11 Cytoreductive surgery has been the first step in the treatment of glial tumors for decades. Even though malignant, high-grade gliomas might be distinguished macroscopically from healthy brain parenchyma more easily than low-grade gliomas in the majority of cases, intraoperative visualization of tumor remnants frequently remains difficult. Even in dedicated neurosurgical centers, these difficulties account for tumor tissue visible on early postoperative magnetic resonance imaging (MRI) scans in a number of patients.1,2 It is a well-recognized, distinct feature of gliomas to diffusely infiltrate brain tissue, rendering them impossible to be completely resected biologically or histopathologically. However, radiographically complete resections of gliomas are not simply the neurosurgeon’s goal: in recent years, several well-conceived and well-conducted studies have indicated that the extent of resection is one of the most important prognostic factors for patients with both high-grade and low-grade gliomas.2–6 To provide the neurosurgeon with information about the extent of resection intraoperatively, different techniques and modalities have been developed and assessed. MRI is the gold standard for the diagnostic and follow-up imaging of gliomas, and intraoperative MRI (iMRI) provides optimal image quality in a familiar way. Although there is ongoing interest in other modalities such as ultrasound or computed tomography (CT), both have significant drawbacks concerning image quality or radiation exposure. Consequently, the intraoperative resection control for tumors of glial origin represents the main indication for the use of iMRI.7 Several different iMRI systems have been developed and are available today. These systems can be categorized by the magnet’s field strength, by the magnet’s configuration, and by the way in which the patient is brought to the scanner or the scanner to the patient. In this chapter, we will review the experiences that others and we have had using low-field iMRI systems in glioma surgery. Peter Black from the Brigham and Women’s Hospital in Boston and General Electric Co. (General Electric Medical Systems, Waukesha, WI) pioneered iMRI with the development of the first iMRI scanner to be used in the neurosurgical operating room (OR) in the 1990s. For technical reasons, this device was equipped with two vertical superconductive magnets employing a magnetic field strength of 0.5 tesla. It required major modifications of OR infrastructure to meet the demands for low radiofrequency (RF) interference as well as the adaptation of surgical tools, instruments, microscopes, and so on, which all needed to be MRI-compatible. There was a 60 cm vertical gap between the magnets, in between which the patient’s head was placed. The surgeon also stood between the two magnets. Due to the shape and appearance of the system, the Signa SP was nicknamed the “double-donut” system.8,9 The main drawbacks of this system was the constrained working area of the surgeon, who has to stand in between the magnets, and the patient positioning restrictions (Fig. 11.1). Simultaneously, Siemens Corporation (SiemensAG, Erlangen, Germany) developed a C-shaped resistive MRI scanner with a static magnetic field with a strength of 0.2 tesla (Magnetom Open). In contrast to the Signa SP, which marked the center of the operating theater, the Magnetom Open was set up at one end of the OR, separated from the surgical area by RF shielding, thus allowing for the use of standard instrumentation during surgery. The patient had to be transferred to the magnet, which featured a 240-degree opening, allowing for safe placement of the anesthetized patient’s head into the scanner’s field of view.10,11 However, image acquisition necessitated transferring the patient from the operating site to the scanner, which was time-consuming. Fig. 11.1 Example of surgery with the GE Signa SP (0.5 tesla; General Electric Medical Systems, Waukesha, WI). The surgeon stands in between the magnets; surgery is performed within the magnetic field using special instruments. Although the AIRIS II MRI scanner (Hitachi Medical Corp., Tokyo, Japan), employing a magnetic field strength of 0.3 tesla, was developed primarily as a conventional diagnostic tool, it was also employed as an iMRI scanner.12,13 It is an open MRI unit with two horizontally oriented magnets with a vertical opening of 17 inches. Surgeries can be performed either in the adjacent OR, or with the patient positioned on the scanner’s table. The PoleStar (Medtronic Navigation, Inc., Louisville, CO) is one of the most frequently used low-field systems today with a static magnetic field strength of 0.12 (Model N-10) or 0.15 (Model N-20) tesla. It was developed to overcome the necessity of using special, MRI-compatible instruments and surgical devices, demanded for by other iMRI systems, while avoiding cumbersome patient transfer to the scanner. The PoleStar was designed as a mobile MRI unit with two vertical magnets spaced 25 or 27 cm apart, respectively. During