5 COMPUTER ANALYSIS OF INTRAVASCULAR ULTRASOUND IMAGES*
1Department of Electrical and Computer Engineering,
2Department of Internal Medicine, The University of Iowa, Iowa City, IA 52242, USA
3Thoraxcenter, Division of Cardiology, Erasmus University Rotterdam, The Netherlands
5.1. INTRODUCTION
While each of the existing methods for assessing coronary artery disease in vivo like coronary angiography, fluoroscopy, fiberoptic angioscopy, ultrafast computed tomography, magnetic resonance angiography, epicardial ultrasonography, and intravascular ultrasound has potential advantages,1–4 only coronary angiography has found widespread clinical use. Coronary angiography produces projection images or silhouettes of the vascular lumen from the absorption of X-rays by radiopaque dye injected into the coronary tree.5 The coronary wall and any atherosclerotic plaque that might be present are not directly visualized. Conclusions about the presence and significance of atherosclerosis are inferred from visual assessment of the reduction in vessel lumen diameter with respect to the lumen diameter of a nearby vessel segment that is presumed to be normal. The shortcomings of visual evaluation of lumen dimensions are widely recognized.6 Even when employed in conjunction with quantitative analysis,7,8 coronary angiography has several limitations. These include the inability to detect plaque in diffusely diseased vessels, inability to directly determine vessel wall and plaque morphology, inaccuracy in analyzing eccentric lesions, and insensitivity to changes in arterial morphology after catheter-based interventional therapy.
Intravascular ultrasound yields tomographic two-dimensional crosssectional images of vessel wall architecture and plaque morphology. From the images obtained by intravascular ultrasound, morphometric measurements of lumen and plaque can directly be performed.9,10 The principle underlying the measurements obtained from intravascular ultrasound images differs substantially from quantitative coronary angiography, which has traditionally been viewed as the “gold standard” in quantifying coronary artery dimensions. The main difference is that quantitative angiography does not permit direct measurement of plaque dimensions, but quantifies lumen diameter and obstruction based on the brightness and contours of the opacified silhouette of the coronary lumen, assuming a non-diseased reference segment.11–13 This assumption, however, is frequently false and particularly in diffusely diseased coronary arteries, the obstruction can be significantly underestimated. Intravascular ultrasound measures lesion significance independently of the reference segments.14 Post-mortem studies and early in vivo studies have shown that intravascular ultrasound yields important information about vessel morphology that is not obtained from angiography.
This chapter summarizes the use of intravascular ultrasound (IVUS) imaging in diagnostics and interventional treatment of coronary arteries and describes computer-aided approaches that help analyze IVUS images. It should be mentioned, however, that intravascular ultrasound is also used to image a variety of other vascular structures; iliac or femoral arteries being the most common example.
5.2. INTRAVASCULAR ULTRASOUND IMAGING
5.2.1. Intravascular Ultrasound Imaging Devices
With intravascular ultrasound, a high-frequency (20–50 MHz) ultrasound source rotates near the tip of a catheter inserted in the arterial lumen.15 Either the piezoelectric crystal generating the ultrasound beam or a mirror deflecting the ultrasound beam is rotated. Alternatively, a stationary multi-element crystal array encircling the catheter may be employed. In the former case, the ultrasound element or the mirror is rotated by use of a long flexible shaft driven by an external motor. The lengths of the shaft and arterial path tortuosity may cause non-uniform rotation creating imaging artifacts. In the latest prototypes, the external motor has been replaced by a micromotor in the catheter tip. In multi-element ultrasound arrays, no mechanical rotation is involved and the signal is processed and multiplexed using an integrated circuit in the catheter tip. Possibility to use a central guide wire with a full 360° field of view is the main advantage of this design. In addition to the single-cross-sectional ultrasound imaging devices, prototypes of forward-looking catheters and imaging ultrasound guidewires have been reported.15
Intravascular ultrasound cross-sectional images directly demonstrate arterial lumen, plaque, and wall morphology. Figure 1 shows examples of two intravascular ultrasound images of a cylindrical latex phantom.16,17 Intravascular ultrasound morphology, determined from operator-defined borders, agrees closely with quantitative histologic determination of lumen area and mural thickness18,19 and angiographic determination of lumen size.9,20,21
5.2.2. Coronary Wall Morphology Imaged by Intravascular Ultrasound
Atherosclerosis is characterized by an accumulation of plaque material in the arterial wall. Figure 2 shows in schematic form the cross-sectional anatomy of a diseased coronary artery. In adults, normal coronary vessel walls (upper portion of Figure 2) have a thin intimal layer bounded by the internal elastic lamina. The media, composed of smooth muscle cells and a reticular collagen network is located between the internal and external elastic laminae and is of nearly constant thickness, typically 0.1–0.3 mm.9,22–24 Outside the external elastic lamina is fibrous and fatty adventitia tissue. With progressive atherosclerosis (bottom portion of Figure 2), intimal cells proliferate, lipid and cholesterol accumulate, fibrosis occurs, and the wall thickens. As intimal plaque thickens, the media may thin and the internal lamina may be disrupted. Initially, eccentric plaque bulk increases the total wall thickness and locally distends the wall such that the lumen shape and diameter is maintained (“arterial wall remodeling”). As plaque continues to accumulate, the lumen is compromised, the plaque may become more concentric, and focal areas of calcification and extracellular lipid or cholesterol depositions (“lipid lake”) are seen. Because these morphologic changes can be visualized by intravascular ultrasound, the appearance of intravascular ultrasound images can be complex.
