in particular represents one of the most important factors influencing the treatment decision, and it is used during follow-up to evaluate the tumor’s response.
Results of Radiosurgery
The first experiments with radiosurgery for the treatment of uveal melanomas were conducted using the rabbit eye melanoma model. The reported tumor lethal dose in this experiment ranged from 60 to 90 Gy applied in a single session.4 Several authors reported the results of Gamma Knife radiosurgery in uveal melanomas, and some observed relatively high radiation-related toxicity.1–3,5,6,21–24 The minimum effective dose for long-lasting local tumor control, as well as the tolerance doses for critical ocular structures, is not yet well defined.
Our own experience represents a group of 126 patients with uveal melanoma treated using the Gamma Knife over a period of 8 years. The LENT SOMA (Late Effects Normal Tissues, subjective, objective, management, and analytic) scoring system25 was used to measure the radiation side effects (Table 17.1). The tumor marginal dose for radiosurgery ranged from 35 to 45 Gy, with a median dose of 41 Gy in our series.
Tumor regression was defined as a decrease in tumor height registered by ultrasonography scans and by control MRI. The tumor regression was achieved in 70% of patients. The maximum local effect has been recorded within 24 months after radiosurgery. Patients with tumor growth progression or without any response 30 months after treatment were candidates for re-treatment by radiosurgery, and patients with a tumor height >10 mm or extrascleral growth were indicated for enucleation (5% in our series).
Complications from Radiosurgery
The most common late toxicity complications for all types of irradiation are retinopathy, cataract, secondary glaucoma, and optic neuropathy.6,24,26–30 The most common cause of the toxicity-related enucleation is secondary neovascular glaucoma.28,31 Secondary glaucoma is typically a late complication, and its incidence increases with longer follow-up. Although the risk of enucleation from this reason is ~6% for a 2-year follow-up period after radiosurgery, it increases to 19% with 8 years of follow-up.31–35 Secondary glaucoma often accompanies uveal melanoma already before the treatment. The question remains: how much of the treatment itself contributes to its development or accentuation? Differentiation of the natural development of this complication from accentuation of secondary glaucoma caused by radiosurgery is not possible at present.
A study with proton beam treatment identified localization of the tumor in the region of the ciliary body or fovea and a height of the tumor >8 mm as risk factors for subsequent enucleation.31 Some observations were made on patients after radiosurgery who had their eye secondarily enucleated. Eyes with secondary enucleations presented an initial tumor height >9 mm. The second frequent reason for enucleation was the origin of the tumor in the region of the ciliary body.24
An analysis of late toxicity of 126 patients of our series recorded severe grade 3–4 retinopathy in 15% of treated patients, radiation-related late toxicity grade 3–4 in the lens was observed in 26%, optic neuropathy in 9%, and secondary glaucoma in 18% of cases. Eleven percent of patients were enucleated for worsening glaucoma during the first 2 years after radiosurgery. The median time to occurrence of secondary neovascular glaucoma was 18 months, so a higher incidence of enucleation during a longer follow-up cannot be excluded. The worsening of useful vision caused by radiation-related toxicity during 3 years after radiosurgery was observed in 34% of patients and was due to tumor progression in 3%, optic nerve neuropathy in 8%, retinopathy in 9%, and neovascular glaucoma in 14%.
