and Rakhi Kaila2
(1)
School of Physics, University of New South Wales, Sydney, NSW, Australia
(2)
School of Medicine, University of New South Wales, Sydney, NSW, Australia
Abstract
Animal and plant life to some extent is replica of the life inside earth itself. Earth lives and so do we. Inside earth’s crust there are elements like iron, copper, silicon, sodium, magnesium, uranium, etc., etc. in the form of stable and not so stable compounds. We mine them, process them and use them, in various technologies, including nuclear energy for peaceful, and sometimes not so peaceful purposes. There are circulating molten solid currents, due to high pressures and temperatures, inside earth’s crust, which give rise to the magnetism inside and outside earth. We are quite familiar with the N–S poles of the giant magnet, in the core of the earth. It provided the source of navigation and discovery of unknown places on the surface of earth, for centuries, to some clever people. The N–S magnetic axis of the earth is not along the geographic N–S poles of the earth. The N–S magnetic axis of the earth is not fixed in time either. It reorients itself on daily, monthly and yearly basis. Why it does so? One scientific reason we know of is that the earth is bombarded by heavy mass of charged particles, on regular basis, from Sun. The mother nature’s magnetic field on the surface of earth which is of the order of only milli to micro Tesla, protects our body from harmful effects of the radiation, we receive on earth on daily basis from the surrounding universe. The radiation e.g., the solar radiation, provides us life on earth, as well, on daily basis. The magnetic field on the surface of earth, changes in a systematic manner, with distance. One can see magnetic field gradient present on the earth and it varies slowly, and is stronger in one direction than the other. Our body is exposed to it all the time. Is it shear coincidence that we use magnetic field gradients of the order mT/meter, close to present on earth, to produce MRI pictures in human body? May be. May be not. Some researchers have used earth’s field as the source of magnetic field to perform MRI experiments. This curiosity has produced some interesting results. That is how the development of the MRI technology we use today was stated half a century ago and is still evolving. This applies to every science-based technology we use.
How and when life started on earth is not known. But its evolution is still going on. There are means available now i.e., intelligent manpower, technologies, nature-science interactions happening-rapidly provoking us round the clock, to guide us into the future. To sit back and think that, we have got what we wanted, why bother any more, is a blitz of ignorance.
Animal and plant life to some extent is replica of the life inside earth itself. Earth lives and so do we. Inside earth’s crust there are elements like iron, copper, silicon, sodium, magnesium, uranium, etc., etc. in the form of stable and not so stable compounds. We mine them, process them and use them, in various technologies, including nuclear energy for peaceful, and sometimes not so peaceful purposes. There are circulating molten solid currents, due to high pressures and temperatures, inside earth’s crust, which give rise to the magnetism inside and outside earth. We are quite familiar with the N–S poles of the giant magnet, in the core of the earth. It provided the source of navigation and discovery of unknown places on the surface of earth, for centuries, to some clever people. The N–S magnetic axis of the earth is not along the geographic N–S poles of the earth. The N–S magnetic axis of the earth is not fixed in time either. It reorients itself on daily, monthly and yearly basis. Why it does so? One scientific reason we know of is that the earth is bombarded by heavy mass of charged particles, on regular basis, from Sun. The mother nature’s magnetic field on the surface of earth which is of the order of only milli to micro Tesla, protects our body from harmful effects of the radiation, we receive on earth on daily basis from the surrounding universe. The radiation e.g., the solar radiation, provides us life on earth, as well, on daily basis. The magnetic field on the surface of earth, changes in a systematic manner, with distance. One can see magnetic field gradient present on the earth and it varies slowly, and is stronger in one direction than the other. Our body is exposed to it all the time. Is it shear coincidence that we use magnetic field gradients of the order mT/meter, close to present on earth, to produce MRI pictures in human body? May be. May be not. Some researchers have used earth’s field as the source of magnetic field to perform MRI experiments. This curiosity has produced some interesting results. That is how the development of the MRI technology we use today was stated half a century ago and is still evolving. This applies to every science-based technology we use.
