Polymorphism
Number of subjectsa
Radioligand
Results
Region
Comment
Heinz et al. (2000b)
5-HTTLPR
22 (14 AD)b
[123I]β-CIT
ll > s-Carriers (healthy subjects)
Brainstem
Opposite genotype effects in ADb
Willeit et al. (2001)
5-HTTLPR
11
[123I]β-CIT
No effect
Midbrain
Van Dyck et al. (2004)
5-HTTLPR
96
[123I]β-CIT
ss > ll > ls
Midbrain
s/s Homozygotes younger than other genotypes
Shioe et al. (2003)
5-HTTLPR
27
[11C]McN 5652
No effect
Midbrain
Parsey et al. (2006a)
Triallelic
67 (25 MDD)c
[11C]McN 5652
No effect
Serotonin-rich regions
5-HTTLPR
Praschak-Rieder et al. (2007)
Triallelic
43
[11C]DASB
La/La > s-carriers
Putamen
Results clearer when covarying for ethnicity and effects of season
5-HTTLPR
Reimold et al. (2007)
Triallelic
19
[11C]DASB
La/La > s-carriers
Midbrain
5-HTTLPR
Kalbitzer et al. (2009)
Triallelic
50
[11C]DASB
La/La > s-carriers
Caudate
5-HTTLPR
Murthy et al. (2010)
Triallelic
63
[11C]DASB
No effect
Serotonin-rich regions
Only male Caucasians, no effects of daylight
5-HTTLPR
Kauppila et al. (2013)
5-HTTLPR
30
[123I]nor-β-CIT
s-Carriers > ll
Thalamus
No effect in other regions
Laje et al. (2010)
5-HT1A
43
[11C]DASB
rs7333412 AA < AG < GG
Thalamus
rs7333412, rs7997012, rs977003
Henningsson et al. (2009)
BDNF Val66Met
25
[11C]MADAM
Val/Val > Met+
Serotonin-rich regions
Finding significant in males only
Klein et al. (2010)
BDNF Val66Met
49
[11C]DASB
No effect
Caudate, putamen, thalamus
Also no effect of plasma BDNF levels
6.2.6 The Serotonin 1A Receptor
The serotonin 1A (5-HT1A) receptor is a G-protein-coupled transmembrane protein whose main signalling effect is a reduction in the formation of cyclic adenosine monophosphate (cAMP). 5-HT1A receptors are abundantly expressed in the frontal and temporal cortex, hippocampus, septum, amygdala and the raphe nuclei, and lower levels are found in basal ganglia and the thalamus (Ito et al. 1999; de Almeida and Mengod 2008; Storvik et al. 2009). 5-HT1A receptors are considered inhibitory as, upon activation, they reduce the probability of neuronal discharge. Cortical and subcortical 5-HT1A receptors are mostly postsynaptic receptors located on glutamatergic and GABAergic neurons (Freund et al. 1990; Palchaudhuri and Flugge 2005; de Almeida and Mengod 2008), while 5-HT1A receptors in the raphe nuclei, the origin of brain serotonin innervation, are somatodendritic autoreceptors that reduce serotonergic output from the raphe into the respective target areas (Sprouse and Aghajanian 1988).
Reductions in brain 5-HT1A receptor binding have been described in anxiety disorders (Neumeister et al. 2004; Lanzenberger et al. 2007; for review, see Akimova et al. 2009), depression (Drevets et al. 1999, 2000; Meltzer et al. 2004; Hirvonen et al. 2008, but see Parsey et al. 2006b) (for review, see Drevets et al. 2007; Savitz et al. 2009) and schizophrenia (Tauscher et al. 2002; but see Frankle et al. 2006). Results on the effects of antidepressant treatment with selective serotonin reuptake inhibitors or electroconvulsive therapy on 5-HT1A binding are mixed (Moses-Kolko et al. 2007; Spindelegger et al. 2009; Saijo et al. 2010; Lanzenberger et al. 2012a). Increased 5-HT1A receptor binding has been observed in anorexia nervosa (Bailer et al. 2005, 2007) and post-traumatic stress disorder (Sullivan et al. 2013).
