Fig. 3.1
Current methodology contains multiple possibilities of failure to accurately assess margin status. Pre-resection: The initial incision is based upon the surgeons’ best assessment of the tumor margins, however overestimating the cancer results in loss of normal tissue while underestimation results in cutting through the cancer and leaving positive margins. Post-resection: the surgeon samples areas suspicious for cancer but rarely can sample the whole bed. Frozen specimen: the pathologist traditionally assesses only two cuts from a specimen, leaving at least 80 % of the tissue unsampled
Components of a Successful Imaging Probe
Early fluorescent contrast agents consisted of small peptides. By their inherent physical structure, these agents contain some property of attenuation or photon emission that is detectable using a probe or camera. These early fluorescent probes used for diagnostic or localized cancer imaging have been single molecular agents, such as aminolevulinic acid (5-ALA) or indocyanine green (ICG), that localize to disease based on disease-specific characteristics rather than qualities inherent to the imaging agent. Early results using these probes have been promising, however the lack of an established standard for optimal fluorescence leaves the scientific community wondering if these probes are ideal.
Toward that end, a variety of molecular probes have been developed for cancer imaging. Variations that have been introduced and under investigation include receptor ligands [14, 15], nanoparticles [16, 17], receptor ligands [18, 19], antibodies/affibodies directed against cell surface molecules [20–23], and self-quenching probes that are activated by peri-tumoral enzymes or subtle changes in PH [24]. Although preclinical data support use of many of these agents, the translational hurdles posed by consistent manufacturing, costs associated with toxicology studies/IND submission, and safety concerns have severely limited the translation of these agents in humans. Importantly, industry is reluctant to support translation of imaging agents into the clinic because revenue associated with diagnostic agents is only a fraction of therapeutic agents with a similar initial investment for FDA approval [25]. Although some optical agents have been evaluated in phase I clinical trials [13], they are not readily applied to other tumor types or carry high costs of adoption.
Antibodies conjugated to fluorescent molecules can provide a viable approach for cancer imaging. FDA-approved antibodies that are repurposed for cancer localization can act as a vector for fluorescence imaging. These actively targeted complexes provide the specificity required to differentiate disease from normal tissue since they are specifically designed to target disease characteristics that are aberrantly expressed relative to normal tissue. In most imaging modalities, successful imaging is not solely dependent upon high tumor accumulation, but the tumor-to-normal ratio of accumulation. This ratio defines the amount of contrast generated between tissues during imaging, which determines the successful use of fluorescence imaging to assist surgical navigation. Antibodies previously approved for cancer therapy target these aberrantly expressed markers, which are innate to cancer but not normal tissue. When combined with fluorescent molecules, cancer-specific probes (Fig. 3.2) produce robust contrast between disease and normal tissue. Importantly, these combinations can be modified to incorporate advanced components as they become available to improve disease visualization.
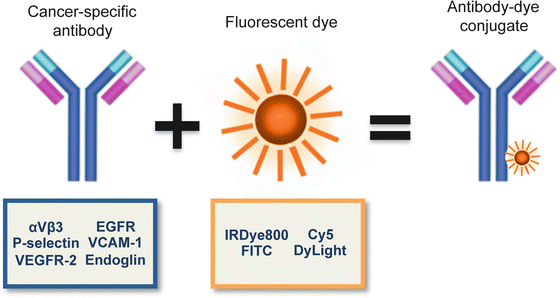
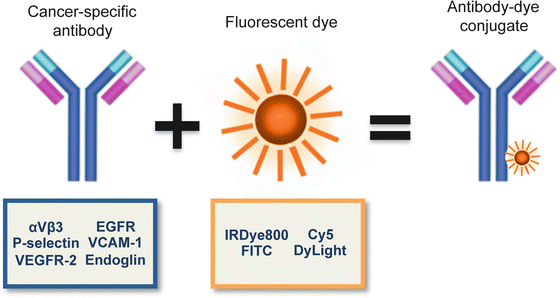
Fig. 3.2
Therapeutic antibodies that target cancer-specific markers can be conjugated to fluorescent molecules to yield a vector for fluorescence imaging of cancer
Cancer-Specific Targeting Using Monoclonal Antibodies
Currently, there are multiple antibodies which are approved for human use by the FDA [26] for the treatment of solid and blood-based cancer (Table 3.1), with many more in various stages of clinical trial. Although radiolabeled and fluorescently labeled antibodies have been used in early phase clinical trials, there is insufficient published data on which antigen will emerge as the optimal imaging target. There are several important properties of an antibody target that would allow accurate imaging of the tumor for detection or for surgical guidance. For example, antigens expressed on the cancer cell surface (EGFR or Her2) would permit greater tumor-specific localization compared to those within the extracellular milieu (VEGF). Realistically, the ideal agent will depend on factors specific to each cancer, but the ideal target would also be broadly applicable to a wide range of cancers. Antibodies that have been assessed in early stage clinical trials for imaging include cetuximab (ClinicalTrials.gov Identifier: NCT01987375) and bevacizumab (ClinicalTrials.gov Identifier: NCT01508572).
