Fig. 10.1
Chemical structures of tau PET radiotracers
10.3.1 Naphthylethylidene Derivative
[18F]FDDNP was developed as a nonselective PET tracer for SPs and NFTs in the brain. Autoradiography of AD brain sections has revealed binding of [18F]FDDNP in the temporal and parietal cortices, which matched the immunohistochemistry of Aβ and tau [10]. Clinical PET studies with [18F]FDDNP have revealed regional accumulation of [18F]FDDNP in the SP- and NFT-rich areas of the AD brain [11]. FDDNP retention in the medial temporal lobes of subjects with mild cognitive impairment (MCI) was at levels that are intermediate relative to FDDNP retention levels in patients with AD and normal subjects [12] and contrasts with the bimodal distribution of Aβ-selective PET tracers in patients with MCI [13]. These findings suggest that FDDNP may be useful in tracing the progression of tau pathology in the MCI stage of AD patients. [18F]FDDNP PET has also been applied to the assessment of patients with Down syndrome [14], suspected of chronic traumatic encephalopathy (CTE) cases [15], and patients with progressive supranuclear palsy (PSP) [16]. However, the lack of selectivity of FDDNP to PHF-tau will make it difficult to interpret the distribution of tau pathology, because the possibility of concomitant tracer binding to Aβ plaques cannot be ruled out.
10.3.2 Phenyl/Pyridinyl-Butadienyl-Benzothiazoles/Benzothiazolium Derivative
A series of phenyl/pyridinyl-butadienyl-benzothiazoles/benzothiazoliums (PBBs) have been developed as candidates for tau PET tracers [17, 18]. These fluorescent compounds clearly stained NFTs, neuropil threads, and dystrophic neurites in AD brain sections. Intriguingly, these compounds additionally stained various tau inclusions in non-AD tauopathies, such as Pick’s disease, PSP, and corticobasal degeneration (CBD). Three hydrophilic compounds (PBB3, PBB4, and PBB5) showed relatively lower binding affinity to Aβ deposits than did the other lipophilic derivatives. Among them, PBB3 exhibited reasonable biostability and sufficient BBB permeability in mice. A study of [11C]PBB3 PET in PS19 mice has shown successful noninvasive visualization of tau protein deposits in the brain. From these findings, [11C]PBB3 was selected as a final tau PET tracer candidate. An exploratory clinical study has been reported in three healthy controls and three patients with AD [17]. The [11C]PBB3 and amyloid PET tracer [11C]PiB exhibited different patterns of brain retention. Of particular note is that [11C]PBB3 showed preferential accumulation in the medial temporal cortex of patients with AD, where the high density of PHF-tau has been observed in postmortem studies. Furthermore, high [11C]PBB3 accumulation has been observed in the neocortex and subcortical structures of a patient with corticobasal syndrome, suggesting the potential utility of this tracer for in vivo detection of tau lesions in non-AD tauopathies. [11C]PBB3 exhibited minimal nonspecific binding to white matter and brainstem. However, a high density of tracer signals was observed in dural venous sinuses. We expect ongoing multicenter PET studies of [11C]PBB3 and the newly developed 18F-labeled derivative to validate the clinical usefulness of PBB3 derivatives for noninvasive assessment of tau pathology in the human brain.