Several polymorphisms in 5-HT1A receptor gene (located on chromosome 5q11.2-q13; Fargin et al. 1989; Albert et al. 1990) have been studied for association with psychiatric disorders (for review, see Drago et al. 2008; Le Francois et al. 2008). The best studied polymorphism is a functional SNP in a palindromic region within the 5-HT1A promoter region (C(-1019)G; rs6295; Wu and Comings 1999) that influences gene expression by modulating the interaction of the promoter with transcription factors. The C(-1019)G C-allele has been associated with reduced 5-HT1A expression (Pernhorst et al. 2013) and was found to be associated with affective disorders in a recent meta-analysis (Kishi et al. 2012).
There is only limited evidence on the in vivo influence of the C(-1019)G polymorphism on brain 5-HT1A receptor binding. One study did not show any effect of the C(-1019)G polymorphism on brain 5-HT1A receptor binding but instead reduced 5-HT1A binding in carriers of the 5-HTTLPR short allele (David et al. 2005). Another study (Parsey et al. 2006b, c) showed increased binding of the selective 5-HT1A receptor radioligand [11C]WAY100635 in carriers of the C(-1019)G G-allele. This study also showed higher 5-HT1A receptor binding in antidepressant-naïve subjects. GG-homozygous subjects were overrepresented in the group of depressed patients in this study; however, the majority of studies find the low-expressing C-allele of C(-1019)G to be overrepresented in major depression (Kishi et al. 2012). A recent study in a relatively large collective of healthy subjects (Lanzenberger et al. 2012b) did not show any effect of C(-1019)G on brain [11C]WAY100635 binding.
The literature also reports some studies that investigated the influence of the 5-HTTLPR polymorphism on 5-HT1A receptor binding in the brain (Table 6.2). Two studies (one of them was performed in a relatively large sample of nonhuman primates) describe lower 5-HT1A receptor binding in carriers of the low-expressing 5-HTTLPR s-allele (David et al. 2005; Christian et al. 2013). Two further studies describe higher 5-HT1A receptor binding in female carriers of the 5-HTTLPR l-allele (Lee et al. 2005; Borg et al. 2009), and a third study does not detect any effect 5-HTTLPR on 5-HT1A receptor binding in a relatively large sample of healthy subjects (Borg et al. 2009). In sum, the evidence concerning the impact of genetic polymorphisms on 5-HT1A receptor binding in the brain is, as of yet, inconclusive.
6.2.7 The Serotonin 4 Receptor
The 5-HT4 receptor is abundant in serotonin-rich areas of the brain like the basal ganglia and hippocampus (Waeber et al. 1996; Varnas et al. 2003) and plays a role in anxiety- and depression-like behaviour. Studies in mice using autoradiography have shown that modification of 5-HTT gene expression was associated with alterations in 5-HT4 density: 5-HTT knockout mice showed reduced 5-HT4 density, and mice overexpressing 5-HTT showed increased 5-HT4 density (Jennings et al. 2011). 5-HT4 receptor expression and function seems to be influenced by extracellular serotonin levels, as increases in extracellular serotonin induced by paroxetine given for several weeks are associated with low 5-HT4 levels (Licht et al. 2009; Vidal et al. 2009).
The PET radioligand [11C]SB207145 is a newly developed selective tracer for imaging 5-HT4 receptors in the human brain (Marner et al. 2009, 2010; Paterson et al. 2010). Since a study in healthy volunteers suggested that acute administration of citalopram does not alter binding of [11C]SB207145 (Marner et al. 2010), it was speculated that [11C]SB207145 PET could be a useful tool for indirectly measuring chronic, but not acute, changes in extracellular serotonin levels. Fisher et al. thus conducted a study on the effects of 5-HTTLPR on binding of the selective 5-HT4 radioligand [11C]SB207145 in healthy subjects (Fisher et al. 2012). 5-HT4 receptor binding in the neocortex was 9 % lower in s-allele carriers compared to l/l homozygous subjects, providing further evidence that 5-HTTLPR genotype may influence serotonin transmission in humans. No effect of season or season-by-5-HTTLPR interaction on [11C]SB207145 binding was found in this sample. Limitations of this study are that l/l subjects were older than s-allele carriers and that datasets were collected on two different PET scanners with a significant effect of scanner type.
