7 Use of MRI in Acute Spinal Trauma
Contents
Indications for the Use of MRI in the Presence of Spinal Trauma
MRI and Acute Traumatic Epidural Lesions
Intervertebral Disk Herniation
MR Angiography and Injuries to the Cervical Arteries
Advantages of MRI
Major advantages of MRI over other techniques include:
• The possibility of obtaining images in all three planes.
• Superior contrast resolution compared with other modalities, providing a higher detection rate for soft-tissue injuries, particularly the spinal ligaments.
• The possibility of generating myelographic-equivalent images to assess the epidural space for evidence of hematoma, bone fragments, herniations and osteophytic changes without the need for administering contrast.
• Contrast-rich imaging of the spinal cord to allow detection of contusion, hematoma or laceration.
• Reliable prognostic information regarding potential for recovery of function based on the MRI appearance of spinal cord injuries.
• The possibility of imaging the paravertebral vessels without the need for contrast application, providing instead various pulse sequences to impart either positive or negative contrast of the vessels.
• MRI is performed without ionizing radiation and is thus free of side effects.
Fig. 7.1 Normal sagittal T1-weighted SE image of the cervical spine. The image displays the spinal cord with moderate signal intensity, surrounded by CSF with less signal intensity. The vertebral bodies show relatively high signal intensity on account of the fatty marrow. The anterior longitudinal ligament and the ventral portion of the annulus fibrosus appear as structures with low signal intensity (arrows) and are therefore well delineated from the higher signal of the disk and prevertebral tissue. The posterior longitudinal ligament and the annulus on the other hand are, together with the ligamentum flavum, somewhat poorly demarcated due to the low contrast relative to CSF.
A variety of imaging sequences are required to provide an exact portrayal of both normal anatomy and the various pathological changes. Some new sequences have recently been introduced to improve further the diagnostic reliability of the examinations and reduce scanning time.
The following scanning regime is generally employed
Sagittal T1-weighted SE sequence to highlight basic anatomy (Fig. 7.1).
Sagittal PD- and T2-weighted SE (or TSE/Fast-Spin-Echo) sequence to identify pathological changes (hemorrhage/edema) or ligament structures (Fig. 7.2).
Sagittal GRE sequence to optimize identification of hemorrhage and to differentiate between osteophytes and disk material. Axial T1-weighted SE sequence to assess the epidural space, spinal cord and the foramina through areas of interest identified on the sagittal sections (Fig. 7.3).
Axial GRE sequence to assess spinal cord anatomy, blood vessels (bright signal) and ligaments (Fig. 7.4).
Optional MR angiography sequence to assess the cervical vessels, depending on the mechanism of injury suffered by the patient (Fig. 7.5).
Fig. 7.2 Normal sagittal T2-weighted SE image of the cervical spine. The image displays CSF with a very high signal in contrast to the moderate signal intensity of the spinal cord. The signal of the vertebral body is lower than on the T1-weighted image, while the intervertebral disks appear brighter because of the high signal of the nucleus. The posterior longitudinal ligament with the annulus (black arrows) and the ligamenta flava (white arrows) are well delineated due to the adjacent structures, which demonstrate higher signal intensity. The dark line in the center of the spinal cord represents an artifact along the phase-encoding direction (Gibbs or truncation artifact) (with permission Mirvis S.E.: Semin. Musculoskelet. Radiol. 1998, 2 27–43).
Fig. 7.3 Normal axial T1-weighted SE image through the mid-third of the cervical spine. This image shows the spinal cord surrounded by low-signal CSF. The exiting nerve roots (white arrows) are well delineated as they approach the intervertebral foramina (black arrowheads). Blood flowing in the vertebral arteries appears dark (flow void phenomenon; black open arrows). Cortical bone appears uniformly dark (with permission Mirvis S.E.: Semin. Musculoskelet. Radiol. 1998, 2 27–43).
Fig. 7.4 Normal axial T2*-weighted GRE image through the mid-third of the cervical spine. This image shows the internal architecture of the spinal cord (the gray matter appears somewhat brighter than the white matter and displays the typical butterfly- or H-shaped configuration). The myelinated tracts in the periphery of the cord have a lower signal intensity. Bony structures appear relatively dark. The dura (arrowheads) is well delineated from the hyperintense CSF as a dark line.
