Terminology and definitions
Single-fraction stereotactic radiosurgery (SRS)
Single-fraction SRS is a specialized radiation therapy technique that delivers a single high dose of radiation to the tumor (usually <3 cm in maximum dimension , ). Multiple coplanar and noncoplanar radiation beams converge on the target lesion, with the marginal dose delivered in a single session usually ranging from 11 to 70 Gy, based on the indication.
Fractionated stereotactic radiotherapy (SRT)
Fractionated SRT delivers multiple, smaller doses of radiation therapy over time, up to a maximum of 5 fractions. This is usually delivered to moderate-large sized lesions (usually >3 cm) in close proximity to critical structures such as the brainstem, optic nerves, and optic chiasm to reduce the risk of long-term radiation-induced toxicity. A 1- to 2-week schedule is most typical.
Rationale for using particle therapy in stereotactic radiosurgery
Particle therapies have dosimetric advantages when compared to photon therapy because of their Bragg-peak characteristics, lower entry, and minimal exit dose. When compared to conventionally-fractionated photon therapy, this results in improved local tumor control, less damage to healthy tissue, less acute and late toxicity, and a lower risk of secondary malignancy. Particle radiosurgery could hypothetically allow for dose escalation through better dose sparing of critical structures such as the brainstem, optic nerve, and optic chiasm when compared to photon radiosurgery. Even though photon-based methods have proven to be highly effective, particle therapy is being investigated as a way of further improving dose precision and radiobiologic effects, especially in reirradiation cases.
Particles and systems used for particle stereotactic radiosurgery/radiotherapy
Various types of particles are being explored in the setting of SRS/SRT.
Protons
The use of proton beam therapy is currently available for SRS/SRT in a limited number of centers, with this number steadily increasing over the last few years. Protons interact with matter by coulomb collisions between electrons and nuclei, leading to bremsstrahlung radiation loss and nuclear reactions. The characteristic energy distribution of high-energy protons (i.e., Bragg peak, beyond which no primary proton dose is deposited) could allow for better dose sparing of normal tissue using fewer treatment beams (typically 3–4 for SRS applications).
Two modalities of proton beam delivery are available for SRS applications. Passive scattering proton systems use a single- or dual-stage scattering system to generate a wide proton field that is then trimmed laterally by apertures and distally by beam-specific compensators. These beam-shaping devices can either be patient-specific or standard in shape, depending on the complexity and size of the target. Apertures allow for the delivery of proton beams with a penumbra equivalent or superior to photons at the expense of a loss in dose delivery efficiency. Pencil beam scanning (PBS) proton delivery is a newer technology that generates highly conformal dose distributions by delivering a particular pattern of spot positions with varying energies and intensities. PBS has the advantage of efficiently generating conformal dose distributions around complex targets (i.e., arteriovenous malformations); however, this can be at the expense of beam penumbra, as the proton spot is inherently larger for lower energies that are typically employed in SRS applications.
Patients can be treated with fixed or gantry-based proton delivery systems, utilizing a frame, bite block, or mask immobilization. The Massachusetts General Hospital (MGH) proton therapy center specifically designed the Stereotactic Alignment for Radiosurgery (STAR) system to allow for more flexible and precise patient positioning. The patient is immobilized by a head frame that is connected to a couch that can be rotated in relation to a fixed beam portal. The patient immobilization during proton SRS was achieved using a Gill-Thomas-Cosman frame.
Carbon ions (and other heavy charged particles)
Carbon ions deliver a narrower Bragg peak with sharper penumbra as compared to protons but generate a fragmentation tail, which is a low-dose, high–linear energy transfer (LET) region distal to the Bragg Peak. Since carbon ions have a larger mass compared to protons, there is decreased beam scatter, resulting in a sharper dose distribution. Carbon ions also have a greater relative biological effectiveness (RBE), giving them an advantage in the treatment of hypoxic and radio-resistant cancers; however, uncertainties with biological modeling mean that the impact on normal critical structures is unclear. With more than 12 operating centers and over 28,000 patients treated to date, carbon ion facilities continue to expand in number and experience.
Protons and carbon ions are used in most heavy-charged particle therapies; however, there is a growing interest in other heavy-charged particles, such as helium. Patients were initially treated with helium ions using passive beam delivery. In terms of physical properties, they generate less projectile fragmentation than carbon ions and reduced lateral beam spread than protons. Furthermore, helium ions have less variation in RBE between tumor and normal tissue than heavier ions, making biological dose estimates less susceptible to such uncertainties.
Particles in research phase (boron neutron capture therapy [BNCT] & very high-energy electrons [VHEE])
BNCT is a technique for delivering tumor cell-specific radiotherapy. It uses relatively tumor-specific agents to deliver boron-10 to the tumor. Upon interaction with the neutron beam, boron-10 is converted to lithium-7 intratumorally, and an alpha particle is emitted. The short path length of the alpha particle preferentially damages tissue localized to the site of interaction. Therefore, localization of the boron-10 compound is key to the success of this therapy. Though it represents a promising treatment modality, its application in SRS/SRT is yet to be determined. However, recent technological advances have led to novel system designs, and unique targeting compounds are in the testing phase.