Table 6.2
Effects of polymorphisms on serotonin 1A (5-HT1A), serotonin 2A (5-HT2A) and serotonin 4 (5-HT4) receptor binding
Polymorphism | Number of subjectsa | Radioligand | Results | Region | Comment | |
---|---|---|---|---|---|---|
David et al. (2005) | 5HT1A | 35 | [11C]WAY 100635 (5-HT1A) | No effect | 5HT1A receptor-rich regions | Subjects also genotyped for 5-HTTLPR (see below) |
C(-1019)G | ||||||
Parsey et al. (2006b) | 5HT1A | 71 (28 MDD)b | [11C]WAY 100635 (5-HT1A) | GG > CG > CC | Raphe nuclei | |
C(-1019)G | ||||||
Lanzenberger et al. (2012b) | 5HT1A | 50 | [11C]WAY 100635 (5-HT1A) | No effect | 5HT1A receptor-rich regions | |
C(-1019)G | ||||||
David et al. (2005) | 5-HTTLPR | 35 | [11C]WAY 100635 (5-HT1A) | ll > ls/ss | Widespread | Subjects also genotyped for 5-HT1A C(-1019)G (see above) |
Lee et al. (2005) | 5-HTTLPR | 16 | [11C]WAY 100635 (5-HT1A) | ll < ls/ss | Anterior cingulum | All female study sample |
Borg et al. (2009) | 5-HTTLPR | 54 | [11C]WAY 100635 (5-HT1A) | No effect | – | 5-HTTLPR effects cognitive performance |
Lothe et al. (2009) | Triallelic | 38 | [18F]MPPF (5-HT1A) | La carriers < ss, females only | Widespread except raphe nuclei | No genotype effect in males (n = 13) |
5-HTTLPR | ||||||
Fisher et al. (2012) | Triallelic | 47 | [11C]SB207145 (5-HT4) | ll > ls/ss | Neocortex | ll Subjects older than ls/ss |
5-HTTLPR | ||||||
Henningsson et al. (2009) | BDNF Val66Met | 53 | [11C]WAY 100635 (5-HT1A) | No effect | Cortex and pons | |
Klein et al. (2010) | BDNF Val66Met | 133 | [18F]altanserin (5-HT2A) | No effect | Cortex |
6.3 Dopamine
Due to their preeminent role in addiction and psychotic disorders, brain dopamine systems have been extensively studied with PET and SPECT imaging techniques in psychiatric populations (see Cumming 2009 for in-depth review of dopamine imaging). Dopamine neurons in the brain originate almost exclusively in the brainstem substantia nigra and the more medially located ventral tegmental area. Important projection areas for dopamine neurons are the frontal cortex and subcortical structures, especially the striatum. The best studied dopaminergic region in the brain is the striatum, since it has a high density of dopamine nerve terminals and is thus a region that is particularly well suited for PET and SPECT imaging. Frequently targeted molecules are G-protein-coupled postsynaptic dopamine receptors and the dopamine transporter (DAT).
6.3.1 Dopamine D2/3 Receptors
According to their effects on postsynaptic levels of cyclic adenosine monophosphate (cAMP), dopamine receptors are grouped into two families, the D1 receptor family (dopamine D1 and D5 receptors) and the D2 receptor family (dopamine D2, D3 and D4 receptors). As the main target of antipsychotic medication, dopamine D2/3 receptors have been of particular interest for imaging studies in schizophrenia. Moreover, D2/3 receptor imaging techniques have successfully been used to demonstrate alterations in brain dopamine transmission in addictive disorders (Volkow et al. 2009; Urban and Martinez 2012). While current evidence speaks against a relevant alteration in the quantity of D2/3 receptors that are accessible to PET imaging in schizophrenia (Howes et al. 2012), several studies have shown a reduction of D2/3 binding in addictive disorders (Volkow et al. 2009; Urban and Martinez 2012).