Figure 1. Intravascular ultrasound images of a cylindrical latex phantom. (left) Cross-section representing an undiseased artery. Strut artifact of the used intravascular ultrasound catheter can be seen as a noisy wedge in the 4- to 6-o’clock position. (right) Lesion cross-section. The latex wall borders and the borders between lumen and plaque are clearly visible, plaque is present in the 6- to 12-o’clock position. The strut artifact is seen in the 3- to 5-o’clock position.
Figure 2. Schematic cross-sectional anatomy of a diseased coronary vessel (not to scale).
Figure 3. Intravascular ultrasound image of a diseased coronary vessel. (top) Original image with 0.5 mm calibration marks showing circumferential plaque that thickens at the 10- to 2-o’clock position. Double-echo response from the internal and external elastic laminae with intervening sonolucent media is seen at the 12-o’clock position (arrow A), and also at 10-o’clock and 5-o’clock. The interface between lumen and plaque is depicted by arrow B and is visible at 9- to 6-o’clock. The catheter near-field artifact surrounds the catheter in the middle of the image and obscures the plaque–lumen border from 6- to 9-o’clock. Arrow C shows a near-field artifact. Two wide arrows point to regions of signal attenuation caused by fibrotic plaque that is highly reflectant. The dark sonolucent region from 10- to 2-o’clock located inside the plaque is due to echo dropout distal to the fibro-calcific plaque at the lumen–plaque interface. Arrow D shows the strut and guidewire artifact. The complex plaque morphology in this vessel was confirmed with light microscopy. (bottom) Positions of external elastic lamina, internal elastic lamina, and coronary lumen defined by an observer.
The interaction of ultrasound with coronary arterial structures gives rise to typical image patterns that may be used to identify arterial wall and intramural structures within the ultrasound image (Figure 3). Intravascular ultrasound images contain both large amplitude signals from specular reflectors at the interface between structures with differing acoustic impedances together with lower amplitude signals or diffusely scattered signals arising from regions of nearly uniform acoustic properties. The lumen is typically a dark echo-free region adjacent to the imaging catheter. The lumen-intima interface constitutes a large acoustic impedance mismatch and produces specular reflections.23 The internal and external elastic laminae enclose the sonolucent media and produce acoustic impedance mismatches. There is an ongoing discussion about what exactly causes the ultrasound echo responses. Additionally, the internal elastic lamina often partially disappears in diseased coronary vessels. The layers typically give rise to a double-echo pattern showing a circumferentially-oriented parallel bright-dark-bright echo pattern25 (arrow A in Figure 3a). The echo-dense responses are from the interfaces of the fibro-elastic internal and external elastic laminae and the homogeneous echo-free media layer. The fibrous adventitia further demarcates the outer wall with abrupt increases in echo-density which parallel the double-echo responses from the laminae and media. With coronary artery disease, the intima thickens and is identified as the echogenic region between the lumen interface and the sonolucent media (arrow B in Figure 3a). The ultrasonic appearance of atherosclerotic plaque depends on its composition.25 Fibromuscular lesions appear as echoes of intermediate intensity attached to the intima. Dense fibrous lesions appear as bright echoes. Calcium deposits also give rise to high intensity echoes. Lipid deposits within a lesion are hypoechoic. Analysis of intravascular ultrasound images can be complicated by focal or radial echo patterns which are generated by local changes in plaque density. For example, regions of substantially attenuated signal intensity (two wide arrows in Figure 3a) may be caused by highly echo-reflectant fibrous plaque or calcium deposits which produce acoustic shadowing.
