Ultrasound
MR imaging
Strain—early acute phase (<6 h)
+
+++
Strain—late acute phase (>24 h)
++
+++
Muscle scar detection
++
+
Recovery time prediction
+
++
Delayed onset muscle soreness
+
++
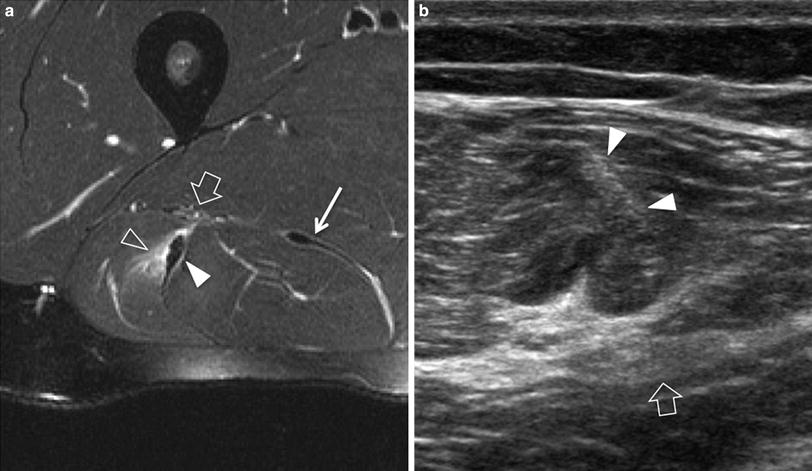
Fig. 1
Acute Grade-1 muscle injury. a Axial fat-suppressed T2-weighted MR image of the proximal thigh shows disruption of muscle fibers (void arrowhead) as they arise from the conjoined intermuscular tendon (white arrowhead) of the biceps femoris and semitendinosus. Note the sciatic nerve (large arrow) and the aponeurosis of the semimembranosus (narrow arrow). b Corresponding transverse 12-5 MHz US image reveals the conjoined aponeurosis as a sagittal hyperechoic band (white arrowheads) located superficial to the sciatic nerve (arrow). The injury in the surrounding muscle fibers is not visualized due to the relatively poor contrast of US
In the sporting setting, selection of the most appropriate imaging modality may be greatly influenced by accuracy concerns, workflow and costs (please see also “MRI of Muscle Injuries”). If US is performed sideline at the sporting event within the first few hours after the injury using portable machines, the risk of missing muscle tears should be taken into account because fresh hemorrhage (within 1-day after trauma) looks echogenic and may be quite similar to normal muscle. This problem may be compounded by two factors: (i) the image quality of portable US equipments may be suboptimal at the sideline of the sporting event to disclose minimal echotextural changes or does not have enough penetration to recognize deep-seated injuries; (ii) the examiner in charge of the event and the team is more likely to have learnt US as an adjunct to clinical work and to be less skilled to recognize the injury in a difficult operating setting (Allen and Wilson 2007). In the few hours after trauma, signs of a significant injury may be, in fact, subtle placing great demand on machine quality and operator experience. On the other hand, MR imaging is more reliable and easy to perform, but there may be problems of access and the cost may not be sustained by minor sports clubs systematically. Given some reservations, a wised use of US with selection of an appropriate timing of examination later than 1 day from trauma, when the hematoma starts to liquefy and collect becoming increasingly hypoechoic and conspicuous, might reduce the number of MR imaging examinations (Allen and Wilson 2007). This seems particularly true in the instance of mild trauma and outside the context of the highest professional levels.