There are number of elements that are essential for higher life forms such as animal and human. They are part of, enzymes and of the proteins. The electrons are involved in the charge and oxygen transport between the neighboring macromolecules. Various charge, magnetic dipole and electric quadrupole activities are often present at the centre of action and are directly involved in the biological transformations [1–8]. Many physiological processes take place in electrolyte solution present in the medium around the cells, tissue, etc. It involves simple ions like, Na+, K+, Mg2+, Ca2+, Cl−, etc. There are important biopolymers like DNA, RNA and ionic mucopolysaccharides. One also encounters aggregates and monomers, like biological membranes, which interact strongly, through their own charge, with the ions. One finds that the ions are unevenly distributed, between intracellular and extra cellular spaces. The physical energy conservation requirements maintain concentration gradients. These are maintained through energy consuming transport e.g. metabolism. The local dipole magnetic-electric qudrupole dynamic activities create changes in the ion gradients. They become the source of the means of signal transmission. All essential elements in biological systems have at least one potentially valuable magnetic isotope. The examples are 1H, 2H, 13C, 15N, 19F, and 31P. One should remember that deep down at atomic and molecular level, there are multi-quantum interactions happening. But they are very difficult to observe with any technology currently available.
It is easy to observe the chemical shift anisotropy (CSA) effects which happen on the mm to cm scale. This is a much broader effect involving the electron clouds, belonging to local macromolecules. The electron clouds tend to smear out, over larger distance the deeper dynamic activities. They mask the magnetic-electric qudrupole interactions. In the simplest local axially asymmetric electric field environment in the medium, which has little influence on the macroscopic environment, one can approximately work out, for the I = 3/2 electric quadruple/magnetic dipole case, the longitudinal R1 = 1/T1 and the transverse R2 = T2 relaxation rates of energy transition, from a higher energy state, to the lower energy state, as follows. It is seen, that for the wider macromolecule situation, one can approximately write as, R1 ~ γ2 H02[2τc/(1 + ω2 τc 2)] and R2 ~ γ2H0 2 [8τc + {6 τc/(1 + ω2 τc 2)}], respectively. Here γ is the gyromagnetic ratio, H0 the static magnetic field applied and ω is the frequency of the incident RF radiation. The correlation time τc is a measure at the core of all quantum mechanical interactions between macromolecules. When ωτc > 1, R1 decreases with ω and as ωτc ≫ 1(very fast motion of nuclei) it becomes independent of H0, since ω = γH0. This situation is more of a representative of the free motion of ions in electrolytes. This is the region where magnetic dipole character of the nuclei dominates, the region of NMR.
The nuclei bound in a tissue, present a situation, which is quite different. Here the nuclei are surrounded by a non-uniform distribution of charges. This is the distribution of bound charges in the form of electric dipoles (one positive and one negative charge bound together) and quadrupoles (two negative and two positive charges bound together). In the quadrupole nuclei (I > 1/2), the situation is more complex. In a local region, the magnetic character of the nuclei is modulated by their overwhelming electric behavior. Now there is non-exponential relaxation of the spins, when excited by the RF pulses. And also there are second order frequency shifts in the received signals. The quadrupole relaxation is caused by the interaction of a nuclear electric quadrupole moment eQ, with the fluctuating electric field gradient, eq, at the position of the nucleus. In isotropic systems, when ωτc ≪ 1 (called as the extreme narrow condition, the nuclear magnetization will decay with a single exponent, and one can write, R1 = R2 ~ [{(2I + 3)}/{I2(2I −1)}] [{χ2}{(1 + η2/3)}τc][11]. Here χ = (e2qzz Q/h) is the nuclear quadrupole coupling constant and η = [(qxx− qyy)/qzz], is the asymmetry parameter. Here qxx, qyy, and qzz are the axial components of the electric field gradients in the x, y, and the z directions. In the biological systems, the true extreme narrowing (ωτc ≪ 1) situation is usually encountered only in the case of free hydrated ions in solution or in the case of low-molecular weight complexes.