The presence of a variety of artifacts can render the interpretation of intravascular images difficult. Blood speckle and near-field artifacts can be identified by observing the real-time video. As the catheter is pulled back, the artifacts persist while the wall morphology changes. A bright near-field “halo” adjacent to the black circle depicting the catheter’s position results from ultrasound reflections from the catheter itself (arrow C in Figure 3a). These artifacts and geometric distortion due to an eccentric or angulated catheter position within the vessel may prevent visualization of the double-echo pattern from the media–laminae interfaces. In second generation catheters, a strut or guidewire artifact across the imaging plane obscures (or distorts) mural details in portions of the arterial wall (arrow D in Figure 3a).
5.3. CLINICAL IMPORTANCE OF INTRAVASCULAR ULTRASOUND IMAGING
Intravascular ultrasound imaging yields morphologic information complementary to contrast angiography. There is substantial enthusiasm in the clinical community for using this emerging imaging method to overcome the shortcomings of angiography and for guiding catheter-based interventions.26–29 Intravascular ultrasound is capable of providing crosssectional images of the coronary arteries and visualizing the structural9,30–33 and functional pathology34,35 of the coronary artery lumen and vessel wall. Plaque eccentricity, lipids, and calcification can be determined.36 Mechanisms of plaque dilatation, recoil, and dissection after catheter-based intervention can be studied.29 Ongoing clinical trials have presented preliminary data showing that immediately after angioplasty, intravascular ultrasound determination of minimum lumen diameter and plaque area as a percentage of total arterial cross-sectional area are predictors of restenosis within 6 months after the interventional therapy.37
5.3.1. Measurements of Plaque Dimensions
Intravascular ultrasound enables the quantitative study of the extent of coronary atherosclerosis. The important landmark study of Glagov et al.38 demonstrated vessel wall remodeling — a compensatory enlargement of the vessel wall in response to progressive atherosclerosis, accommodating the increasing atherosclerotic burden up to 40% of the cross-sectional reference area. The results of Glagov’s study highlight the importance of direct visualization and measurement of plaque dimensions, provided by intravascular ultrasound. Comparing histology and angiograms in coronary arteries of postmortem human hearts, Stiel et al. confirmed that the compensatory enlargement of atherosclerotic coronary arteries results in a significant angiographic underestimation of the extent of atherosclerosis during early stages of coronary artery disease.39 In 1987, McPherson et al. demonstrated the unique capabilities of epicardial high-frequency ultrasound imaging, which has been a forerunner of intravascular ultrasound, in detecting coronary plaques in patients without angiographic evidence of atherosclerosis.40 Intravascular ultrasound is indeed able to detect atherosclerosis in angiographically normal vessels.41 St. Goar et al. studied the coronary arteries in cardiac transplant recipients using intravascular ultrasound. In the majority of patients who were studied one or more years after transplantation, an intimal thickening could be demonstrated that was not visible on the angiograms.32 During the early phases, atherosclerosis can frequently be found by intravascular ultrasound at the site of a focal vasospasm,35 which demonstrates the significant sensitivity of this technique.
Echocardiographic confirmation of the compensatory enlargement of coronary arteries during the progression of atherosclerosis was first performed intraoperatively, using high-frequency epicardial echocardiography.42 Thereafter the mechanism could be demonstrated by intravascular ultrasound in coronary30,43,44 and in femoral arteries.45 However, intravascular ultrasound offers not only an ideal way of evaluating the dimensions of atherosclerotic arteries at a point in time,28 but can also be used for serial studies, for instance in trials assessing the progression/regression of coronary atherosclerosis.46,47 Opposite to quantitative coronary angiography, intravascular ultrasound measures dimensions independently of the reference segment disease which is likely to be involved in the process of progression or regression.