When trauma occur in a professional sporting setting, the coaching staff of the club typically faces pressure to ensure a quick return of the athlete to play in order to avoid too many lost games, but a premature return to competition in an incompletely assessed and rehabilitated athlete has a high risk of reinjury and may result in a longer time removed from activity (Koulouris et al. 2007). Under these circumstances, US and MR imaging are extensively used, sometimes redundantly and in combination, to better prognosticate lay-off, healing time, mid-term outcome, as well as to guide rehabilitation and assess risk of late complication such as posttraumatic fibrosis, herniation and ossification (Connell et al. 2004; Gibbs et al. 2004; Schneider-Kolsky et al. 2006; Koulouris et al. 2007; Slavotinek 2010). Outside the high-performance context, muscle injuries often occur in the general population engaged in amateur athletic and non-athletic trivial activities. In these cases, imaging is basically directed to focus on more essential information, such as confirming the injury, defining its location and severity without the need to speculate on the exact time of return to play (Peetrons 2002). The need for serial follow-up examinations is also less. Given these considerations, one can imagine that the choice of the most appropriate imaging modality to perform is not only based on accuracy concerns but it may change depending on non-clinical factors such as level of the team, league requirements and budget (Allen and Wilson 2007). In the hamstrings, the percentage cross-sectional area (or volume) of injured muscle has been found to be a promising parameter to predict outcome, somewhat reflecting the proportion of myofibrils that have been disrupted at the level of measurement. An association between longer recovery time (>6 weeks) and involved muscle cross-sectional area >55 % has also been found (Slavotinek et al. 2002; Slavotinek 2010; Ekstrand et al. 2012). However, the strongest correlation with prognosis is given by the craniocaudal extension of muscle abnormality, as an estimate of the number of muscle units separated from the aponeurosis at the time of injury (Connell et al. 2004). Despite noticeably improvement in US technology with introduction and refinement of 3D systems and wide-FOV algorithms, size parameters are measured more simply and reproducibly on MR imaging rather than on US. In addition, the extent of the injury appears consistently smaller on US than on MR imaging, both in longitudinal and cross-sectional views, due to a lower sensitivity of US to depict edematous changes (Connell et al. 2004). Similarly, abnormalities tend to resolve sooner on US than on MR imaging during the healing process, thus making US less accurate in predicting the exact time to return to full competition.
US seems to have some advantages over MR imaging to depict posttraumatic scars (Fig. 2). They typically appear as a linear or stellate hyperechoic areas attached to the affected aponeurosis or the epimysium and cause distortion of the surrounding pennate pattern (Koh and McNally 2007). Local abnormal contraction of the muscle fibers can be revealed around the scar. From the biomechanical point of view, the local amount and severity of architectural distortion in a scarred muscle can be nicely estimated with US due to its dynamic capability. Scar conspicuity is also greater with US (Allen and Wilson 2007). On MR imaging, fresh scar may be possibly confused with an acute tear/retear due to a high signal on fluid-sensitive sequences, whereas old scars may be missed or underestimated in their extension as they are associated with low signal intensity in all sequences. Differently, a fresh retear on an old scar is more conspicuous on MR imaging due to its higher sensitivity in edema detection.
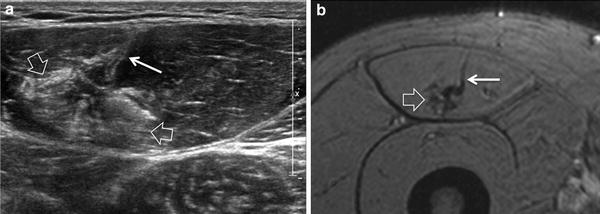
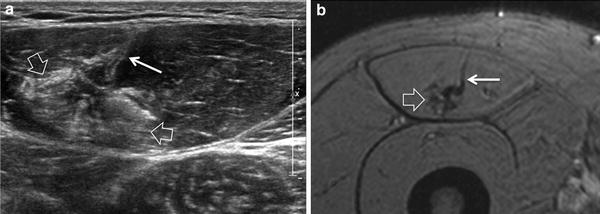
Fig. 2
Posttraumatic fibrous scar. a Transverse 12-5 MHz US image of the rectus femoris muscle with b correlative GRE T1-weighted MR imaging reveals an irregular hyperechoic image (large arrows) around the deep end of the central aponeurosis (narrow arrow) related to intramuscular fibrous scar. This finding appears more conspicuous on US
4 Delayed Onset Muscle Soreness
Delayed onset muscle soreness is a peculiar form of self-limiting injury related to muscle strain that typically does not cause serious clinical consequences (Cleak and Eston 1992). This condition is secondary to repeated eccentric contractions and its severity is proportional to the duration and intensity of the exercise (Shellock et al. 1991). One-two days following exertion, local pain, soreness, and soft-tissue swelling appear with a peak at 2–3 days and reduce until gone over the course of one week. In the early phase, the appearance of the muscle is similar to Grade-1 strain injury with a feathery-like pattern seen on fluid-sensitive sequences related to interfascicular edema (Blankenbaker and Tuite 2010). Some perifascial fluid can also be found. Compared with Grade-1 strains, delayed onset muscle soreness affects more extensive patches of the muscle, irrespective of the location of aponeuroses (Fig. 3). In cases with severe pain, imaging studies may be required to rule out a strain. Due to its ability to reveal intramuscular edematous changes, MR imaging is intrinsically more sensitive than US to detect this condition (Dierking et al. 2000) (please see also “MRI of Muscle Injuries”). In the late phase, edematous changes may persist far longer than the resolution of symptoms (Shellock et al. 1991).