surgery, conventional instruments can be used while the magnet is parked underneath the operating table (Fig. 11.2). The scanner is moved upward for intraoperative image aquisition.14,15 In other groups’ as well as our experience, there are no specific safety concerns in using low-field iMRI, and there are no risks on top of those related to cranial surgery in a conventional OR setting.10,12,16–18 There are no reports of patients being harmed by the use of low-field iMRI itself despite large numbers of patients being treated.19,20 On the contrary, iMRI even allows for the intraoperative detection of complications, such as hematomas.21,22 Further, low-field iMRI is not limited to adult patients only. Several groups have successfully performed surgeries with the help of iMRI in series of pediatric patients with comparable results to adult patients without complications pertaining to MRI.23,24 Although there is a variety of different magnet designs, field strengths, and investigational concepts, there are many similarities in the information obtained to guide and monitor neurosurgical procedures. All of the above-mentioned systems have been successfully used to monitor craniotomy and extent of resection in patients with gliomas.12,14,18,21,25–30 However, each has its own set of advantages and disadvantages, and as iMRI still evolves, no single system has gained universal use. Image quality in MRI is directly linked to the field strength of the magnet and to the homogeneity and stability of the static and gradient magnetic fields. Undoubtedly, high-field, 1.5 or even 3 tesla systems offer an excellent image quality that cannot be reached by low-field systems. They are, however, very expensive, and are yet limited to a small number of centers worldwide. In iMRI in general, there is a constant trade-off between signal-to-noise ratio, access to the patient, and usable field of view. The optimal design of a magnet with regard to homogeneity would be a complete sphere without opening, which is obviously impossible in both conventional “closed” and intraoperative “open” MRI systems.31 To overcome this problem and to achieve an acceptable signal-to-noise ratio in low-field open MRI systems, longer acquisition times are necessary. Although weaker magnets are usually less expensive and can allow near-real-time imaging, they suffer from poorer image resolution. High-field systems on the other hand are more expensive and permit only interruptive scanning. Fig. 11.2 Example of surgery with the PoleStar N-20 (0.15 Tesla; Medtronic Navigation, Inc., Louisville, CO): standard instruments can be used when the magnet is placed underneath the operating table. From an image-quality point of view, cylindrical superconductive systems bear significant advantages relative to static magnetic field strength and homogeneity.32 In the Signa SP scanner, the central segment of a cylindrical system was taken out, allowing for direct access to the patient in the scanner at the expense of a decreased field strength at the imaging isocenter compared with 1.5 tesla. Nonetheless, image quality is still almost comparable to high-field imaging and sufficient for the delineation of both contrast-enhancing and noncontrast-enhancing lesions such as high- and low-grade gliomas.21 In contrast to this system, the PoleStar MRI system offers more convenience for the surgeon with a larger working space, yet its field strength is even lower, and the magnet’s field of view is considerably smaller, requiring accurate patient positioning and rendering a slightly lower signal-to-noise ratio. Still, the PoleStar provides good quality visualization of contrast-enhancing tumors and fair quality for nonenhancing lesions (Figs. 11.3 and 11.4).16,33 In our experience, the image quality in high-grade gliomas is comparable in both systems, but image quality in low-grade gliomas is to some extent better when using the Signa SP.30 Contrast-enhancing gliomas are displayed best on T1-weighted imaging with application of contrast agent; usually higher doses of contrast agent are recommended for optimal lesion to white matter contrast.34 Several groups have experienced difficulties with visualizing the tumor margins on regular T2-weighted imaging in nonenhancing tumors with different low-field MRI units; fluid-attenuated inversion recovery (FLAIR) sequences were found to be superior in these cases.9,16,35 The introduction of image-guided frameless neuronavigation was an important step toward an enhanced visualization of the surgical field. However, although helpful in planning the initial surgical approach, the method of neuronavigation is of limited use during or at the end of tumor resection because of intraoperative anatomical alterations called “brain-shift,” caused by surgical retraction, cerebrospinal fluid (CSF) loss, edema, and tumor removal itself (Fig. 11.5).36 As a result, neuronavigation that is based solely on preoperative imaging data is not reliable in terms of determining residual tumor tissue intraoperatively.