VHEEs are typically generated at energies between 100 to 250 MeV. Higher-energy electrons penetrate deeper and provide a sharper transverse penumbra, possibly allowing for the treatment of deep-seated tumors. VHEE beams have the ability to deliver extremely high dose rates, which may be consistent with the generation of the “FLASH effect.” However, its clinical application to SRS/SRT is yet to be explored.
Current indications and overview of top-line results using particle radiosurgery
Brain metastasis
Data on the use of particle radiosurgery in brain metastasis are limited. Atkins et al. published the first and largest series of proton SRS experiences for the treatment of single or multiple brain metastases in 370 patients. The 90% isodose line received a median prescription dose of 17.3 Gy relative biological effectiveness (GyRBE). At 12 months, the local and distant failure rates were 8.5% (95% confidence interval [CI], 6.7%–10.6%) and 48.2% (95% CI, 43.0%–53.2%), respectively. At 6 and 12 months, overall survival was 76.0% (95% CI, 71.3% –80.0%) and 51.5% (95% CI, 46.3%–56.5%), respectively. Grades 1–3 acute toxicities were seen in 40.5% of patients. The rate of radiation necrosis was 3.6% at 12 months, and target volume was found to have a significant association with this risk. This experience suggests that proton SRS is comparable to photon SRS for brain metastases. Future strategies must look at determining which subset of patients will benefit the most from integral dose reduction, especially in patients treated for multiple metastases.
Arteriovenous malformation (AVM)
Kjellberg et al. reported the first study of proton SRS for AVMs in 75 patients, with complete obliteration in 20% and a size reduction of approximately 50% in nearly 56% of patients. The original beam delivery used two opposed, uncollimated beams. Seizures, headaches, and neurologic deficits were improved in the majority of cases. Barker et al. reported a large retrospective study of 1250 AVM patients treated with proton SRS. The median radiation dose and treatment volumes were 10.5 Gy and 33.7 cc, respectively. The 10-year actuarial complication rate was estimated to be 4.5%, which is very favorable compared to the photon SRS experience. Seifert et al. reported that long-term clinical and radiological outcomes were linked to AVM size and the Spetzler-Martin scale. Obliteration was seen only in lesions smaller than 3 cm. Only one-third of patients showed improvement in clinical symptoms of AVMs between 3 to 6 cm in diameter. Nearly 28.6% of patients reported worsening symptoms. Vernimmen et al. found the obliteration rate to be 67% with volumes less than 14 cc and 43% with volumes greater than or equal to 14 cc. They concluded that proton SRS is a safe modality for treating large AVMs.
Steinberg et al. published a retrospective study of 86 AVM patients treated with helium ions. The 3-year obliteration rate was 100% for lesions smaller than 4 cc, 95% for those between 4 to 25 cc, and 70% for those larger than 25 cc.
Pituitary adenoma
Particle SRS has been predominantly used in the treatment of patients with hormone-secreting pituitary adenomas. In one series, single-fraction proton-beam SRS was used to treat acromegaly with a median dose of 20 Gy equivalent (GyE). At least partial hormonal response was achieved in 95% and complete response in 59% at 6 years. Similarly, Petit et al. reported a complete hormonal response in 59% of patients; however, eight patients experienced new hypopituitarism. The same group reported outcomes of 38 patients with persistent Cushing disease treated with proton-beam SRS after surgery. There was complete response in 58% of patients after 5 years of follow-up; however, 52% of patients had a new pituitary deficiency. Although the target volumes treated with proton SRS are generally larger than those treated with photon SRS, the results appear comparable.
Vestibular schwannoma
Harsh et al. reported the initial experience with proton-beam SRS treatment of vestibular schwannomas, which was later updated. , A total of 88 patients received a median dose of 12 GyE (range 10–18 GyE). At 2 and 5 years, the local control rates were 95.3% and 93.6%, respectively. The actuarial 5-year radiological reduction and facial and trigeminal nerve function preservation rates were 94.7%, 91.1%, and 89.4%, respectively. These results compare favorably with photon SRS.
Meningioma
Halasz et al. reported the first series of proton SRS for the treatment of benign meningiomas. Tumors less than 4 cm in diameter were included, and a median dose of 13 GyE (range 10–15.5 GyE) was delivered to the 90% isodose line. The 3-year tumor control rate was 94% with improvement of symptoms seen in 47% of patients. No new cranial nerve deficits were observed, and the rate of permanent adverse effects after SRS was only 5.9%. Proton SRS had a low incidence of treatment-related morbidity and was effective in the treatment of small, benign meningiomas.
Complications of particle radiosurgery
Limited data for the utilization of particle SRS in intracranial tumors exist, with the vast majority composed of single-institution retrospective studies. The complications reported in these studies are comparable to those seen with photon SRS. The improved dose conformity of particle SRS comes at the cost of increased sensitivity to range uncertainties. Robust planning and optimization techniques can help reduce such dosimetric uncertainties; however, particle therapy can also have various effects on the tumor and underlying normal tissues from a radiobiological perspective. As such, consideration of LET and varying RBE during plan optimization is also recommended to minimize the impact on nearby at-risk organs. Future developments in image guidance, beam delivery, and modeling are expected to widen the clinical deployment of particle-based radiosurgery, further demonstrating the safety and efficacy of this treatment technique. In particular, the recent development of proton arc therapy could open new opportunities to further improve proton SRS.
