Abstract
This chapter reviews basic physics principles behind medical ultrasound (US). Six components are discussed: the stimulus (the US pulse), the object (the human body), the object’s response to the stimulus (the echo), the source of the stimulus and the response detector (the US transducer), and the image generator (the scanner). These components are used as a frame of reference to explain important concepts such as descriptors of the US pulse (frequency and amplitude); pulse-echo signal generation; attenuation, reflection, and scattering of US energy; speckle and echogenicity; and image quality in terms of spatial, temporal, and contrast resolutions. Also addressed is the trade-off between image quality and acoustic energy deposition.
Keywords
ultrasound, pulse, physics, frequency, resolution
Introduction
Since its introduction to the medical community in the 1950s, ultrasound (US) has become a widespread imaging modality because of its portability, nonionizing energy, and relatively low cost. This chapter reviews basic physics concepts behind the formation of an US image, emphasizing conventional B-mode imaging. Other approaches, including US-based elasticity assessment, Doppler, and three-dimensional imaging, as well as magnetic resonance imaging, are discussed in other chapters.
Components of an Imaging System
In an imaging system, a source produces a stimulus that interacts with the object to be imaged. That interaction produces a response within the object, which is sensed by a detector, and transformed into a signal that an image generator uses to produce the image.
The Stimulus
In a clinical US system, the stimulus is a US pulse. This is a short disturbance (about one-millionth of a second) that progressively moves from the source (the US transducer, described subsequently) through the object (the human body). This disturbance consists of a sound wave, which is a series of pressure increments and reductions that cause small volumes (approx. 0.1 mm 3 ) of tissue to sequentially shrink (compression) and expand (decompression, also called rarefaction). One compression followed by one rarefaction is called a cycle, and a typical US pulse might contain between one and four cycles.
The amplitude of the wave can be described by the maximum compression achieved by the pulse. The frequency is the number of compression and decompression cycles that a small volume experiences per second. The unit “one cycle per second” is called a Hertz (Hz). Humans are able to hear sound waves with frequencies between about 20 Hz and 20 kHz. The prefix ultra – refers to sound waves with frequency beyond the audible range, i.e., higher than 20 kHz. Frequencies in diagnostic obstetric US span roughly 1 to 10 MHz.
The volume occupied by the US pulse (pulse size) is about 0.25 mm 3 and is defined in three dimensions: the axial length, the lateral width, and the elevational width (crosshairs in Fig. 169.1 ). The axial dimension is parallel to the propagation direction of the pulse and is called the pulse length, i.e., the extent occupied by adjacent compression/decompression zones within the pulse (about 0.5 mm). The lateral and elevational dimensions are perpendicular to the propagation direction—one within and one outside of the image plane, respectively. These dimensions are on the order of 1 mm and vary with the distance of the US pulse from the source, with the US beam geometry having an hourglass shape (see dashed blue lines in Fig. 169.1 ) determined by the size of the source (see red area in the transducer in Fig. 169.1 ). The depth at which the lateral and elevational dimensions are the smallest is the focus (see green mark in Fig. 169.1 ).
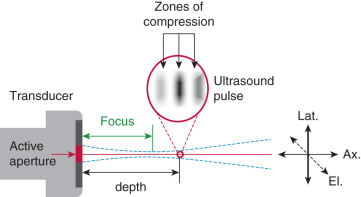
The Object
The object with which the US pulse interacts is the human body. The pulse travels within the object along a well-defined path from the source, the propagation axis, represented by the red line in Fig. 169.1 . To the pulse, the body is a collection of materials with different acoustic impedances. The acoustic impedance is a measure of the resistance of a material to compress and decompress under the action of the US pulse (how much the material impedes the propagation of the wave). Because acoustic impedance is related to a tissue’s density and stiffness, it informs the speed at which the pulse travels. Specifically, a pulse will move faster in tissues with larger impedance (tendon, about 1750 m/s) than in those with lower impedance (fat, about 1400 m/s). The average sound speed in soft tissue is 1540 m/s.
