© Springer Science+Business Media LLC 2017
Laura M. Cole (ed.)Imaging Mass Spectrometry Methods in Molecular Biology161810.1007/978-1-4939-7051-3_1616. The Future in Disease Models for Mass Spectrometry Imaging, Ethical Issues, and the Way Forward
(1)
Centre for Mass Spectrometry Imaging, Biomolecular Sciences Research Centre, Sheffield Hallam University, City Campus, Howard St., Sheffield, S1 1WB, UK
Abstract
Mass Spectrometry Imaging (MSI) has evolved into a valuable tool for research into and the diagnosis of disease pathology. The ability to perform multiplex analysis of a wide range of molecules (e.g., proteins, lipids, and metabolites) simultaneously per tissue section while retaining the histological structure of the sample allows molecular information and tissue morphology to be correlated, thus increasing our understanding of a particular disease. Further development of MSI is required to improve suitability to the alternative models available, so that the combined approach can successfully provide the information required in disease characterization and prevention. MSI has been shown to be capable of providing spatiomolecular information in tumor spheroids, living skin equivalents, and ex vivo human tissues. Due to a considerable interest and scientific effort there are many more designed alternative disease models available which would benefit from the information MSI could provide.
Key words
Multiplex analysisDisease modelsTumor spheroidsLiving skin equivalentsSpatiomolecular1 Mass Spectrometry Imaging of Disease
Biological tissue of both human and animal origin is vastly complex; the spatial and temporal variation in the molecular processes of disease requires advances in experimental techniques and instrumentation in order to further elucidate the pathological processes of disease. Mass Spectrometry Imaging (MSI) has evolved into a valuable tool for research into and the diagnosis of disease pathology. The ability to perform multiplex analysis of a wide range of molecules (e.g., proteins, lipids , and metabolites) simultaneously per tissue section while retaining the histological structure of the sample allows molecular information and tissue morphology to be correlated, thus increasing our understanding of a particular disease. There are several advantages to using MSI for the analysis of tissues, especially with proteomic studies. Immunohistochemistry, for example, is a robust and popular technique for the investigation of protein expression and localization within tissues. However, much like many conventional protein analysis techniques, it involves lengthy protocols for the extraction and detection of target proteins. Furthermore, although this technique can provide spatial information, it is majorly limited in the amount of data that can be acquired from each experiment and the target protein must be known in advance in order to select the appropriate antibody for staining. On the other hand, MSI can be used for the discovery of proteins related to a specific disease or treatment with no target-specific reagents needed; in addition to this, it is highly specific and hundreds if not thousands of peptides can be detected simultaneously from one experiment on one single tissue section. Following MSI, the tissue section that has been imaged can be removed from the mass spectrometer and subjected to histological staining, such as Hematoxylin and Eosin staining, to provide architectural information about the exact piece of tissue which has been imaged.
MSI is commonly used in various modalities to study the pathogenicity of disease progression or tissue response to treatments; matrix -assisted laser desorption ionization MSI (MALDI-MSI ) was used to observe the spatial distribution of peptides within a mouse fibrosarcoma model following treatment with the tubulin-binding tumor vascular disrupting agent, combretastatin A-4-phosphate (CA-4-P) [1]. Alterations in peptide distribution as a result of an increase in hemoglobin following treatment with CA-4-P were observed within the MALDI-MSI images . Additionally, MSI is also showing its potential to be applied to disease diagnosis and classification. This is particularly useful in diseases such as breast cancer where the heterogenic nature means patients often have very different survival rates due to tumor phenotypes affecting the metabolic response to therapeutic agents [2, 3]. Guenther and colleagues performed a study using Desorption Electrospray Ionization MSI (DESI -MSI) in order to diagnose and evaluate the metabolic phenotype of human breast tumor biopsies [4]. DESI -MSI was able to achieve 98.2% accuracy in breast cancer diagnosis along with a correlation between the metabolomics profile and grade of the tumors with hormone receptor status. Human breast cancer tumor type and grade has also recently been analyzed by Airflow-assisted ionization MSI (AFAI-MSI) based on the lipid profiles of invasive ductal carcinomas and in situ ductal carcinomas [5]. AFAI-MSI does not require any sample pretreatment or labeling and analysis results in the production of a multi-color map illustrating the spatial distribution and intensities of molecules within a tissue. MSI research has now entered the frontier of whole-body imaging allowing a multi-modality approach to biomedical imaging. MALDI-MSI was used to detect inflammatory protein responses in rats infected with Staphylococcus aureus [6]. The specificity of MALDI-MS was used in conjunction with high-resolution images produced by magnetic resonance imaging to produce an image obtaining molecular and structural information on the distribution of immune response proteins throughout the entire rat body following systemic infection.