Fig. 7.5 Normal time-of-flight angiography of the cervical vessels. Flowing blood demonstrates a high signal intensity using this two-dimensional GRE time-of-flight technique. The right vertebral artery appears hypoplastic. The horizontal part of the vessel above the loop of the vertebral artery (arrow) shows complete loss of signal due to in-plane signal saturation effects — a typical phenomenon. The suppression of the venous signal is achieved by a saturation pulse applied above the cervical region (with permission Mirvis S.E.: Semin. Musculoskelet. Radiol. 1998, 2 27–43).
Another sequence, the IR sequence, is also recommended to identify ligament injuries and to distinguish osteophytes from bulging or herniated disks.
Recently, three-dimensional volume acquisition of MR data has been introduced and offers the potential of acquiring the entire cervical region in one scanning procedure and reformatting images through any plane with the resolution of a direct two-dimensional scan. The procedure is particularly useful for exact imaging of the foramina, although with agitated patients image quality is very limited (Ross, 1992; Georgy and Hesselink, 1994).
Echo planar imaging (EPI) or other fast imaging sequences can be useful when particularly short acquisition times are required. TSE and fast SE (FSE) sequences are associated with a significant reduction of acquisition time and allow the use of particularly long TR and TE times to achieve more heavily T2-weighted images and improve visualization of the nerve roots within the spinal canal on axial sections (Gundry and Fritts, 1997). TSE sequences also have advantages when imaging patients who have metallic hardware after surgery because metal-induced artifacts appear less pronounced due to decreased magnetic susceptibility effects. However, there is one disadvantage in that these sequences may emphasize CSF motion artifacts (Gundry and Fritts, 1997).
Both GRE and TSE (FSE) sequences are less sensitive in portraying bone-marrow edema than T2-weighted sequences. The combination of TSE (FSE) sequences with fat suppression techniques produces particularly contrast-rich MR myelograms that succeed very well in depicting extradural defects of the nerve roots sleeves. Furthermore, the extent of spinal stenosis can be much better assessed on these images.
Disadvantages of MRI
Disadvantages of MRI include:
• Bone is only poorly demarcated because it contains no hydrogen atoms. Only bone marrow containing fat and blood is depicted as hyperintense. Consequently, only major injuries to the bone are reliably shown on MRI, which should therefore not be considered a sensitive technique for detecting subtle bone injury, particularly injury involving the posterior vertebral elements (Schroeder et al., 1995).
• Currently, an MRI scan of the spine requires somewhat more time than comparable CT studies because of longer data acquisition. However, new imaging acquisition sequences are rendering MRI almost as fast or even faster than CT.
• Hemodynamically unstable patients should not be examined acutely by MRI because cardiopulmonary resuscitation is considerably more difficult to perform in the MR scanning room due to problems associated with the magnetic field.
• Exact monitoring requires MR-compatible supervisory systems that can be used without restriction in the magnet room and without creating radiofrequency noise, which interferes with the image acquisition process (Shellock et al., 1993, 1995).
• The development of non-magnetic neck braces, patient transport systems and remote telemetric monitoring systems now allow MR examination of patients with acute spinal injury, in the majority of cases. However, patients with certain types of ferromagnetic intracranial aneurysm clips, cochlear implants and pacemakers must still be excluded from MR scanning and should be kept away from the magnetic field. Furthermore, patients with metal foreign bodies in the immediate vicinity of vital soft-tissue structures such as the spinal cord, nerve roots, or the orbita are at risk while undergoing MR examination, especially when these metallic foreign bodies show no scar-tissue formation within the tissue (Shellock, 1993). If there is any uncertainty whether the patient has in fact been exposed to metallic foreign bodies, e.g. in the case of welders, then radiographic screening should be performed prior to MR examination.
• It may be assumed that, due to severe claustrophobia, 3 % of patients can only be examined under sedation (Katz et al., 1994).
Indications for the Use of MRI in the Presence of Spinal Trauma
The use of MRI in spinal trauma has clearly increased with improved technology, especially with regard to reduced screening time as well as the introduction of MR-compatible devices for patient positioning and monitoring:
• Today MRI is indicated in the evaluation of all patients with a neurological deficit after spinal injury, especially to the cervical region, when permitted by the patient’s overall clinical state and in the absence of any absolute contraindications.
• MRI is particularly indicated in patients with an incomplete or progressive neurological deficit related to spinal trauma.