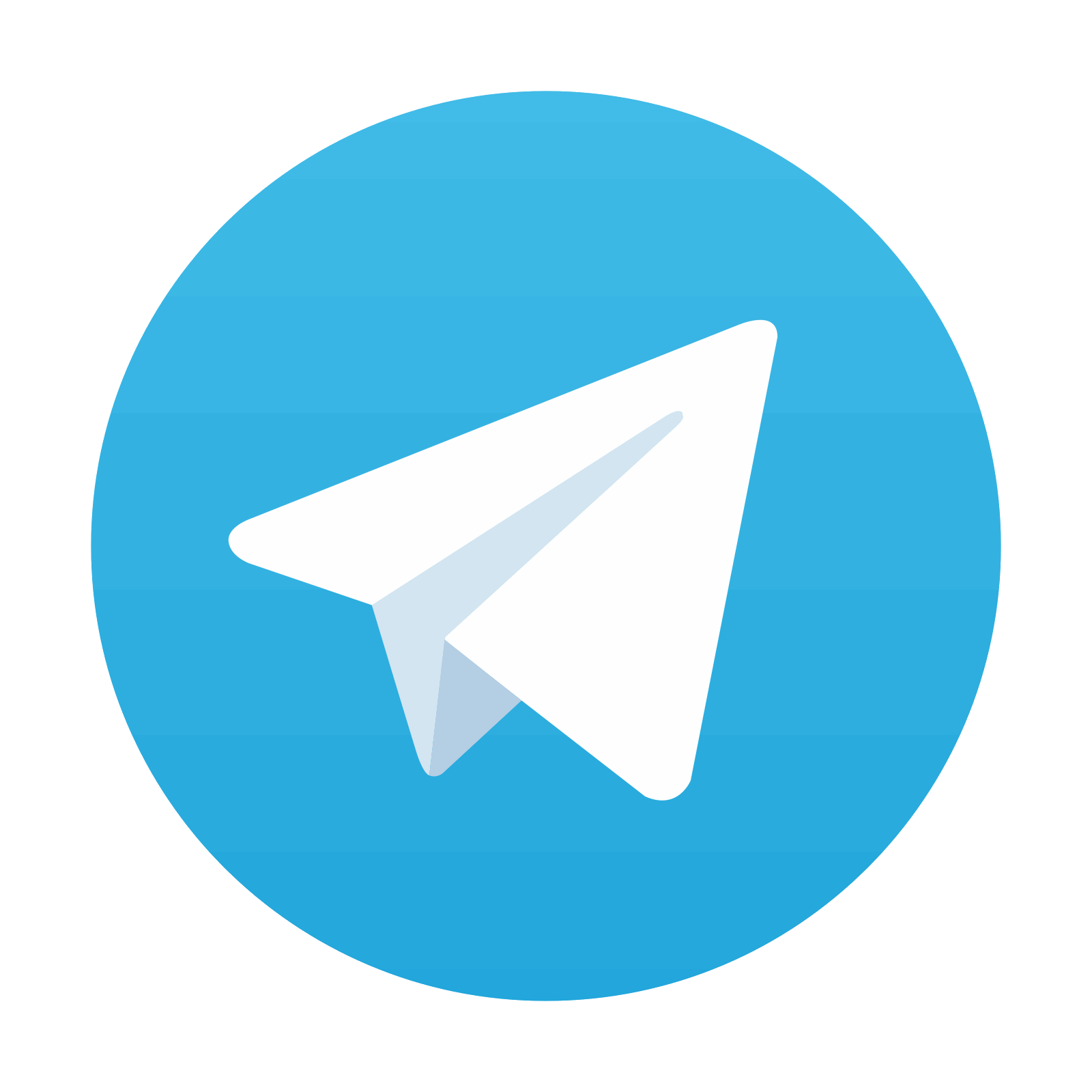
Stay updated, free articles. Join our Telegram channel

Full access? Get Clinical Tree
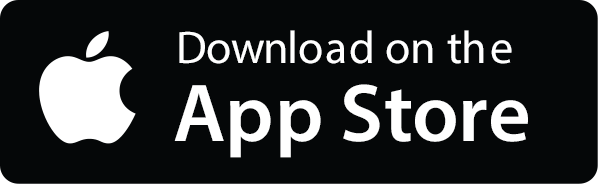
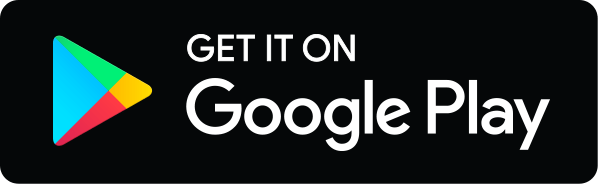