10.3.3 Benzimidazole Pyrimidine Derivatives
A series of derivatives of benzimidazole pyrimidines have been developed as potential candidates for use as tau tracers. Two promising derivatives, [18F]AV-1451 ([18F]T807) and [18F]T808, have been reported as highly selective tau tracers [19, 20]. Human AD brain sections were used for the assessment of binding selectivity to PHF-tau. An in vitro autoradiography study in AD brain sections has shown the high binding affinity of [18F]AV-1451 to PHF-tau (K d = 14.6 nM). Furthermore, [18F]AV-1451 binding on AD brain slices co-localizes completely with immunoreactive tau pathology. As mentioned above, the high binding selectivity to tau over Aβ is one of the requirements for a useful tau PET tracer. From the analysis of human brain sections, [18F]AV-1451 showed an estimate 29-fold in vitro binding selectivity to tau over Aβ. A PET study using [18F]AV-1451 conducted in patients with AD and MCI and healthy participants [19]. There was rapid and sufficient tracer distribution in the brain and low white matter retention after intravenous administration of this tracer. Cortical [18F]AV-1451 retention followed the known distribution of PHF-tau in AD brain. Signal-to-background ratio of [18F]AV-1451 was far higher than those of abovementioned tracers. Furthermore, [18F]AV-1451 retention was strongly associated with disease severity. Recent PET studies have suggested the potential usefulness of this tracer for imaging tau lesions in chronic traumatic encephalopathy [21, 22] and non-AD tauopathies.
First-in-man [18F]T808 PET study showed better tracer kinetics than [18F]AV-1451 [23]. However, further clinical evaluation of [18F]T808 was terminated due to substantial defluorination of this tracer in some cases.
10.3.4 Arylquinoline Derivatives
Quinoline and benzimidazole derivatives were discovered to be potential candidates for tau tracer by the screening of a chemical compound library [24]. The quinoline derivative BF-170 showed high binding affinity to synthetic tau protein fibrils and selectively bound to neurofibrillary tangles, neuropil threads, and dystrophic neurites in AD brain sections. Subsequently, the binding selectivity of 18F-labeled BF-170 ([18F]THK-523) was confirmed by the autoradiography of AD brain sections [25, 26]. Following compound optimization, the three 18F-labeled quinoline derivatives ([18F]THK-5105, [18F]THK-5117, and [18F]THK-5351) have been developed and tested in humans [27–29]. Autoradiographic analyses of AD brain sections showed the preferential binding of these tracers to NFTs and neuropil threads, which co-localized with Gallyas-positive and immunoreactive tau protein deposits (Fig. 10.2) [26, 27]. The binding selectivity of THK-5117 to tau over Aβ was estimated to be 30-fold greater. The amount of tracer binding was correlated with the amount of PHF-tau in AD brain homogenates, but it was not correlated with that of Aβ. The biodistribution of 18F-labeled THK compounds was examined in ICR mice. The initial uptake of these tracers in mice (>4% ID/g at 2 min postinjection) meets the prerequisites for useful PET imaging agents described above.
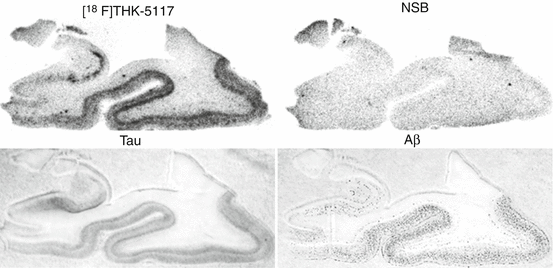
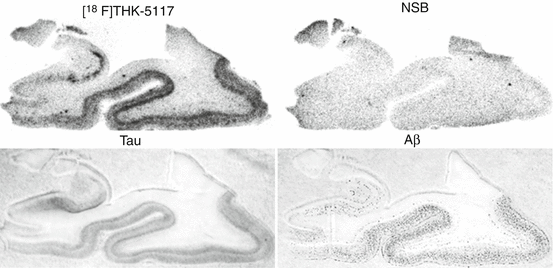
Fig. 10.2
In vitro autoradiography of [18F]THK-5117 in a temporal section from an AD patient, and immunostaining with anti-tau and anti-Aβ antibodies
First-in-human PET studies with [18F]THK-5105 and [18F]THK-5117 showed a clear distinction between patients with AD and healthy elderly controls. Partial volume corrected [18F]THK-5117 PET images of a healthy control subject (73-year-old man, MMSE score 30) and a patient with AD (79-year-old, MMSE score 16) are shown in Fig. 10.3. Retention of [18F]THK-5117 was observed in the medial temporal cortex of patients with AD, while it was not remarkable in the healthy control subject. [18F]THK-5117 was preferentially accumulated in the temporal and parietal lobe of the AD case, despite diffuse neocortical [11C]PiB retention (Fig. 10.4). In addition, the amount and extent of [18F]THK-5117 retention was associated with the clinical severity of dementia. These results were consistent with findings from postmortem studies showing significant correlations of NFT density with clinical severity of dementia. A new tracer [18F]THK-5351 showed a higher signal-to-noise ratio and lower white matter retention than [18F]THK-5105 and [18F]THK-5117, which will allow clear visual assessment and sensitive detection of tau pathology.