• Patients with a complete neurological deficit should also undergo MRI assessment if decompression is being considered in an attempt to promote nerve root recovery.
• A further indication for MRI of the spine is given in patients with myelopathy or radiculopathy after spinal trauma in whom previous radiological or CT studies have been negative and in cases where the level of the neurological deficit does not correlate with clinical evidence of location.
• MRI is also useful in deciding the need for internal fixation and the most appropriate surgical approach because MRI best assesses the level or levels of potential mechanical instability secondary to ligament injury, or the presence of spinal-cord compression from epidural processes such as disk herniation or reactive osteophytes.
MRI and Soft-Tissue Injuries
MRI is capable of demonstrating acute injuries to soft tissues secondary to spinal trauma with high contrast resolution and can easily differentiate edema, hemorrhage and direct spinal cord injury. Cord contusions are usually non-hemorrhagic and appear isointense or slightly hypointense relative to the normal cord on T1-weighted SE images, but become brighter in signal intensity than the normal cord on the T2-weighted SE or GRE image sequences (Figs. 7.6–7.9).
Fig. 7.6 Contusion of the cervical cord. The sagittal T2-weighted image shows a hyperflexion fracture of C5 vertebral body with loss of anterior height. The high signal intensity in the vertebral bodies of C5 and C6 is due to marrow edema and hemorrhage, indicating that both vertebral bodies have been injured. The increased signal within the spinal cord at the levels C4 and C5 (arrows) is an indication of contusion-related edema. In addition, a prevertebral edema is apparent, recognizable by the bright signal anterior to the spine along C2–C6.
When there is significant hemorrhage present within the cord, its appearance on MRI depends on the degree of blood degradation, the field strength of the magnet as well as the imaging sequence used. In the acute to subacute phase after trauma, which ranges approximately from days 1 to 7, blood generally appears dark on the T2-weighted SE images, with this reduction in signal becoming even more intensified on T2*-weighted GRE sequences. Edema, on the other hand, displays a bright signal on T2-weighted images (Figs.7.10 and 7.11).
Fig. 7.7 Spinal cord contusion. The sagittal T2-weighted SE image shows increased signal intensity within the cord at the level C3/C4 (open arrow). A hyperextension mechanism of injury is indicated by the ventral rupture of the anterior longitudinal ligament at the C5/C6 level (arrow) and the widening and signal enhancement within the disk space C5/C6. There is a disk herniation and hypertrophy of the ligamentum flavum at the C3/C4 level, which have contributed to the contusion of the spinal cord at this level. This image shows a multilevel spinal stenosis at C3–C7. Note, furthermore, the extensive prevertebral edema from C1 to the inferior margin of C4.
After about 7 days, when the erythrocyte cell membrane has broken down, extracellular blood develops high signal intensity on both T1- and T2-weighted SE images.
Kulkarni et al. (1987) discovered a relationship between the characteristics of signal changes in the spinal cord and the patient’s neurological prognosis, suggesting that the signal pattern of the cord on MRI reflects actual cord histopathology.
Fig. 7.8 Contusion of the cervical cord. The sagittal T2-weighted SE image in a patient who had sustained cervical spine injury displays increased signal intensity in the cord at C3/C4 level due to cord edema (open arrow). A hyperextension mechanism is assumed to be the cause and is indicated by the signal interruption of the anterior longitudinal ligament and annulus fibrosus at the C3/C4 level (arrow). Note the marked prevertebral edema (with permission Mirvis S.E.: Semin. Musculoskelet. Radiol. 1998, 2 27–43).
Fig. 7.9 Contusion of the cervical cord. T2-weighted TSE sequence in a patient with a teardrop fracture at the C5 level secondary to a hyperflexion trauma. The image demonstrates extensive cord edema in the C4–C6 segment (white arrows). There is also a circumscribed structure with reduced signal intensity recognizable at the C5 level, compatible with intramedullary hemorrhage associated with deoxy- and methemoglobin components (arrowheads). Moderate prevertebral edema.
Fig. 7.10 Contusion of the thoracic cord. Sagittal T2-weighted image in a patient who sustained paraplegia after thoracic hyperflexion injury. The image shows an intramedullary edema at the mid-thoracic level. The ligamentum flavum, which usually displays a low signal intensity, appears disrupted at this level (open arrow). The high signal intensity seen dorsally at several adjacent levels suggests injury of the dorsal soft tissue located in the region of the spinous process (with permission Mirvis S.E.: Semin. Musculoskelet. Radiol. 1998, 2 27–43).