References
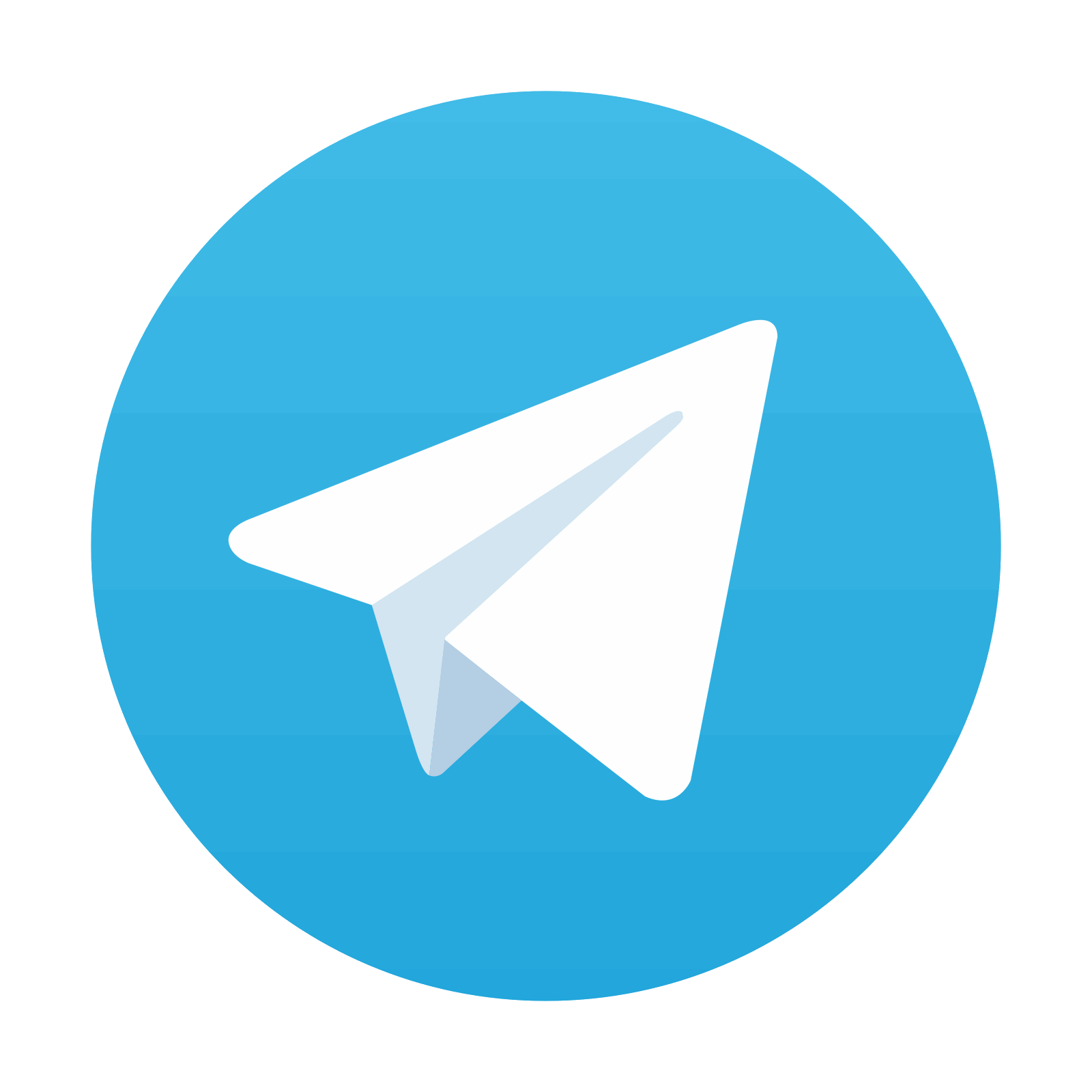
Stay updated, free articles. Join our Telegram channel

Full access? Get Clinical Tree
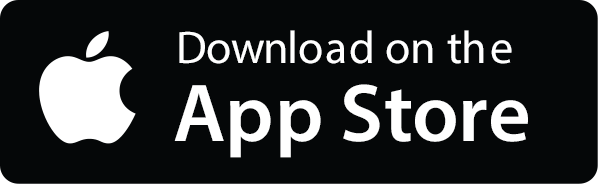
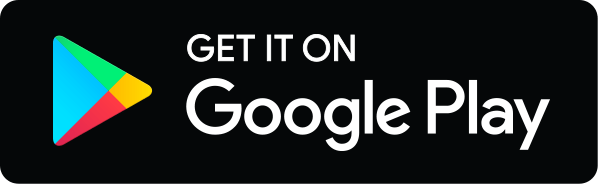