There are several studies on the influence of genetic variation on D2/3 receptor binding in the literature (Table 6.4; for review, see Willeit and Praschak-Rieder 2010). To our knowledge, the only replicated finding as of yet is a reduction in binding of the D2/3 radioligand [11C]raclopride in carriers of the Taq1 A1 allele as compared to A2 homozygotes (Pohjalainen et al. 1998; Jonsson et al. 1999). However, a study of this polymorphism with SPECT and the radioligand [123]IBZM did not show differences between the genotypic groups (Laruelle et al. 1998). Other polymorphisms in the D2 receptor gene showed in part significant effects but still warrant replication (Table 6.3). A recent study investigated a possible association between polymorphisms in the Period2 (PER2) gene and dopamine D2/3 receptor binding as measured using [11C]raclopride and PET (Shumay et al. 2012a). PER2 is part of the clock-gene family and involved in the reaction of organisms to circadian and circannual changes in lighting conditions and day length. Dopamine transmission shows circadian and seasonal changes in rodents and humans (Castaneda et al. 2004; Tsai et al. 2011; Eisenberg et al. 2010). The authors report on a newly identified VNTR polymorphism in the human PER2 gene that was associated with cocaine craving and striatal dopamine D2/3 receptor binding. This finding is so far very exciting as it brings together several research strings on the physiology of dopamine function and its implication in addiction (see also Praschak-Rieder and Willeit 2012 for further discussion).
Table 6.3
Effects of dopamine D2 receptor polymorphisms on D2 receptor binding, dopamine transporter (DAT) binding and dopamine synthesis
Polymorphism | Number of subjectsa | Radioligand | Results | Region | Comment | |
---|---|---|---|---|---|---|
Pohjalainen et al. (1998) | Taq1 A | 54 | [11C]raclopride (2 scans) | A1 carriers < A2/A2 | Striatum | No effect on D2 affinity |
Laruelle et al. (1998) | Taq1 A, Taq1 B | 70 (23 SCZ)b | [123I]IBZM | No effect | Striatum | |
Jönsson et al. (1999) | Taq1 A | 56 | [11C]raclopride | A1 carriers < A2/A2 | Striatum | |
Taq1 B | B1 carriers < B2/B2 | |||||
–141C Ins/Del | Del carriers > Ins/Ins | |||||
Pohjalainen et al. (1999) | −141C Ins/Del | 52 | [11C]raclopride | No effect | Striatum | Same sample as in Pohjalainen et al. (1998) |
Hirvonen et al. (2004) | C957T | 45 | [11C]raclopride | T/T > C/T > CC | Striatum | Same sample as in Pohjalainen et al. (1998) |
Hirvonen et al. (2009) | C957T | 45 | [11C]raclopride (2 scans) | Effect on affinity: K D C/C > C/T > TT | Striatum | Same sample as in Pohjalainen et al. (1998) |
No effect on B max | ||||||
Bertolino et al. (2010) | D2 rs1076560 (G > T) | 37 | [123I]IBZM | D2 binding T < G | Striatum | Effect on correlation between D2 binding and prefrontal activity |
[123I]FP-CIT | DAT binding T < G | |||||
Laakso et al. (2005) | Taq1 A | 33 | [18F]FDOPA | Higher uptake in A1 carriers; no effect of –141C Ins/Del, C957T | Striatum | |
–141C Ins/Del | ||||||
C957T | ||||||
Laine et al. (2001) | Taq1 A | 29 ADc | [123I] ß-CIT | Higher DAT binding in A1 carriers | Striatum | |
Sambataro et al. (2011) | D2 rs1076560 (G > T) | 28 | [123I]FP-CIT | No effect | Striatum | |
Shumay et al. (2012a) | PER2 (Period2) | 52 | [11C]raclopride | Higher binding in 4R and 3R homozygotes | Striatum | Clock-gene family |
6.3.2 The Dopamine Transporter
Another frequently studied target is the dopamine transporter (DAT), a member of the monoamine-transporter family that transports dopamine back into the presynaptic neuron immediately after its release into the extracellular space. DAT is the main target of therapeutic drugs, for example, methylphenidate, used to treat attention-deficit hyperactivity disorder (ADHD) or the antidepressant bupropion. DAT is also the main site of action of drugs of abuse such as cocaine or amphetamines. Radiolabelled DAT blockers such as [11C]methylphenidate or [11C]cocaine have successfully been used to quantify DAT availability in the human brain.