Fig. 7.11 Spinal cord injury with development of edema and hemorrhage. Sagittal T2-weighted image in a patient with a bursting fracture of C5. The image shows a high signal intensity of the cord edema and a low signal in the center of this edematous zone (white arrow) indicating hemorrhage at the C4/C5 level. There is also marrow edema recognizable at C5 and C6 in the region of the vertebral bodies. Extensive prevertebral edema is also present. Retropulsion of C5 has resulted in cord injury. The posterior longitudinal ligament has been separated from the posterior cortex of C6 and is shown as a dark epidural line (arrowhead) (with permission Mirvis S.E.: Semin. Musculoskelet. Radiol. 1998, 2 27–43).
Fig. 7.12a, b Spinal cord injury with extensive hemorrhage. 28-year-old patient with tetraplegia secondary to a C5 teardrop fracture. The images show decreased signal intensity in the center of the spinal cord due to intracellular methemoglobin and deoxyhemoglobin with surrounding peripheral edema. The GRE sequence is more sensitive to field inhomogeneity created across the intact erythrocyte membranes and producing larger areas of signal loss. Edema is present in the C4/C5 disk and anterior C5 body:
a Sagittal T2-weighted SE image.
Types of spinal cord injury according to Kulkarni et al.
Type I with hematoma within the cord.
Type II with cord edema (Figs. 7.6–7.9).
Type III with a mixed pattern of edema and hemorrhage (Figs. 7.11–7.13).
The significance of these different signal changes within the spinal cord with regard to the patient’s prognosis has been verified by several other studies (Mirvis et al., 1988; Schaefer et al., 1992; Silberstein et al., 1992; Mascalchi et al., 1993). Patients with cord lesions containing hematoma generally have a poorer prognosis regarding recovery of neurological function than those with only edema within the cord.
The fact that the MRI finding to a certain extent reflects the histopathology of acute cord injury has been confirmed by direct comparison in experimental studies (Weirich et al., 1990; Quencer et al., 1992).
Fig. 7.13 Edema and hemorrhage within the lower thoracic cord. Sagittal T2-weighted TSE image at the dorsolumbar level in a 31-year-old patient with a complete transverse spinal lesion at the lower thoracic level. Edema with central hematoma at the T10/T11 level is recognizable on the image, as well as disruption of the ligamentum flavum (black arrowhead) and the annulus-posterior longitudinal ligament complex (white arrow) (with permission Mirvis S.E.: Semin. Musculoskelet. Radiol. 1998, 2 27–43).
MRI and Ligament Injuries
The mechanism of injury as well as the fracture pattern and alignment of the vertebrae will allow inferences to be drawn on the type of ligament injury sustained from acute spinal trauma. However, even significant ligament injury leading to mechanical instability can go undetected radiographically, particularly in the case of hyperflexion and hyperextension subluxation without concurrent fracture. This is particularly so if concurrent fractures are absent and if the patient is examined radiographically in the supine position, which produces a neutral position or slight extension of the spine. Furthermore, the vertebral alignment shown on plain radiographs may only reveal the main site of mechanical instability, but not demonstrate all segments with ligament injuries and other sites of immediate or potential mechanical instability (Warner et al., 1996).
MRI displays ligaments as structures of low signal intensity due to their lack of mobile hydrogen atoms. Ligament disruption will be seen on MRI as an abrupt interruption of this low signal band, as well as showing thinning or stretching of the ligaments, avulsion of the ligament from its bony attachment, or the combination of a torn ligament with an avulsed bone fragment (an osseous-ligament complex) (Figs. 7.14–7.21) (Silberstein et al., 1992; Warner et al., 1996; Saifuddin et al., 1996; Benzel et al., 1996; Terk et al., 1997).
The changes to the ligamentous structures of the spine, found at MRI, have a definite bearing on subsequent therapeutic approaches.