5.3.2. Lesion Calcification
5.3.2.1. Detection of lesion calcification
Identification of calcific lesions is important. Calcium appears to stabilize a non-treated plaque and protect patients against unstable clinical syndromes.31 However, following directional coronary atherectomy, a higher incidence of complications and a smaller amount of retrievable material can be observed in the presence of diffuse subendothelial calcification48 (Figure 4). The angiographic assessment of 400 lesions, reported by Ellis et al., demonstrated an independent correlation of target lesion calcium with adverse outcome after directional coronary atherectomy.49 Mintz et al. reported that coherent calcific deposits in the target lesion were discovered in 75% of patients examined by intravascular ultrasound before or after various catheter-based interventions, however, calcium confirmed by ultrasound could be detected in less than 50% of the patients during angiography.50 Another study reported that target lesion calcification was detected by intravascular ultrasound in 83% whereas calcium was seen in only 14% of the lesions by angiography.51 These studies demonstrate that intravascular ultrasound is much more sensitive than angiography in detecting calcium. Only visualization of accumulated microcalcifications by intravascular ultrasound appears to be limited,52 reflecting the limited lateral resolution of the current intravascular ultrasound transducers.53
5.3.2.2. Relation of dissections to calcium deposits
Dissections are commonly accepted as an operative mechanism of balloon angioplasty.54,55 However, larger dissections can cause acute vessel closure, and when confirmed by intravascular ultrasound, dissections correlate with an increased risk of subsequent adverse events after angiographically successful coronary artery interventions.56 Fitzgerald et al. examined peripheral and coronary arteries of 41 patients in vivo after balloon angioplasty and demonstrated that in 87% of the cases, dissections were adjacent to a calcific portion of the vessel wall.23 Furthermore, an increased incidence, depth, and circumferential extension of dissections after balloon dilatation was found in calcific segments of atherosclerotic plaques.23,53,57 Results of these studies suggest that localized calcium deposits play a significant role in promoting the incidence of dissections.
Figure 4. Cross-sectional intracoronary ultrasound image showing a cut in the vessel wall (arrowhead) resulting from the application of a directional atherectomy device. The cut is located next to a deposit of superficial calcium, obscuring the underlying vessel wall in its echo shade. A larger part of the arterial wall is obscured by another arc of deep calcium.
5.3.3. Role of Intravascular Ultrasound Analysis in Pre-interventional Decision-making
Intravascular ultrasound findings frequently give rise to a modification of treatment strategy. Mintz et al. reported that intravascular ultrasound examination of 313 target lesions resulted in a change in therapy in 124 cases that corresponds to almost 40%.58 In particular, lesion calcification and eccentricity were reasons for changing or selecting specific interventional devices. Generally, eccentric lesions without evidence of superficial calcium were treated with directional atherectomy, while in superficially calcified plaques rotational atherectomy or eximer laser angioplasty were performed.58 Rotational atherectomy is particularly suitable for the treatment of superficially calcified, diffusely diseased coronary arteries, since the device ablates and pulverizes non-compliant plaque material by high-frequency rotation of a diamond-coated burr, resulting in a relatively circular and smooth shape of the coronary lumen.59 It may be used as a stand-alone procedure but is frequently used as a primary device followed by adjunctive balloon angioplasty or directional atherectomy.60 Thus, lesion eccentricity and the presence or absence of calcium and its superficial or deep location in the atherosclerotic plaque demonstrated by intravascular ultrasound imaging have major implications in the choice of an interventional device.
5.3.3.1. Balloon angioplasty
Serial intravascular ultrasound studies performed before and after balloon angioplasty have helped to identify the operative mechanisms of this device. In coronary and peripheral vessels in vitro and in vivo, vessel wall stretching, plaque rupture and dissection, and to some extent plaque compression were confirmed to reflect the principal operative mechanisms of balloon angioplasty.61–64 The acute lumen gain and possible complications of a procedure such as large dissections with threatening vessel closure can be studied by intravascular ultrasound. Dissections of the coronary plaque are commonly found after balloon angioplasty,28 but even complex dissections, frequently not detected by angiography, can be demonstrated by intravascular ultrasound.56,57,65,66 Recently the diagnosis of a pseudoaneurysm formation following balloon angioplasty was made using intravascular ultrasound.67 A new catheter combining ultrasound imaging and balloon angioplasty has been developed and preliminary experience with this device has demonstrated its feasibility and usefulness.68–70 The device can be utilized to obtain additional qualitative and quantitative information about vessel lumen and wall before and after an intervention. Intravascular imaging through the angioplasty balloon permits direct examination of the vessel wall morphology and furthermore acute recoil can be evaluated immediately. The combination of a diagnostic and a therapeutic device may reduce the time required for the exchange of catheters in separate ultrasound examinations and minimize the small risk of inducing or aggravating vessel wall injury during repeated insertions of the catheters. In coronary stenting this combined approach may facilitate stent implantation and expansion and reduce the risk of stent strut damage.71
Figure 5. Well deployed Wallstent. The brightness of the echo-reflective struts allows to distinguish between the stent and the artery wall. A circular stent configuration and a good apposition to the vessel wall are demonstrated.