Several polymorphisms within the DAT gene or in its promoter region have been identified and studied for association with psychiatric disorders, and there is extensive literature on imaging the effects of some of these polymorphisms on DAT binding in the human brain (Willeit and Praschak-Rieder 2010). The most frequently imaged polymorphism is a 40-bp (base pair) VNTR (variable number of tandem repeats) polymorphism in the 3′ untranslated region of exon 15 of the DAT gene SLC6A3 (Vandenbergh et al. 1992, 2000). These studies included healthy subjects as well as patients with alcohol dependence, schizophrenia, ADHD and Parkinson’s disease (Heinz et al. 2000a; Jacobsen et al. 2000; Martinez et al. 2001; Lynch et al. 2003; Cheon et al. 2005; van Dyck et al. 2005; Krause et al. 2006; Lafuente et al. 2007; van de Giessen et al. 2009; Table 6.4). A recent methodologically sound meta-analysis (Costa et al. 2011) provides no evidence to support the hypothesis that the DAT 40-bp VNTR is significantly associated with interindividual differences in DAT availability in the striatum. A SPECT study investigating a cluster of polymorphisms flanking a recently described splice variant in the DAT gene (Talkowski et al. 2010) did not show significant differences in [123I]-β-CIT binding (van de Giessen et al. 2012). In summary, the results of these studies give no clear picture on the effects of the DAT 40-bp VNTR polymorphism. However, the number of studies is relatively small, and there is significant heterogeneity between the studies.
Table 6.4
Effects of polymorphisms within the dopamine transporter gene SLC6A3 on in vivo dopamine transporter binding in humans
Polymorphism | Number of subjectsa | Radioligand | Results | Region | Comment | |
---|---|---|---|---|---|---|
Heinz et al. (2000a) | DAT 3′ VNTR | 25 (14 AD)b | [123I]-β-CIT | 9-repeat carriers <10/10 | Putamen | Alcohol not associated with DAT binding genotype |
Jacobsen et al. (2000) | DAT 3′ VNTR | 44 (14 CA)c | [123I]-β-CIT | 9-repeat carriers >10/10 | Striatum | 5-HTTLPR not associated with [123I]-β-CIT binding in midbrain |
Martinez et al. (2001) | DAT 3′ VNTR | 43 (22 SCZ)d | [123I]-β-CIT | No effect | Striatum | No genotype effect on amphetamine-induced dopamine release |
Lynch et al. (2003) | DAT 3′ VNTR | 166 (100 PD)e | [99mTc]TRODAT-1 | No effect | Striatum | Also no effect of COMT and MAO-B polymorphisms |
Van Dyck et al. (2005) | DAT 3′ VNTR | 96 | [123I]-β-CIT | 9-repeat carriers >10/10 | Striatum | |
Cheon et al. (2005) | DAT 3′ VNTR | 11 children with ADHDf | [123 I] IPT | 9-repeat carriers <10/10 | Basal ganglia | Response to methylphenidate better in 9-repeat-allele carriers |
Krause et al. (2006) | DAT 3′ VNTR | 22 ADHDf | [99mTc]TRODAT | No effect | Striatum | |
Lafuente et al. (2007) | DAT 3′ VNTR | 15 SCZd | [123I] FP-CIT | No effect | Striatum | |
van de Giessen et al. (2009) | DAT 3′ VNTR | 79 | [123I]-β-CIT | 9-repeat carriers > 10/10 | Striatum | Haplotype 5′ T-A 3′ 9R highest DAT binding |
DAT 5′ VNTR | ||||||
Drgon et al. (2006) | DAT 5′ VNTR (rs2652511, rs2937639) | 15 (6 ADHD)f | [11C]-cocaine PET | C-G > T-A | Ventral striatum | 5′ C-G combination confirmed postmortem |
Szobot et al. (2010) | DAT 3′ VNTR | 17 ADHDf | [99mTc]TRODAT | Reduced occupancy in DAT10R homozygotes/DRD4-7R carriers | Striatum | Subjects scanned before and after 3 weeks of methylphenidate treatment |
DRD4 48-bp VNTR |
6.3.3 Measuring Dopamine Transporter Function
Radiolabelled dopamine precursors able to cross the blood-brain barrier (e.g. [18F]dihydroxyphenylalanine, [18F]DOPA) are converted into the DAT substrate [18F]dopamine in dopaminergic neurons. The striatal [18F]DOPA influx constant Ki reflects presynaptic dopamine uptake, synthesis and storage capacity and is regarded as a good proxy for presynaptic dopamine metabolism in the brain (Cumming 2009). Together with so-called ‘competition’ paradigms, a research technique that allows for measuring the inhibition of radioligand binding at D2/3 receptors by endogenous dopamine, [18F]DOPA studies have now clearly shown that presynaptic dopamine synthesis capacity (Reith et al. 1994; Hietala et al. 1995, 1999; Lindstrom et al. 1999; Meyer-Lindenberg et al. 2002; McGowan et al. 2004; Kumakura et al. 2007; Bose et al. 2008; Nozaki et al. 2009), baseline synaptic dopamine levels (Abi-Dargham et al. 2000, 2009; Kegeles et al. 2010) and pharmacologically induced dopamine release (Laruelle et al. 1996; Breier et al. 1997; Abi-Dargham et al. 1998, 2009) are increased in schizophrenia (for review, see Howes et al. 2012).