The Response
The tissue response from which US signals and images are created is called an echo . An echo is created when the pulse reaches an interface between two materials of different acoustic impedance. The amplitude of the echo depends on three main factors: the properties of the impedance interface, the amplitude of the ultrasonic pulse when it reaches the interface, and the energy lost by the echo on its way back to the detector. The larger the amplitude of an echo, the brighter it appears in the image.
The main property of the impedance interface that defines the echo’s amplitude is its physical dimensions compared to the size of the US pulse. If the size of the impedance interface is significantly larger than that of the pulse, the interface acts as a (partially reflective) mirror that reflects the pulse. The stronger the difference in acoustic impedance at either side of the interface, the larger the fraction of the incident pulse amplitude in the echo. Echoes from large interfaces provide the anatomic landmarks of conventional US images. For clinical imaging, gels or oils are used to couple the surface of the US transducer to the patient’s skin to avoid a large impedance difference between air and tissue. This coupling creates an acoustic “window” into the body.
Once in the tissue, the pulse encounters scatterers, which are microstructural elements such as collagen bundles or cells that create a set of minute changes in acoustic impedance on a physically small size compared to the pulse. Each of these redirects the pulse with smaller amplitude. All the scattered pulses within the volume occupied by the US pulse interact with each other to generate a total echo. The amplitude of the total echo depends on the number of scatterers and their spatial distribution within the US pulse, as well as their impedance difference relative to the surrounding tissue. Most of the US appearance of tissue parenchyma is produced by scatterers that are poorly organized, e.g., collagen bundles. As a result, the amplitude of the total echo varies from location to location, creating a ubiquitous “noise” known as speckle . The term echogenicity refers to the overall brightness of speckle from tissue parenchyma. The terms hypoechoic , isoechoic or hyperechoic describe a region of the image that looks less, equal, or more echogenic than its surroundings, respectively.
Other properties that define the amplitude of the echo signal are the amplitude of the pulse that reaches a particular location within tissue. This is affected by tissue attenuation. Attenuation is the exponential loss of energy that the US pulse experiences as it travels through tissue. This loss is caused by reflection and scattering as well as absorption (the conversion of acoustic energy to heat). Attenuation is usually quantified in the amount of energy lost by the pulse as it traverses 1 cm of tissue, expressed in decibels, which is defined as 10 times the logarithm of the energy loss. For instance, an energy loss of 50% corresponds to 10 × log (0.5) = 3 dB. Higher frequencies attenuate more than lower frequencies, and most tissues have attenuation between 0.5 and 1 dB/cm/MHz. The attenuation-related loss of US signal with depth can be partially compensated by amplifying echoes coming from deeper locations through adjusting control knobs (time-gain compensation) on the imaging system.
Finally, the amplitude of the echo itself has both a geometric and a physical component. The geometric component is related to the sensitivity of the detector to the spatial location at which each echo is generated. The spatial pattern of sensitivity is similar to the focused beam geometry that determines the size of the US pulse. The physical component is also caused by attenuation.