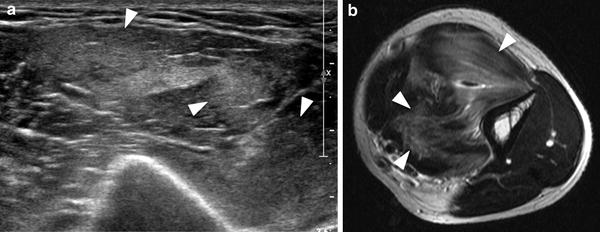
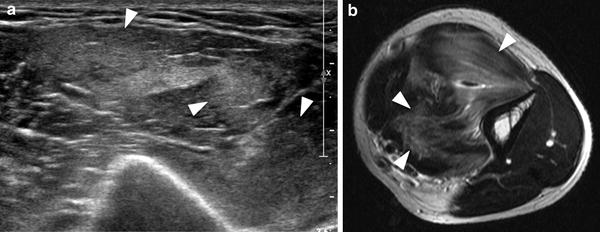
Fig. 3
Delayed onset muscle soreness. a Transverse 12-5 MHz US image of the brachialis muscle with b correlative Turbo-Spin-Echo T2-weighted MR imaging correlation demonstrates patches of muscle tissue (arrowheads) characterized by loss of fibrillar pattern and hyperechoic appearance. Diffuse feathery edema is shown on MR imaging
5 Myositis Ossificans
In early stages, myositis ossificans is a challenging diagnosis on both imaging and histopathology, because it exhibits a non-specific appearance at its onset and may often mimic an infiltrative malignant soft-tissue mass (please see also “MRI in Muscle Tumors and Tumors of Fasciae and Tendon Sheaths”). In approximately 40 % of cases, myositis ossificans cannot be linked to a specific injury and this may create difficulties in the differential diagnosis. Imaging is able to reveal the phasic changes of the process. In the initial precalcified stage, the mass grows rapidly. Initial mineralization starts around the 2nd/3rd week. During this phase, US have been found to be superior to MR imaging and radiography to detect the initial formation of calcifications (Kramer et al. 1979; Allen and Wilson 2007) (Fig. 4). Ossification represents the main clue for the diagnosis and typically consists of highly echogenic foci with posterior acoustic shadowing located at the periphery of the lesion. The peripheral pattern of mineralization is pathognomonic for myositis ossificans given that calcification is mostly central in sarcomas as a result of necrosis. Because ossification looks hypointense in all pulse sequences, it is more difficult to detect on MR imaging at early stages. Radiography may also miss initial calcific deposits due to their blurred undefined appearance or inadequate view. Later on, myositis ossificans assumes a heterogeneous appearance with more consistent calcified shell. The mass may exhibit a hypervascular pattern both at the periphery and in the center. Central hyperemia may help distinguish this lesion from an intramuscular abscess (Koh and McNally 2007). As the maturation progresses, the lesion gradually takes the form of a bulky calcification, which is often longitudinally oriented and parallel to adjacent bone.