5.3.3.2. Coronary stenting
In acute or chronic dissection, intravascular ultrasound imaging helps to distinguish between true and false lumen and thus increase the safety of the stenting procedure.72,73 The struts of the metal stents are echo-reflective and well visualized (Figure 5). After elective coronary stenting, Laskey et al. found substantial improvement of angiography-related parameters, but less favorable outcome resulted from the intravascular ultrasound examination. This emphasizes the benefit of routine ultrasound studies during the implantation of coronary stents.74 Furthermore, information concerning a significant disease of the reference segments can only be provided by intravascular ultrasound, and thus used to control the deployment and expansion of the stent.
The major criteria of the quality of stent deployment are considered to be lumen symmetry, stent apposition to the vessel wall and the absence of a residual lumen narrowing inside the stent. Detection of a moderate residual stenosis inside the stented coronary segment by angiography is difficult. Unfortunately, such an intra-stent stenosis carries a higher chance of turbulent blood flow which can result in the accumulation of thrombus inside the stent and finally increase the risk of stent thrombosis and restenosis. The optimization of stent deployment based on the utilization of imaging and morphometric measurements by intravascular ultrasound, however, virtually eliminates the risk of acute or subacute stent thrombosis.75 As a consequence, coronary stenting can be performed without anticoagulation.76 The large single-center experience of Colombo et al. with intravascular ultrasound guided stenting has demonstrated an evident inadequacy of angiographically guided stenting. In order to achieve improved stent apposition, higher balloon-to-artery ratios and higher balloon inflation pressures are advocated. Ongoing multicenter studies are required to establish whether this concept without ultrasound guidance is sufficient to achieve results comparable to the outcome after ultrasound guided stenting.77 A new mechanically rotated intravascular ultrasound guidewire78 that can be passed centrally through the lumen of the stent delivery balloon represents a concept with the inherent potential to minimize the time required for ultrasound imaging and the risk of strut damage. The ultrasound guidewire may increase the accuracy of stent placement and may be particularly beneficial in the implantation of multiple stents.
5.3.3.3. Directional coronary atherectomy
Directional coronary atherectomy is particularly efficient when it is applied to “soft”, eccentric plaques without superficial calcium in proximal or mid-coronary segments.49,58,79 Popma et al. studied 57 patients after directional atherectomy using intravascular ultrasound and found that the amount of residual plaque mass remained significantly higher in lesions with an arc of calcium larger than 90°.80 Intravascular ultrasound is clinically useful in guiding the device since it demonstrates plaque composition and provides more reliable information about plaque eccentricity29,48 in contrast to angiography. Ultrasound guidance facilitates the optimization of plaque removal (Figure 4) and minimizes the trauma to the non-diseased vessel wall, which appear to be important factors in the process of restenosis.81 A prototype device combining ultrasound imaging and directional atherectomy provides images from the aperture of the atherectomy catheter.82 This approach allows the adjustment of the orientation of the cutter, scanning the plaque portion before the excision, and thus improving the procedural result. Ultrasound imaging can be performed repeatedly when the housing of a conventional atherectome has to be emptied of accumulated samples. The ultrasound examination pre- and post-intervention provides additional information suggesting that the operative mechanism is mainly based on plaque removal but also to a certain extent on the vessel stretch.62,64 Suneja et al. found that “plaque compression” accounts for 50% of the enlargement of lumen area, however, this finding seems to reflect the problem of pre-/post-studies which consider only changes at the site of the minimal luminal area,83 a limitation which can only be overcome by quantification with three-dimensional ultrasound.84,85
5.4. AUTOMATED ANALYSIS OF INTRAVASCULAR ULTRASOUND IMAGES
To date, quantitative studies of coronary atherosclerosis have nearly always relied on manual measurements of arterial structure dimensions and manual tracing of lumen and wall borders. While a number of studies demonstrate that experienced observers can identify plaque and wall borders in intravascular ultrasound images that agree with histology and angiography,3,26 the utility of analysis approaches relying upon manual border identification is limited by the need for observers with substantial experience and by the tedious nature of manual tracing. Recently, a number of groups have begun to develop computer-based methods for identifying lumen and wall borders.17,50,86–88 The most experience has been gained with computer methods that are based on image intensity thresholding. These methods classify each image pixel as belonging to lumen or vessel wall and generally are unable to distinguish between plaque, intima, media, and adventitia. Very little data demonstrating the accuracy of such techniques have been reported.89 Given the commonly recognized limitations of thresholding approaches to segmentation, it is unlikely that such approaches will be widely adopted for quantitative analysis of lumen and wall morphology from intravascular ultrasound images.