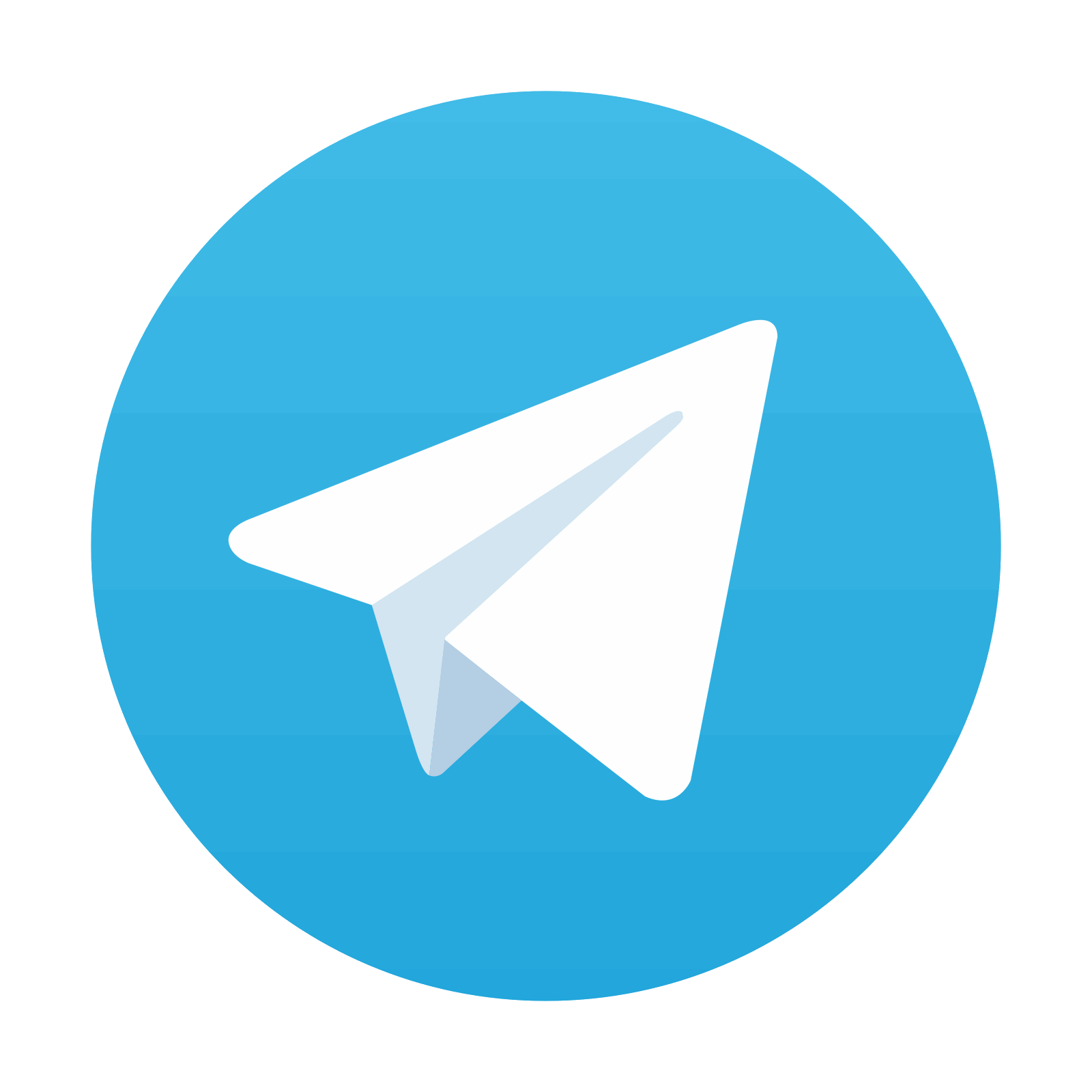
Stay updated, free articles. Join our Telegram channel

Full access? Get Clinical Tree
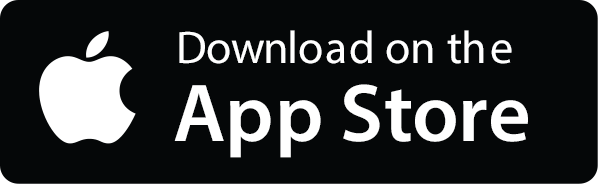
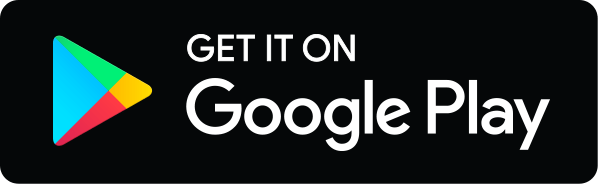