Table 3.1
Antibodies approved for the treatment of cancer
Antibody | Target | Isotype | Approval | Cancer |
---|---|---|---|---|
Alemtuzumab (Campath) | CD52 | Humanized IgG1 | 2001 (US) 2001 (EU) | Chronic lymphocytic leukemia |
Bevacizumab (Avastin) | VEGF | Humanized IgG1 | 2004 (US) 2005 (EU) | Glioblastoma, non-small cell lung cancer |
Brentuximab (Adcetris) | CD30 | Chimeric IgG1 | 2011 (US) | Lymphoma |
Cetuximab (Erbitux) | EGFR | Chimeric IgG1 | 2004 (US) 2004 (EU) | Colorectal, head, and neck |
Ibritumomab (Zevalin) | CD20 | Murine IgG1 | 2002 (US) 2004 (EU) | Lymphoma |
Obinutuzumab (Gazyva) | CD20 | Humanized glycol-engineered | 2013 (US) | Lymphoma |
Ofatumumab (Arzerra) | CD20 | Human IgG1 | 2009 (EU) | Chronic lymphocytic leukemia |
Panitumumab (Vectibix) | EGFR | Human IgG2 | 2006 (US) | Metastatic colorectal |
Pertuzumab (Perjeta) | Her2 | Humanized IgG1 | 2012 (US) | Breast |
Rituximab (Rituxan) | CD20 | Chimeric IgG1 | 1997 (US) 1998 (EU) | Lymphoma |
Tositumumab (Bexxar) | CD20 | Murine IgG2a | 2003 (US) | Lymphoma |
Trastuzumab (Herceptin) | Her2 | Humanized IgG1 | 1998 (US) 2000 (EU) | Breast |
An additional consideration is that the fluorescent signal could be enhanced if the prolonged circulating time of the antibody leads to greater antibody–receptor complex internalization. This receptor cycling leads to greater cellular incorporation of the fluorescence molecule over time, leading to greater signal amplification in those tissues containing the targeted receptor, but not in normal tissues. Whole antibody conjugates would therefore have an inherent advantage over antibody fragments or small peptides due to their longer circulatory half-life, which in some cases can be many folds higher. Preliminary in vitro data and murine xenograft models suggest that the absolute expression level of the antigen may not be as important as the cellular incorporation or the permeability of the microvasculature [27, 28]. However, the conventional dogma has held that the targeted antigen itself should be widely expressed on tumor cells but not on normal tissues, should have equal distribution throughout the tumor, and expression levels relatively consistent between patients.
For use in fluorescence-guided surgery, it has commonly been assumed that the ideal imaging vector would allow tumor imaging immediately after systemic administration of the diagnostic agent. However, the half-life of whole antibodies is usually measured in days when given as a therapeutic agent over many doses while the pharmacokinetics are non-linear such that when administered as a single dose the half-life is significantly shorter. Therapeutic antibodies administered at diagnostic levels have a half-life around 24 h, which will likely require administration several days before imaging to permit adequate tumor-specific accumulation and subsequent clearance from the circulation. This will produce the greatest amount of fluorescence contrast between tumor and normal tissue. Additionally, animal data of xenograft tumors suggest that the tumor to background ratio continues to increase over weeks as the background decreases [6].