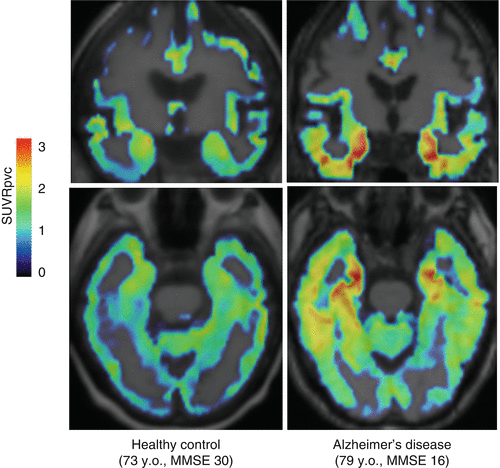
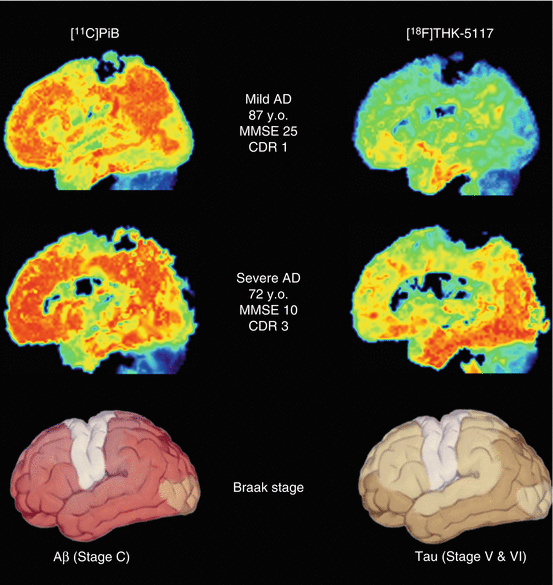
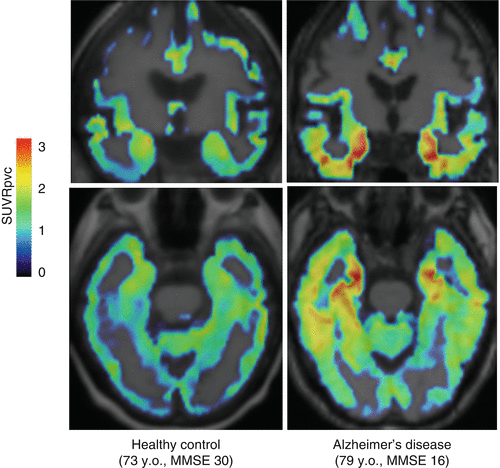
Fig. 10.3
[18F]THK-5117 PET images (after partial volume correction) from 60 to 80 min postinjection in an HC (73 years old, MMSE 30) and in AD patient (79 years old, MMSE 16)
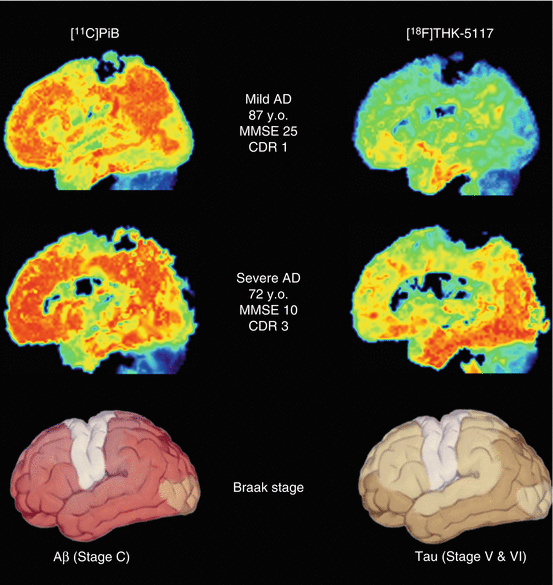
Fig. 10.4
Maximum intensity projection of [11C]PiB and [18F]THK-5117 PET images (after partial volume correction) in mild AD (87 years old, MMSE 25, CDR 1) and severe AD (72 years old, MMSE 10, CDR 3)
10.4 The Role of Tau Imaging
Although AD is the most common tauopathy, many other neurodegenerative diseases are also known to be associated with the pathological aggregation of tau protein in the brain. These neurodegenerative diseases include frontotemporal dementia, PSP, CBD, CTE, argyrophilic grain, disease (AGD) [30], and senile dementia of neurofibrillary tangle type (SDNFT) [31]. Tau lesions in CTE are associated with repeated head trauma and are frequently observed in military veterans and retired football players [32]. They are considered as high-risk populations for future progression to dementia and depression. Therefore, these populations might also be eligible to participate in preventive intervention using anti-tau drugs. The clinical diagnosis of AGD and SDNFT is generally difficult, because of the similarity of the clinical phenotype of these diseases with that of AD. Using a combination of both amyloid and tau PET imaging, these diseases could be accurately diagnosed in live patients. Recently, individuals with imaging/biomarker