Imaging measures of the hyper-dopaminergic state in schizophrenia can as well be conceptualized as an endophenotype of the disorder. Indeed, there are a number of studies on the genetic background of in vivo imaging measures of presynaptic dopamine function (Table 6.5). These studies investigated the influence of polymorphisms in the DAT gene (Martinez et al. 2001; Brody et al. 2006), in D2 and D3 receptor-related genes (Brody et al. 2006; Pecina et al. 2012; Savitz et al. 2013), in the gene coding for oxytocin (Love et al. 2012) and in the μ-opioid receptor gene (Domino et al. 2010) on DAT function. In these studies, dopamine release was induced either by administration of d-amphetamine, by nicotine from cigarettes or by behavioural stimuli such as stress and pain. All of the above-mentioned studies describe an association with reductions in D2/3 radioligand binding (as an indirect measure for dopamine release) and the respective candidate genes. Although there emerges no consistent picture due to the variety of polymorphisms and the different methods used, all of these studies were able to show that results of ‘competition studies’ are in part driven by genetic variation. Some of the information collected in these studies might thus be used for refining future studies in patients with schizophrenia. Perhaps even more important, these studies were able to show that several neurochemical pathways are involved in regulating dopamine release in the living human brain. Regulatory mechanisms include feedback regulation in dopamine terminal fields (see, e.g. Savitz et al. 2013, brain opioid systems (Domino et al. 2010) and the neuropeptide oxytocin (Love et al. 2012)).
Table 6.5
Effects of genetic polymorphisms on dopamine-induced reductions in D2/3 receptor binding
Polymorphism | Number of subjectsa | Outcome measure | Results | Region | Comment | |
---|---|---|---|---|---|---|
Martinez et al. (2001) | DAT 3′ VNTR | 59 (28 SCZ)b | d-Amphetamine-induced decrease in [123I]IBZM binding | No effect | Striatum | Greater reductions in patients; trend for greater reductions in 9-repeat carriers |
Brody et al. (2006) | DAT 3′ VNTR, D2 Taq A1/A, D4 VNTR, COMT Val158Met | 45 | Nicotine-induced decrease in [11C]raclopride binding | DAT: 9R > 10/10R | Striatum | |
D2: no effect | ||||||
D4: greater in <7R | ||||||
Val/Val > Met | ||||||
Pecina et al. (2012) | D2 rs4274224 (A > G) | 52 | Stress-induced decrease in [11C] raclopride binding | AG > AA/GG | Caudate, putamen, ventral striatum | |
Savitz et al. (2013) | D3 Ser9Gly | 36 (10 MDD)c | Reward-induced decrease in [11C] raclopride binding | Gly > Ser | Caudate, ventral striatum | No genotype effect on baseline D2/3 binding |
Love et al. (2012) | Oxytocin | 55 | Pain-stress-induced decrease in [11C] raclopride binding | GC/CC > GG | Ventromedial caudate | Significant effects in females (n = 32) but not males |
Rs4813625 | ||||||
Domino et al. (2012) | μ-Opioid 1 receptor A118G | 19 males | Nicotine-induced decrease in [11C]carfentanyl binding | AG/GG > AA | Caudate, ventral putamen | Significant effect of the polymorphism also on plasma cortisol levels |
6.4 Other Polymorphisms
6.4.1 The Catechol-O-methyltransferase Val158Met Polymorphism
Catechol-O-methyltransferase (COMT) is an enzyme that catalyzes degradation of catecholamines (including epinephrine, norepinephrine and dopamine) in the periphery and the brain. While regulation of extracellular dopamine levels is heavily dependent on DAT function in subcortical brain areas, COMT is believed to be a key mechanism regulating dopamine activity in cortical, especially frontal cortical brain areas (Ciliax et al. 1999; Lewis et al. 2001; Matsumoto et al. 2003).