2 Tissue Sample Preparation: Fresh Frozen
When tissue is harvested for use in scientific research applications, specifically MSI, it is vital that such specimens are handled correctly in order to preserve tissue viability. It is imperative that minimal time passes from the harvest event to freezing the tissue as ischemia, nutrient starvation, and temperature will all contribute to enzymatic degradation within the tissue which will lead to poor sample quality for MS analysis, particularly for proteomic and metabolomic analysis. Snap freezing tissue in isopentane cooled by liquid nitrogen [7] or floating the specimen on liquid nitrogen is advised as immersing the tissue within liquid nitrogen can induce tearing and cracking [8]. The main disadvantage of fresh frozen tissue is the limited lifespan of the samples and demand on freezer storage space, if samples are not stored in an embedding medium they will begin to dry out over time making sectioning and subsequent analysis difficult. However, this method of sample preparation for MSI is often favorable due to the reduced washing steps therefore retaining small molecules, drugs, and lipids for the detection by MSI, especially important when performing drug distribution studies.
3 Tissue Sample Preparation: Formalin-Fixed Paraffin Embedded
Formalin fixation followed by embedding in paraffin wax is a widely used tissue preservation and storage technique that allows the long-term storage of tissues at room temperature. Formalin-fixed paraffin-embedded (FFPE) tissue is first dehydrated in graded ethanol and cleared in Xylene before fixation in 10% neutral buffered formalin; this step induces the formation of protein crosslinks within the tissue [9]. The specimen is then embedded within paraffin wax and is stored permanently like this ready for sectioning.
There is a vast library of available FFPE tissue samples; this provides scientists with the ability to acquire data sets from large patient cohorts, very important when obtaining statistically relevant data from the diverse human population pool. FFPE tissue can be archived for many years in hospitals and universities, along with data from the patient the specimen originated from. These tissue archives allow scientist’s access to large cohorts of samples for investigation of specific diseases. As technology advances samples can be reanalyzed providing a rich source of new information. A study using an FFPE biopsy from the spleen of a patient who died from tuberculosis and secondary amyloidosis in 1899 was analyzed revealing the presence of peptides relating to serum amyloid [10]. Another advantage of FFPE tissue is its suitability to be used for the creation of tissue microarrays (TMAs). TMAs are formed from needle core biopsies from entire patient cohorts (often hundreds) that are placed into one paraffin block. This paraffin block will then be sectioned and subjected to MSI and pathological examination allowing the acquisition of data from an entire patient cohort simultaneously. TMAs were originally developed as a higher throughput method of performing molecular and pathological analysis of tissue specimens, such as protein expression studies using IHC [11, 12]. TMAs have since been shown to provide a valuable method for protein analysis by MSI; a study using a TMA comprised of 112 needle core biopsies from lung tumor patients showed that incorporating antigen retrieval, on-tissue enzymatic digestion, and MSI proteomic analysis combined with histochemical analysis could provide the ability for classification of lung tumor biopsies [13]. Another study using MALDI-MSI with ion-mobility separation in combination with principal component analysis (PCA) showed significant differentiation between tumor types due to the distinct peptide profiles and distribution within images of a pancreatic carcinoma TMA [14].