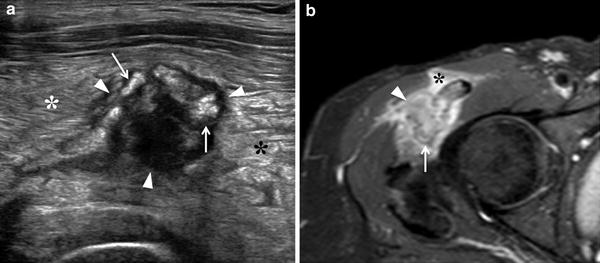
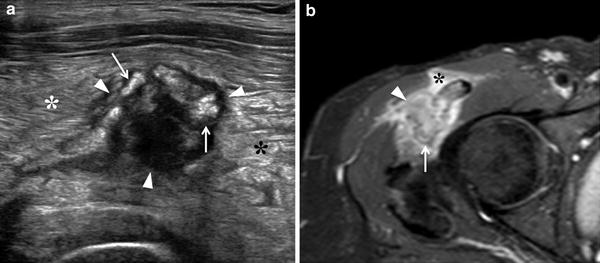
Fig. 4
Myositis ossificans, early stage. a Transverse 12-5 MHz US image over the anterolateral right hip with b correlative fat-suppressed Turbo-Spin-Echo T2-weighted MR image demonstrates a heterogeneous soft-tissue mass (arrowheads) with peripheral calcified deposits (arrows) surrounded by intense inflammatory changes (asterisks). The calcific component of the mass is better visualized with US
6 Muscle Hernia
US is an accurate means to identify muscle hernias and assess their size during dynamic scanning (Bianchi et al. 1995; Beggs 2003; Gokhale 2006). Muscle hernias most commonly occur in the lower limbs, involving the anterolateral compartment. On MR imaging, signs of muscle herniation may be subtle, often consisting of minimal fascial bulging without signal abnormalities (please see also “MRI of Muscle Injuries”). Attempts to increase the conspicuity of hernia and associated fascial defect can be made inviting the patient to repeat a known precipitating exercise prior to imaging and changing position (e.g., from plantarflexion to dorsiflexion) to check the muscle in a relaxed and contracted state (Mellado and Pérez del Palomar 1999). However, isometric contraction cannot be sustained for long time within the magnet without inducing motion artifacts. The diagnosis of muscle hernia with MR imaging remains, therefore, not straightforward, including many false negatives (Fig. 5). On the other hand, US provides a sensitive and elegant way to confirm the clinical diagnosis. US should be performed in the patient’s position most adequate to reveal the lump. In general, examining the patient while squatting to increase the pressure within the anterolateral compartment of the leg, or immediately after strenuous exercise can help increase the visibility of the lump (Bates 2001). Initially, the probe is applied lightly on the skin to avoid reduction of the hernia and a false negative examination. US findings of muscle herniation include focal discontinuity of the fascia and a “mushroom-like” portion of muscle fibers outpouching focally through the defect. Because the hernia is reducible below the fascial defect in most cases, applying graded pressure with the probe over it may give real-time depiction of its intermittent extrusion and relocation (Bates 2001) (Fig. 6). It is not uncommon to demonstrate prominent vessels which traverse the fascia at the site of muscle herniation. Similar to vessels, the abnormal outgrowth of muscle tissue through fascial discontinuities can also determine entrapment of nerve bundles, such as the medial and intermediate dorsal cutaneous nerves. In these cases, patients complain about sensory disturbances over the dorsal aspect of the midfoot and forefoot.