Utilization of Low-Field Intraoperative MRI in Glioma Surgery: An Overview
Prevailing Low-Field iMRI Systems
Safety Considerations
Image Quality in Low-Field MRI
Indications for iMRI
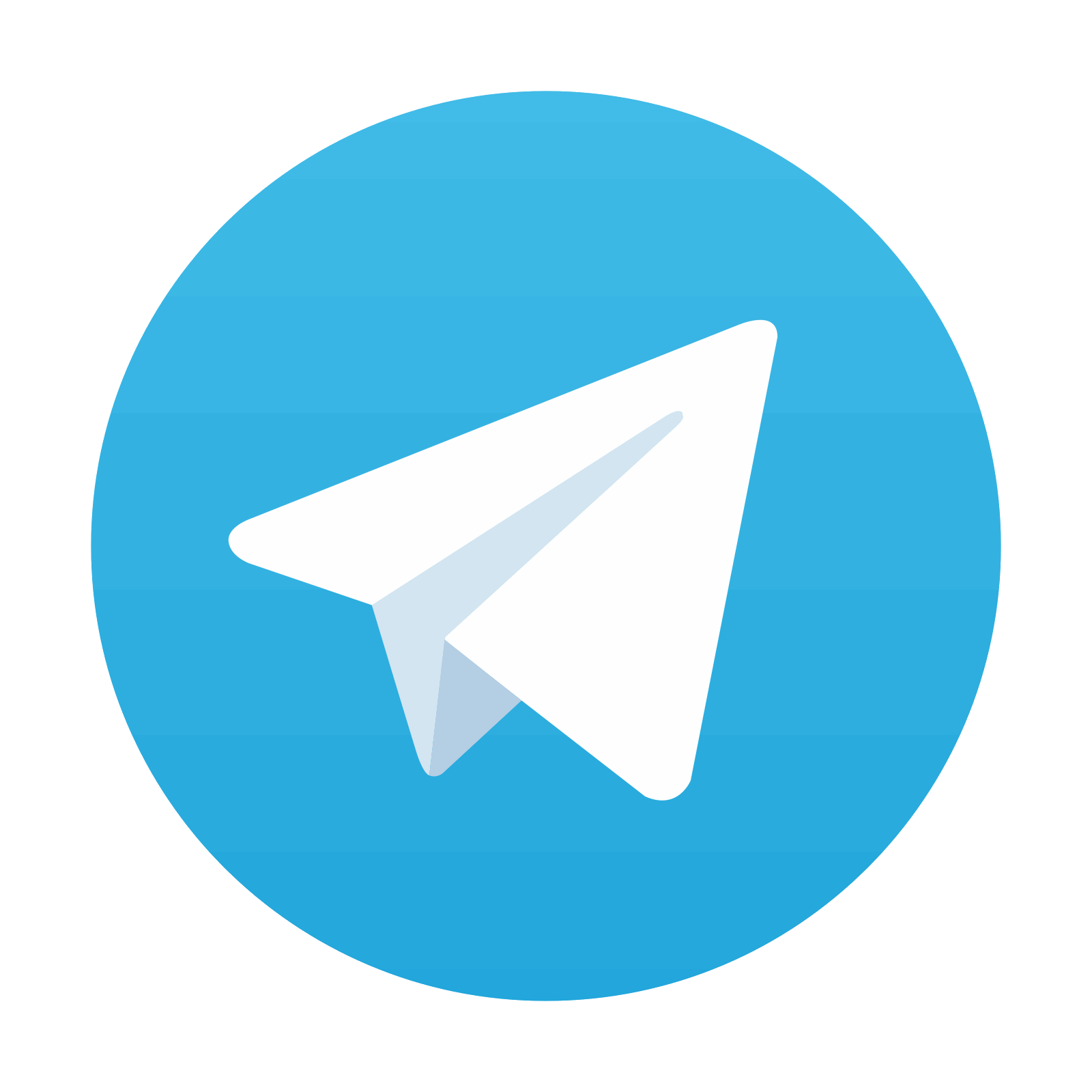
Stay updated, free articles. Join our Telegram channel

Full access? Get Clinical Tree
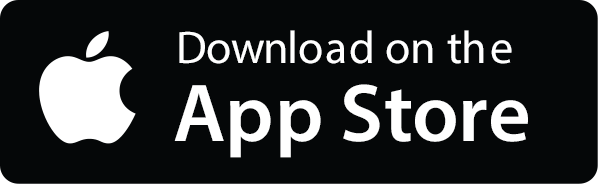
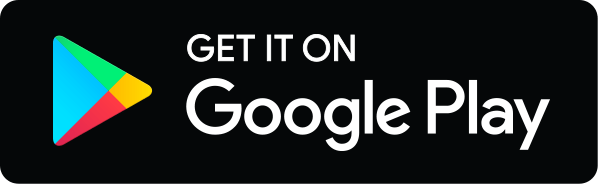