Although FFPE tissue is described by some as the “gold standard” for histopathological analysis of tissue [15], especially proteomic analysis; challenges are still faced with the analysis of lipids and exogenous pharmaceuticals by MSI. The processing of FFPE tissue specimens includes many washing steps and de-waxing that washes away drug compounds and strips lipids from the tissues. The analysis of lipids in biological tissues is of great importance as they are often shown to play a key role in the development and progression of various diseases [16]. There is currently very little literature on the analysis of small molecules and metabolites within FFPE tissue. However, a recent study used high-resolution MALDI-FT-ICR MSI for in-situ imaging of metabolites in FFPE human tissue. Initially, they compared spectral signals from both FF and FFPE between m/z 50 and 1000 m/z revealing a high proportion of overlapping peaks and interestingly peaks from FFPE tissue were more intense between m/z 50 and 400. Signal intensity in the 600–1000 m/z range was more intense in the FF samples , perhaps because a large proportion of peaks within this mass range are lipids . Following this, using FFPE tissue, they were able to distinguish between tumor tissue and healthy mucosa, chromophobe renal cell carcinoma and renal oncocytoma, a distinction that is difficult to make using just histology and finally a prognostic marker for oesophageal adenocarcinoma was identified [15]. This data shows promise for MSI of small molecules within FFPE tissue and as it is known that small molecules and cellular components can be trapped within the meshwork of protein cross-links as a result of formalin fixation; further method development into this application would be worthwhile [17].
4 Human vs. Animal Disease Models
The analysis of human biological tissue samples by MSI currently poses more challenges and limitations due to the level of genetic diversity of human tissues and diseases compared with that of animal models; discoveries made from studies using animal models may not accurately represent the biological processes within human systems [18]. The heterogeneity, disease aetiology, and diverse environmental factors associated with human samples mean that a wide variation in data is seen with human samples, even those affected with the same disease [19]. Additionally, biopsies are often either difficult to obtain or are not suitable for the study performed due to ethical considerations; therefore, many tissue samples derive from animal origin. It is well known that the animal model is prominently integrated into scientific research. In the study of disease, an animal model is often used as a surrogate of a human subject. These studies often involve creating disorders in the animal by chemical, genetic, or surgical means in order to mimic the disease of interest or accurate animal response to treatment. However, the animal model has received an abundance of criticism for being inappropriate for use to test human disease [20]. Various animal models of disease have been shown unable to recreate the complexity of the human genome, physiology, and environment. The effect is observed in both disease characterization and treatment studies, and is becoming particularly prominent in the drug discovery field. A group of leading pharmaceutical companies have released publications on the failure of animal model-derived results to translate to clinical studies and are calling for improvement of the current models and further self-assessment of the effectiveness of their use [21]. Several studies have shown the predictive ability of prevalent models to be limited, when taking the most commonly observed adverse effects into consideration [22, 23]. Therefore, the general consensus is that improvement is required in the way that animal research is performed, and the ability of this model to sufficiently recapitulate human clinical response should be closely monitored.