The toxicity of the agent should also drive selection of the appropriate antibody for imaging. Because the acceptable range of toxicity for imaging agents is lower compared to anticancer agents, the antibody should have a limited toxicity profile when delivered in low doses. Several antibody-related toxicities have been reported at therapeutic dosing, especially after repeat dosing, however, the immediate infusion reactions and true anaphylaxis will occur after a limited administration of the drug. Infusion reactions are more commonly associated with chimeric antibodies as opposed to fully human antibodies. Recent studies have shown that at low molar ratios [2] liver clearance of antibodies is largely unchanged, and other studies in non-human primates have demonstrated that the antibody–dye conjugate has the same pharmacokinetics of the antibody alone [1, 2]. An antibody–dye agent currently being investigated in patients is shown in Fig. 3.3.
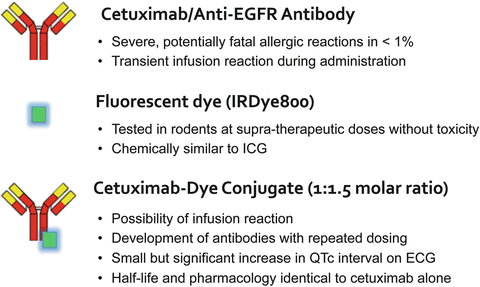
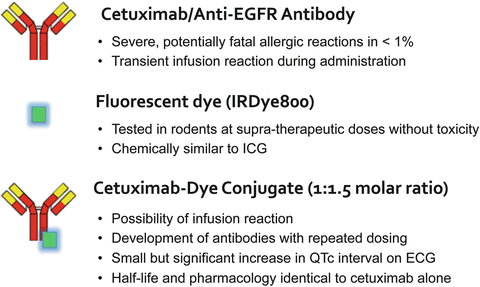
Fig. 3.3
The addition of the fluorescent probe to an antibody is not associated with significant toxicity in non-human primate studies [1]
Selection of a Fluorescent Molecule
Most fluorescent molecules can be linked to an antibody, however the extent of modification will determine the immune response and clearance rates of the bioconjugate. There are a variety of fluorescent probes that can be conjugated to proteins through relatively simple covalent chemistry at consistent molar ratios (Fig. 3.2). Optical dyes are dependent on the properties of light and are therefore limited in depth of tissue penetration. While attenuation and scattering are consequential artifacts, optical imaging affords high resolution when imaged superficially. In the surgical setting, cancer-containing tissues are exposed and overlying tissue, which could attenuate fluorescence signal, is debulked or removed. Fluorescence agents are therefore well suited for the surgical arena, where high-resolution imaging can be applied in real-time. Furthermore, optical probes can be designed to emit fluorescence and toxic reactive oxygen species after light-based activation. This theranostic imaging strategy, referred to as photoimmunotherapy (PIT), can be used to optically resect the tumor and simultaneously treat residual microscopic disease.
There are several factors to consider before selecting the optimal dye for fluorescence-guided surgical navigation. Examples of fluorescence molecules currently under investigation for use in image-guided surgery are listed in Table 3.2. The labeling procedure must be standardized so that the dye-to-protein (D:P) ratio remains consistent between conjugation procedures. Often, the optimal D:P ratio is discovered from a balance between unaltered pharmacokinetics and robust signal emission for each antibody conjugated. Anther factor to consider is the absorption and emission wavelengths. Excitation and emission wavelengths in the near infrared range (>700 nm) permit greater tissue penetration (1 cm) with less scattering and attenuation. In addition, properties of the fluorescent molecule will determine the appropriate hardware to use for imaging. This has significant impact on clinical translation and also the type of surgical wound bed that can be imaged (laparoscopic versus open field imaging). Certain nanoparticles and other novel substances have significant toxicity or antigenic properties and their application should be clearly justified prior to serious efforts toward clinical translation.