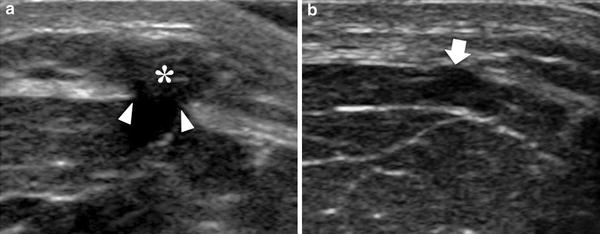
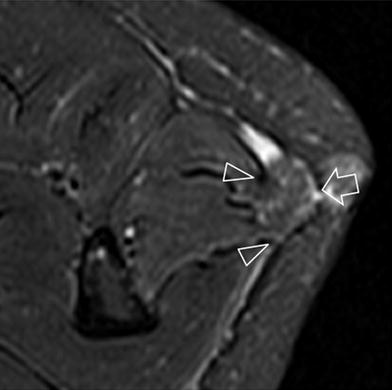
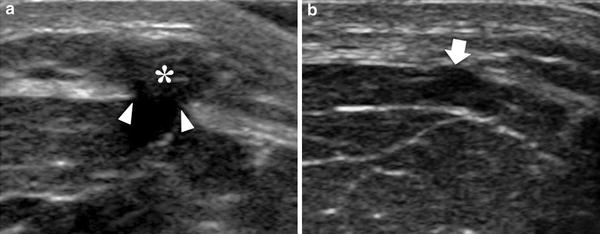
Fig. 5
Muscle hernia. a, b Transverse 12-5 MHz US images obtained with different degrees of probe pressure over a fascial defect (arrowheads) in the tibialis anterior shows a mushroom-like muscle bulge (asterisk) within the subcutaneous tissue. In b moderate pressure applied with the probe effaces the hernia (arrow)
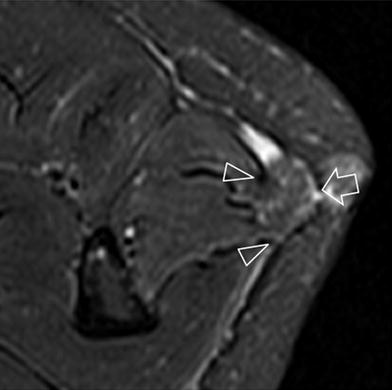
Fig. 6
Posttraumatic muscle hernia. Fat-suppressed Turbo-Spin-Echo T2-weighted MR image reveals herniation (arrow) of the peroneus longus muscle through a wide fascial defect (arrowheads). No signal intensity changes are demonstrated in the herniated and adjacent muscle
7 Compartment Syndrome
The establishment of an acute compartment syndrome is related to an abrupt increase in pressure within a closed muscle- or fascial compartment. Edema following strenuous exercise or blunt injury may lead to increased interstitial pressure within the compartment due to the limited fascial compliance and impaired capillary perfusion up to cause damage to the neuromuscular function units, ischemia, and necrosis (Kirsten et al. 2003). Although blood flow at the capillary level is severely compromised, large arteries remain patent and there are no clinical signs of distal leg ischemia. In this setting, diagnosis and decompressive treatment must be established within a few hours to prevent irreversible damage of the affected muscles (Ulmer 2002). Nevertheless, clinical findings may be equivocal and the available options for the diagnosis (e.g., intracompartmental pressure measurement) are invasive and not always accessible and reliable, so a true gold standard is lacking (Wiemann et al. 2006). Imaging may be contributory but both US and MR imaging have inherent drawbacks in making the diagnosis. At US, initial compartment swelling and fascial bulging may not be clearly recognized. In addition, Doppler imaging is not informative because the ischemic process takes place at the capillary level (beyond the sensitivity threshold of US equipments), whereas large vessels in the compartment look normal even in cases of severe ischemia. Contrast-enhanced US is opening new perspectives as a means to check the level of microvasculature. Analysis of contrast uptake and distribution and intensity-time curve measurements can contribute to increase the diagnostic confidence of US and confirm the need for immediate decompression. US detection of an abnormal echotextural appearance with blurred and less defined visualization of fibroadipose septa indicates progression of ischemia toward irreversible changes and a poor outcome. On MR imaging, signal intensity changes in the affected muscle compartment may be easily seen on T1- and T2-weighted sequences (please see also “MRI of Muscle Injuries”). Due to abnormal cell membrane permeability, the affected compartment strongly enhances after gadolinium administration (Rominger et al. 2004). Problems are related to confounding factors (e.g., atherosclerosis) that may produce misleading findings (Fleckenstein 1998; Kirsten et al. 2003). In addition, swelling and edema may not be easily distinguished from an acute compartment syndrome in a traumatic setting. Given these limitations, one should always take into account that compartment syndrome is an emergency situation and imaging must not delay surgery.