evidence of AD-like neurodegeneration—but without β-amyloidosis—have been called “suspected non-Alzheimer’s pathophysiology (SNAP)” [33]. Previous studies have shown that more than 20% of cognitively normal individuals and MCI subjects were categorized as SNAP. Although hippocampal atrophy correlates with tau pathology, other pathologies (including ischemia and hippocampal sclerosis) are also known to produce hippocampal atrophy. Tau PET will clarify the contribution of tau pathology to the neurodegenerative process observed in subjects with SNAP.
The diagnosis of AD is complicated by the fact that there is a discrepancy between the clinical phenotype and neuropathological findings. Recent amyloid PET studies have shown that a large amount of SPs is already present in the brain, even in an asymptomatic stage of AD. The pathological Aβ deposition in the brain starts 15–20 years before clinical dementia symptoms appear. A high amyloid burden in elderly people is considered a strong risk factor for future progression to dementia. However, it is hard to predict when these individuals will exhibit dementia using only amyloid PET findings. For the prediction of progression to AD, it is necessary to know the progress of downstream events including tau deposition, inflammation, synaptic failure, and neurodegeneration. The assessment of neurofibrillary pathology using tau PET will support an accurate prediction of prognosis in people who are at high risk of dementia. Furthermore, this technology could be used for the assessment of disease severity in the early stages of AD.