Table 3.2
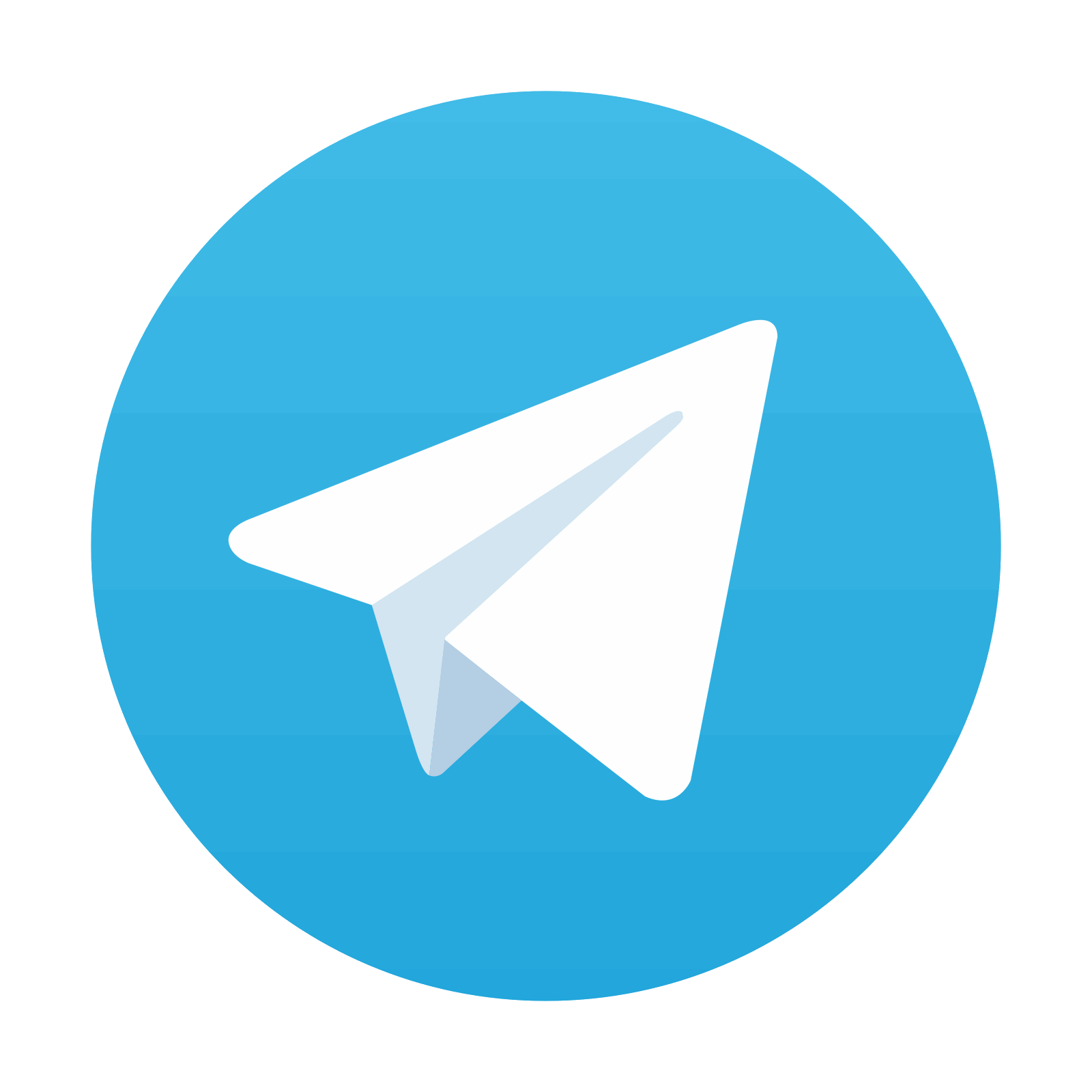
Fluorescent dyes currently under investigation
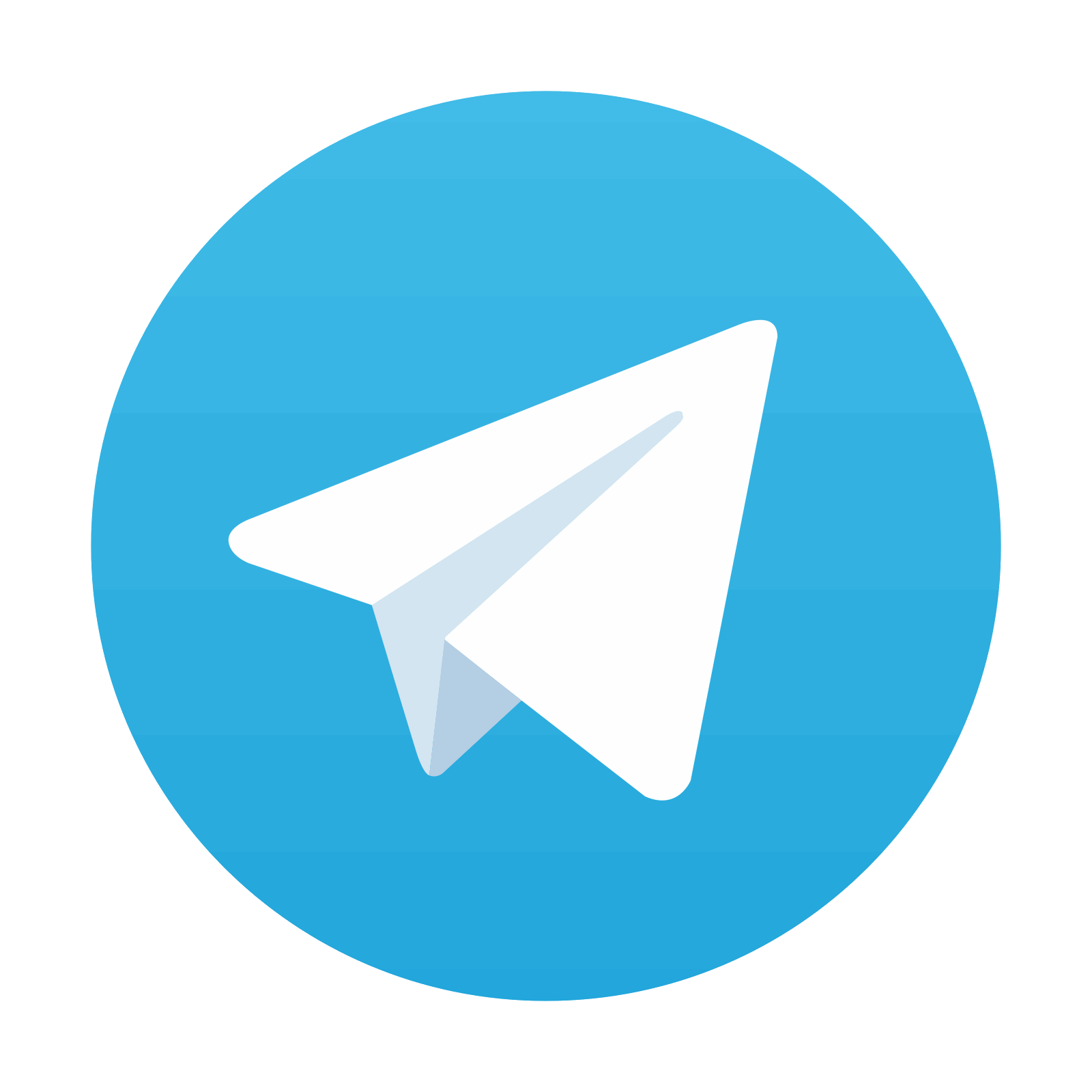
Stay updated, free articles. Join our Telegram channel

Full access? Get Clinical Tree
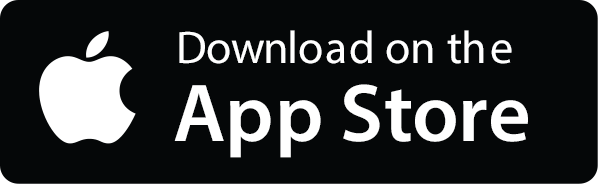
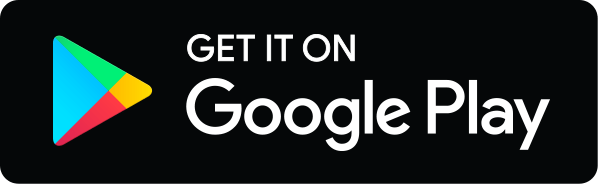