One commonly encounters the situation where ωτc > 1. In this case the decay of the longitudinal (Mz) and transverse magnetization (Mx.y) for quadrupolar nuclei with spins I > 1 is no longer exponential. In metal ion nuclei we have ωτc ≈ 1 and also holds for the I = 3/2 quadrupolar nuclei. The decay of Mz and Mx.y will be nearly not exactly exponential. In addition to non-exponential relaxation for quadrupolar nuclei under conditions of non- extreme narrowing, the NMR signal will also be modified by the chemical shift (the smearing electron cloud around nuclei) changes, termed as ‘second-order dynamic frequency shifts’. In the limit ωτc ≫ 1, the m = 1/2 to m = −1/2 magnetic component will dominate the spectrum because it is much narrower than the other components. The intensity of this component relative to the total intensity will be 40 % for the I = 3/2 (Na) case. One can in the lowest approximation consider a tissue, containing Na as a metabolic agent, in the brain, as a single quantum (all spins being identical) ensemble of nuclei. This means basically there is no multi-quantum correlation (interaction) between the I = 3/2, sodium nuclei. They act as independent entities, just adding up to the total signal, as absorption and dispersion of energy, in the neighboring environment. A small region of interest (ROI) behaves like a dispersive medium, different sections contribute to imaging character, through different echo time (TEs). This is an oversimplified model, nevertheless a useful one to start with. In the sense of the spectroscopy of the, I = 3/2, spin ensemble, one should expect, a superposition of two components, a broadband and a narrowed component [1].
In the extreme narrowing condition, these components are degenerate in both width and absorption frequency. In the other extreme, when here is no extreme narrowing condition, this degeneracy does not result. The broad and narrow (precise) transverse magnetizations can be created by applying a resonant RF field of magnitude (amplitude) H1 and duration = (θ/γH1). Here θ is the flip angle and γ the gyromagnetic ratio (GMR) of the nucleus. The relaxation of the spins after irradiation with RF radiation is considered to be due to the interaction between the electric quadrupole moment of the nucleus and the motionally modulated electric field gradients, around the nucleus. If the interactions are of random field approximation (RFA) type, the narrow and broad components are not distinguishable. But if both the reorientational correlation time τc and the random field correlation time are much larger than the inverse of the Larmor (resonance) frequency, the time dependent character of the transverse magnetization, may be dominated by random field interaction. This may be so even if the qudrupole moment (e2qQ) of the nucleus is much larger than the magnitude of fluctuations in the local random field. Here eq is the electric field gradient and eQ the qudrupole moment of a macro-molecule.
One can use inversion recovery (application of 180° pulse) RF magnetization technique, to obtain different relaxation rates for the longitudinal Mz and transverse, Mx,y magnetizations. We notice through detailed theoretical analysis that in the longitudinal magnetization, the broad component of the spectrum decays as a single exponential, whereas the narrow component decays, nonexponetially, dominated by bi-exponential behavior. It is common knowledge in the MRI literature [6] now that the T2 (transverse relaxation time) of the intracellular sodium is shorter (~4 ms) than that of the extra cellular (~20 ms) sodium. In pure aqueous solutions on the other hand Na relaxes monoexponentially with a much longer (~50 ms) T2. It is observed [2] that in the brain tissue situation, there are electrostatic field gradients (EFGs) present around the cell membranes and the macromolecules. EFGs interaction with the nuclear quadrupole moment, results in creation of the two T2 components.