Several SNPs have been described in the COMT gene, but without doubt, the SNP causing a valine-to-methionine substitution at position 158 (COMT Val158Met) is one of the most studied polymorphisms in psychiatry and a series of other disorders. The COMT 158Val variant degrades dopamine more efficiently than the COMT 158Met variant (Lachman et al. 1996), and the polymorphism has been associated with several psychiatric and other disorders. A finding that seems to hold true is a difference in executive function mediated mainly by prefrontal cortex dopamine between carriers of the gene variants. Thus, several studies have investigated behavioural, cognitive, functional and anatomical variance associated with this gene as an endophenotype in various disorders (see, e.g. Schosser et al. 2011; Witte and Floel 2011; Ira et al. 2013).
Two studies investigated the effects of COMT Val158Met using [18F]DOPA and PET. A study (Meyer-Lindenberg et al. 2005) in healthy volunteers found increased striatal [18F]DOPA uptake in 158Val carriers when compared to 158Met homozygotes. Another study in patients with early Parkinson’s disease (Wu et al. 2012) describes higher [18F]DOPA uptake measured between 150 and 210 min after radioligand injection in 158Met homozygous patients. Besides differences between study samples (young healthy volunteers versus patients with Parkinson’s disease), the difference might be due to the fact that higher [18F]DOPA in 158VMet homozygous patients with Parkinson’s disease was measured from 150 min after tracer injection onwards. At this time, the [18F]DOPA signal is believed to reflect rates of dopamine metabolism to 3,4-dihydroxyphenylacetic acid and homovanillic acid (Ruottinen et al. 2001; Ceravolo et al. 2002).
A study in healthy volunteers investigated the effects of COMT Val158Met on dopamine D2/3 receptor binding using PET and the radioligands [11C]raclopride and [11C]FLB457 for assessing striatal and cortical D2/3 binding, respectively. Since genotype had no effect on binding of either radioligand, the authors concluded that baseline in vivo D2/3 receptor availability does not differ between COMT Val158Met genotypic groups. In contrast, another study using the dopamine D1 receptor radioligand [11C]NNC112 (Slifstein et al. 2008) found significantly higher cortical D1 receptor binding in Val158 homozygotes, while no difference was found in striatum. This finding is in good agreement with the functional role of COMT Val158Met, as low dopamine levels have been found to cause upregulation of dopamine D1 receptors in the rodent brain, while dopamine transmission in the basal ganglia is less affected due to the prominent role of DAT in these brain areas (Gogos et al. 1998; Guo et al. 2003). A recent study investigating the effects of the COMT Val158Met polymorphism on brain 5-HT1A receptor binding found significantly higher [11C]WAY100635 binding in Val158 homozygotes in orbitofrontal cortex, cingulum, insula and amygdale (Baldinger et al. 2013). Peak differences were found in the posterior cingulate cortex.