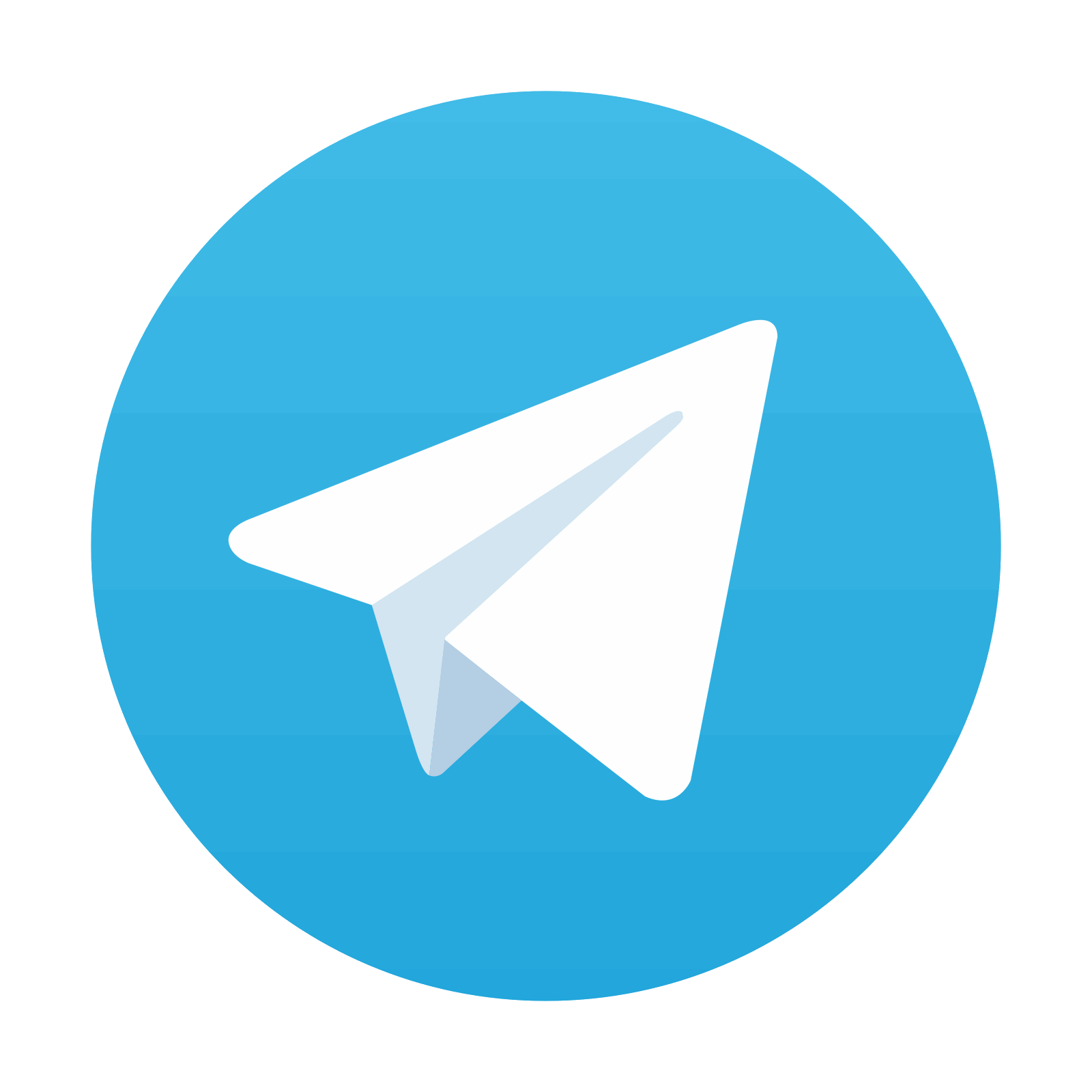
Stay updated, free articles. Join our Telegram channel

Full access? Get Clinical Tree
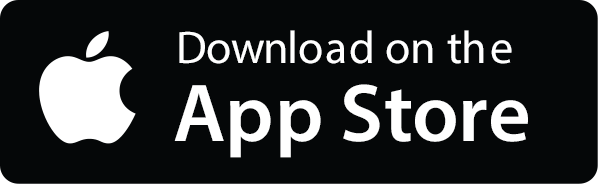
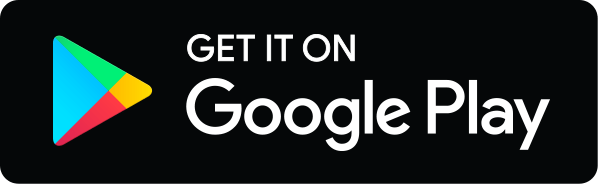