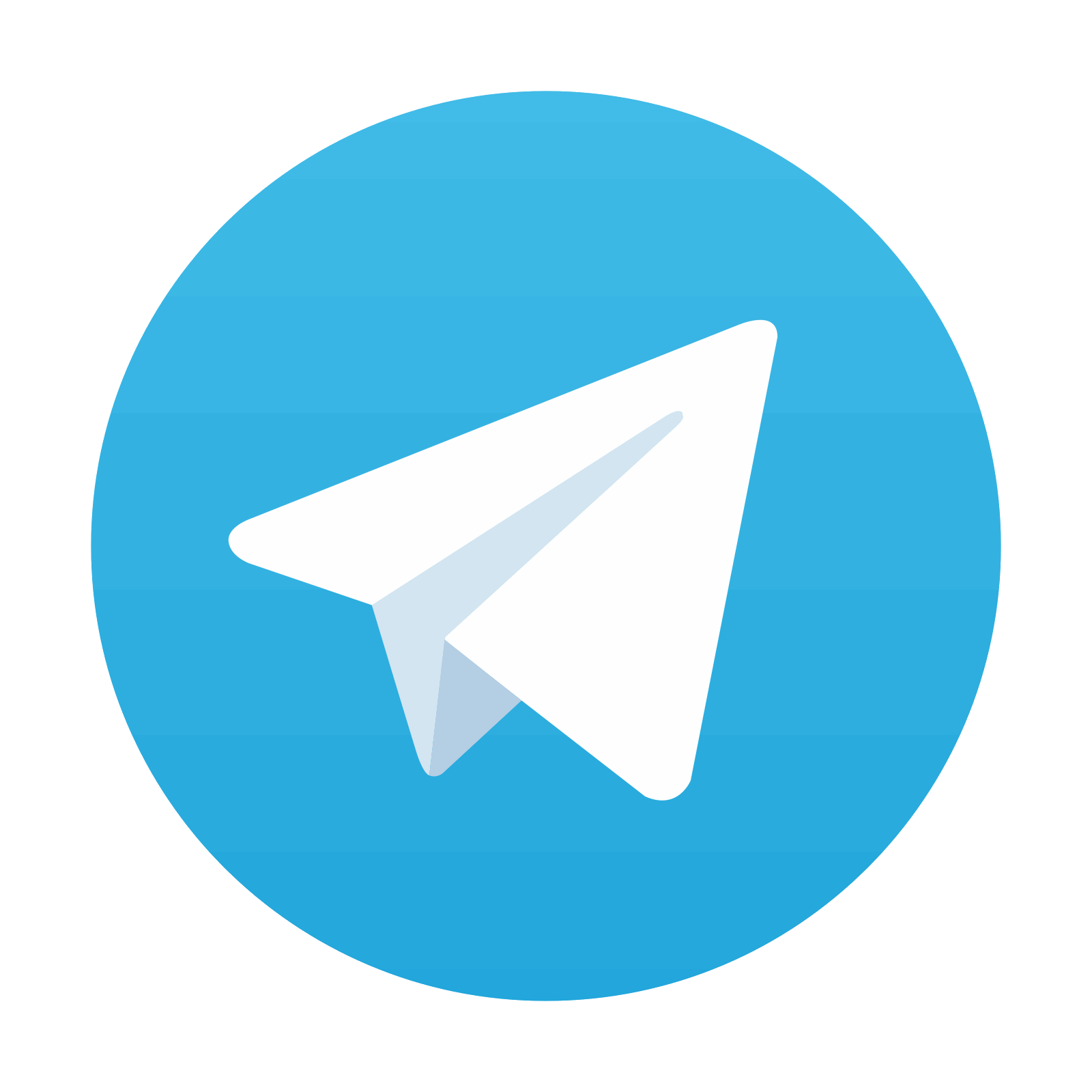
Stay updated, free articles. Join our Telegram channel

Full access? Get Clinical Tree
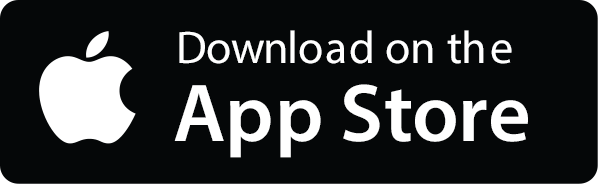
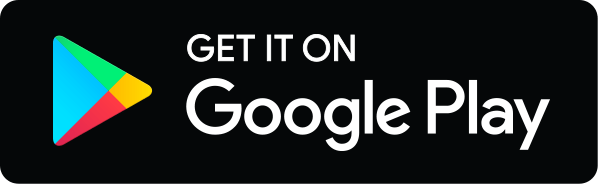