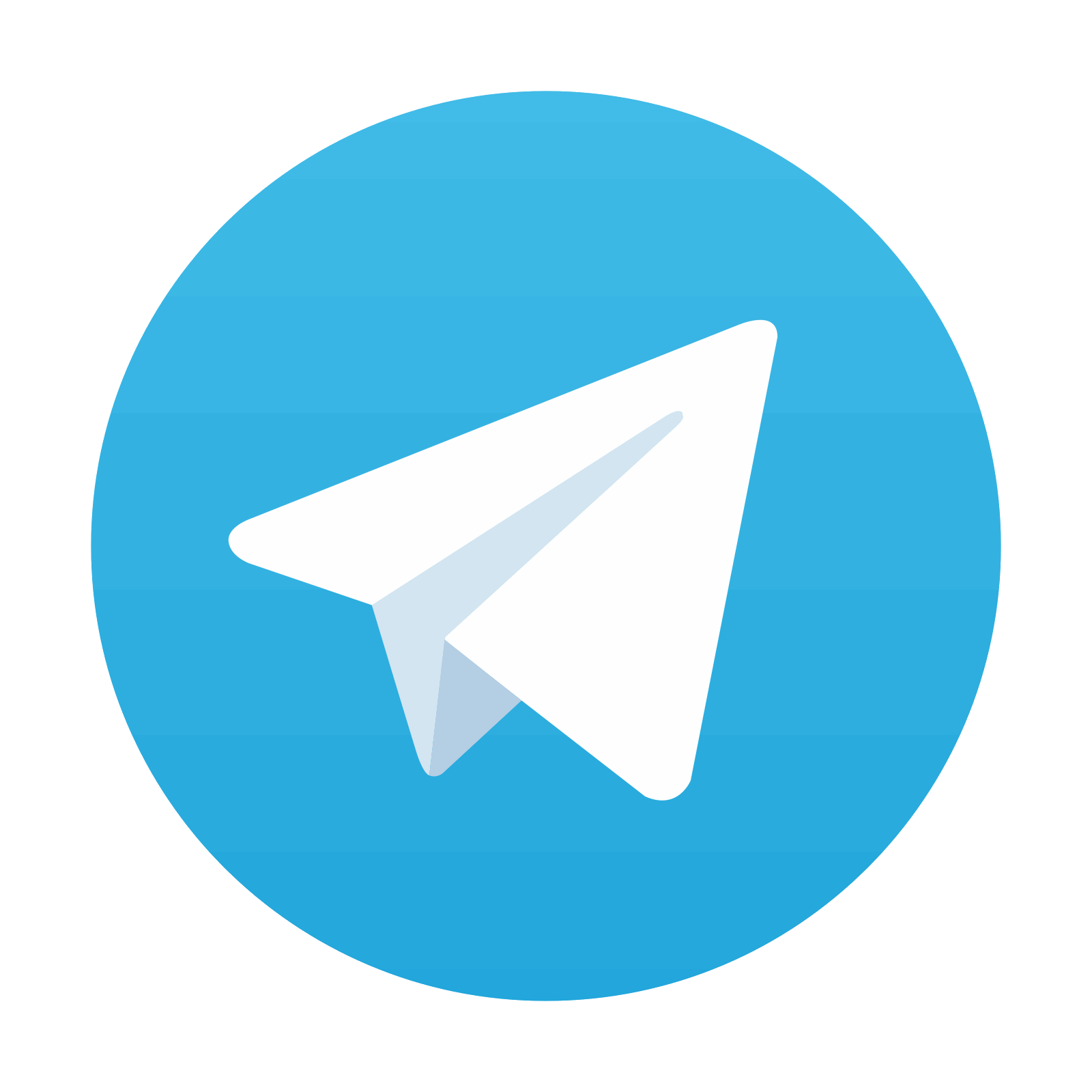
Stay updated, free articles. Join our Telegram channel

Full access? Get Clinical Tree
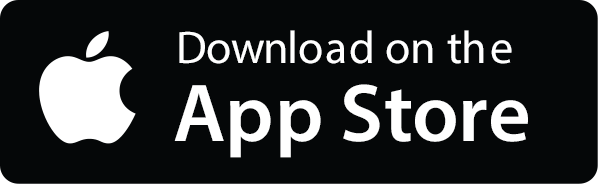
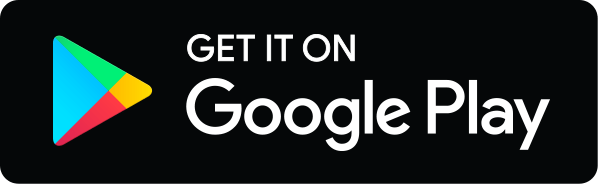