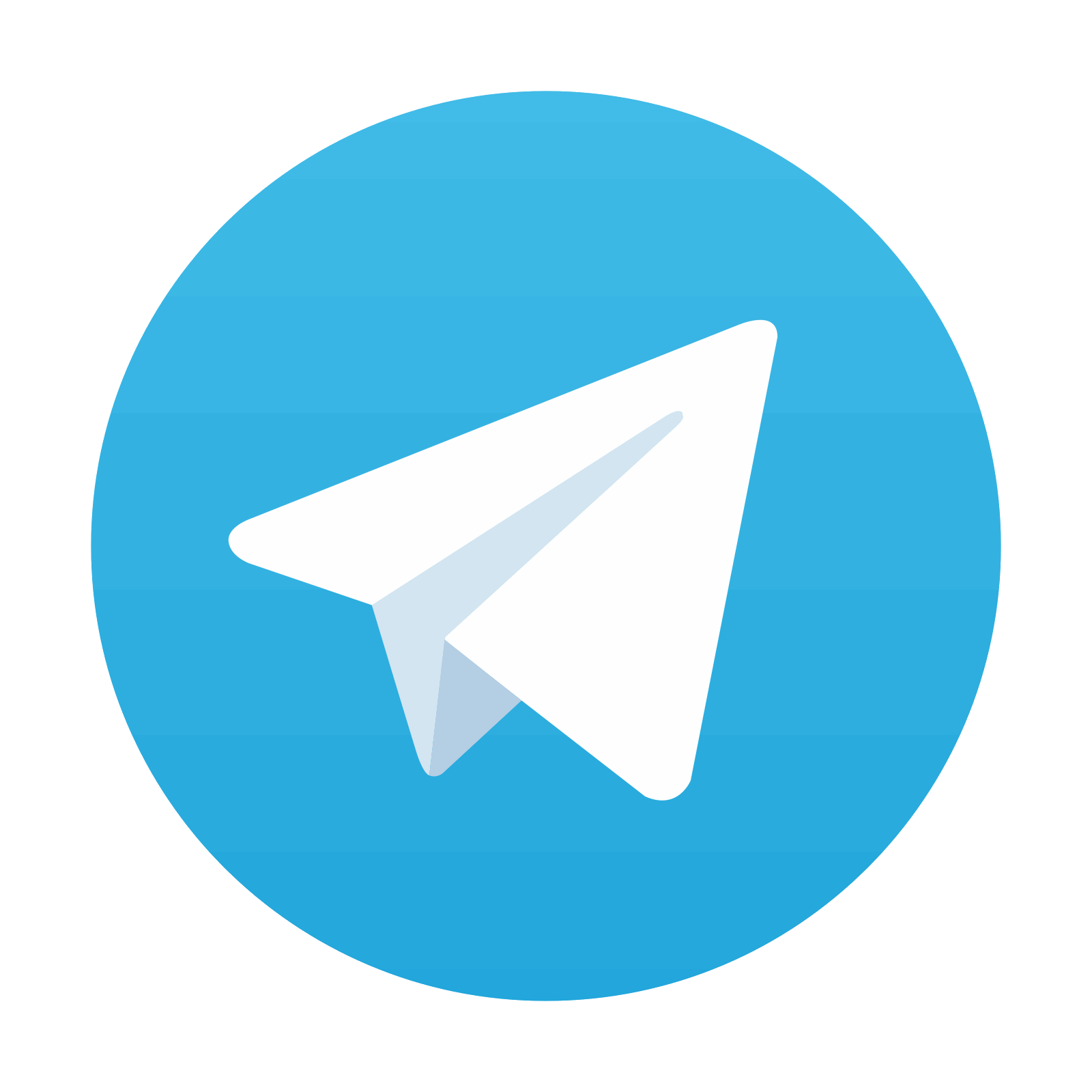
Stay updated, free articles. Join our Telegram channel

Full access? Get Clinical Tree
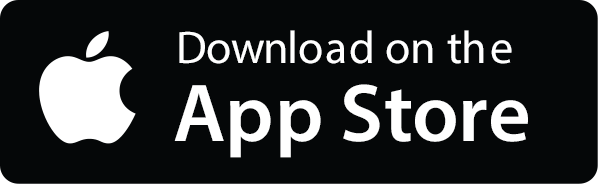
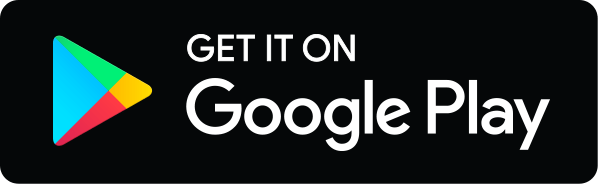