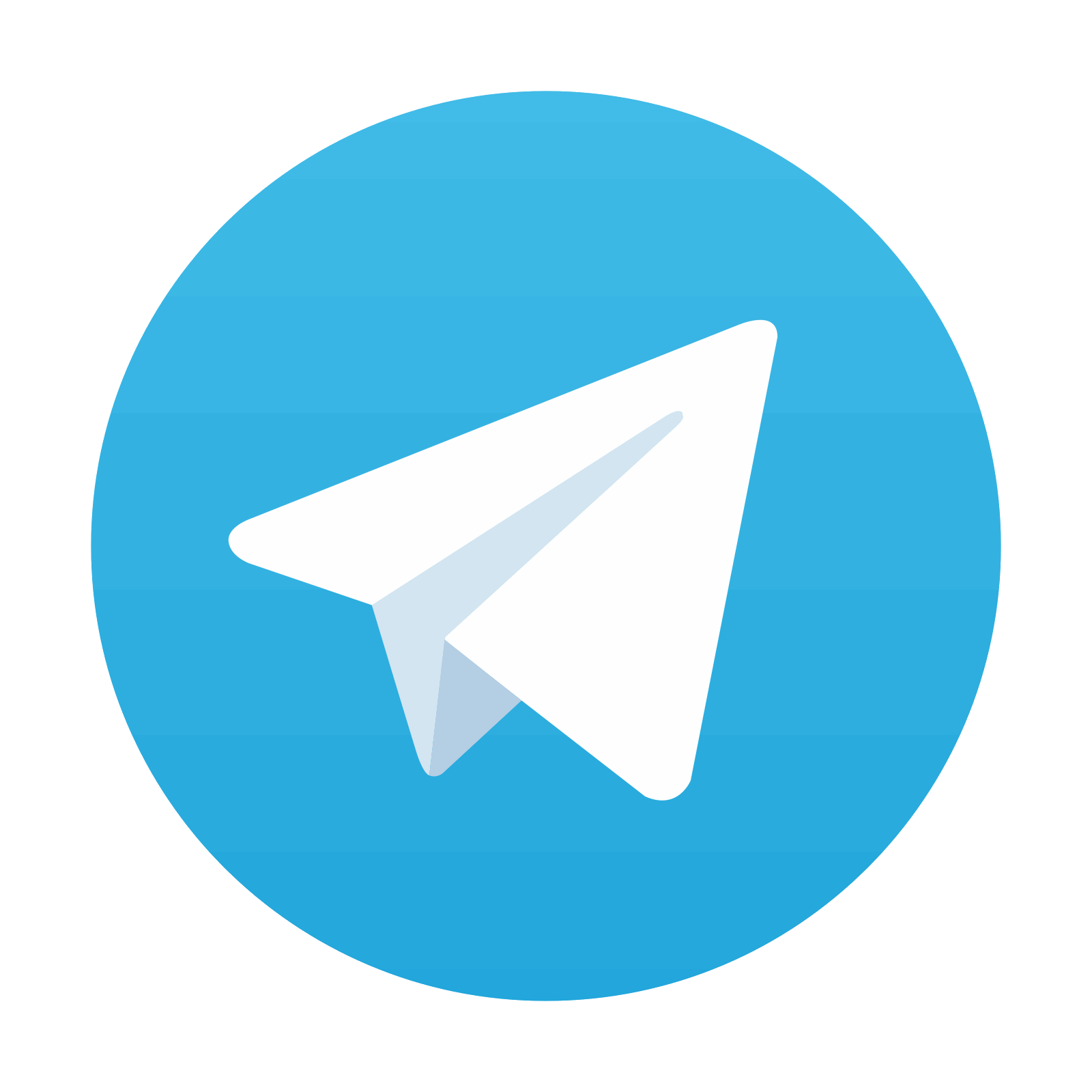
Stay updated, free articles. Join our Telegram channel

Full access? Get Clinical Tree
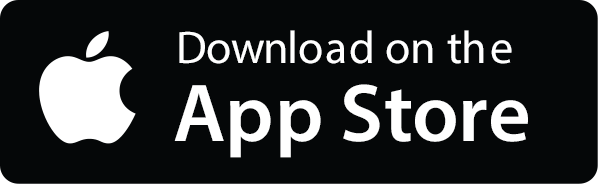
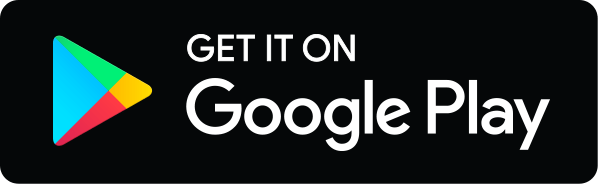