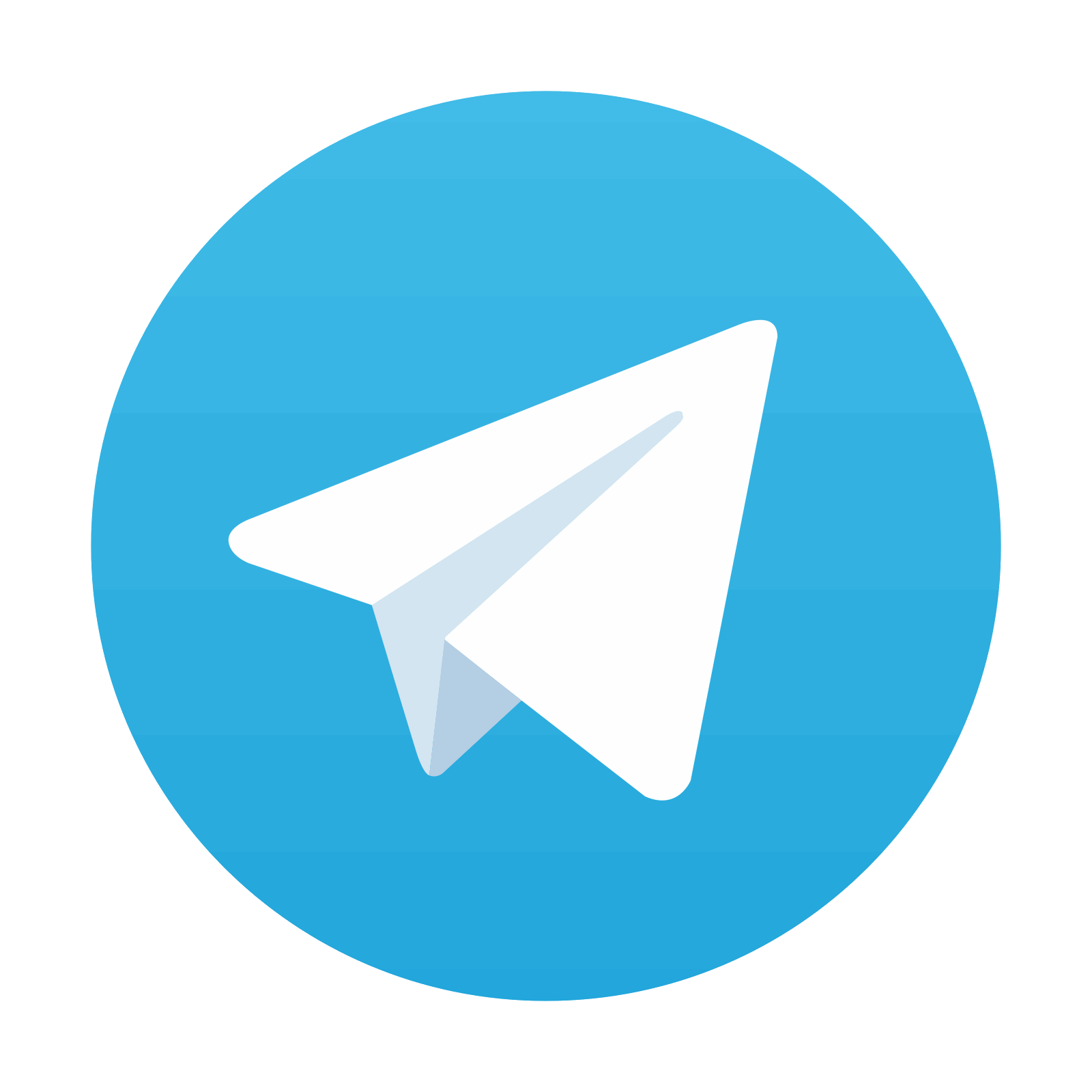
Stay updated, free articles. Join our Telegram channel

Full access? Get Clinical Tree
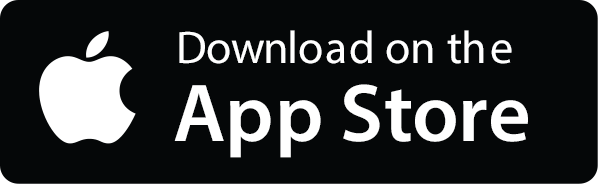
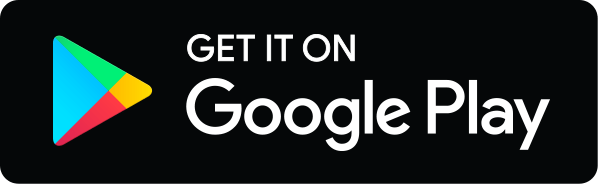