Development of methods for automated identification of lumen and mural structures has been limited in the past by the relatively poor quality of intravascular ultrasound images. Even with the recent substantial improvements in imaging catheters and signal processing hardware, accurate and robust segmentation of intravascular ultrasound images presents a very challenging problem. Studies correlating in vitro and in vivo intravascular ultrasound measurements with histology and angiography suggest that experienced observers can identify important luminal and mural structures. These studies suggest that when combined with appropriate a priori knowledge or context, the information necessary to perform such identification is present in the image data.
5.4.1. Approaches to Single-frame IVUS Image Analysis
Currently, visual identification of arterial wall and plaque borders is most commonly used to assess disease severity from intravascular ultrasound images. Manually traced borders of vascular structures in intravascular ultrasound images are typically used to calculate lumen and plaque crosssectional areas to distinguish critical and non-critical lesions,28,90 assess and classify the morphologic effects of percutaneous coronary angioplasty,65 and compute arterial biomechanical properties.91,92 The ability of experienced observers to integrate information from real-time image sequences and to apply a priori knowledge of arterial morphology are crucial determinants of the accuracy of visual border detection. A number of in vitro and in vivo studies have demonstrated the accuracy of visual border detection in intravascular ultrasound images by comparison to quantitative histopathology3,19,22,25,65,93 and to coronary angiography.21 The intra- and inter-observer reproducibility of lumen area and wall thickness measurements with intravascular ultrasound are good with mean percent difference between repeated determinations by the same observer of 2 ± 9% for lumen cross-sectional areas and 3.6 ± 18% for wall thicknesses, and between measurements by two observers of 1.8 ± 15% for lumen areas and 12 ±15% for wall thicknesses.22 While observers can manually identify accurate borders in individual intravascular ultrasound images, quantitative analysis of three-dimensional or dynamic arterial morphology based on manual tracing is impractical.
Automated or semi-automated image segmentation methods using image intensity thresholding to distinguish among vessel lumen, wall, and surrounding tissue have been employed to identify vessel lumen and to produce three-dimensional renderings of vessel geometry.50,86,94 Vessel wall detected by these methods typically includes plaque, intima, media, and adventitia with no or limited ability to distinguish between them. Rosenfield and associates consider a voxel as part of the vessel wall if its intensity lies between minimum and maximum threshold values specified by an operator.87,94 Threshold selection plays a crucial role in the analysis. An interactively-defined region of interest bounded by an inner and outer circle is provided by the operator and used to enclose the arterial wall and exclude the catheter and the associated ring-down artifact. Measurements of lumen area obtained with this method have been validated in comparison to quantitative angiography in an atherosclerotic swine model.89 A similar approach was reported by Mintz and colleagues.50 To avoid image thresholding difficulties arising from the presence of artifacts inside the vessel lumen and outside the vessel wall, they manually define regions of interest to only include arterial structures between adventitia and intima. The limitations of thresholding using fixed thresholds as a segmentation method are widely recognized in the medical imaging community. Even very careful region of interest selection does not obviate these problems. Nevertheless, substantial improvements in the utility of threshold-based segmentation can be expected with improvements in intravascular image quality.
Much effort is currently being devoted to development of blood backscatter-based techniques to clean-up the noise from the imaged vessel lumen.15 It is a well-known observation that arterial walls tend to be represented by a more stable echo signal while the blood echo signal in the lumen is changing rapidly. The general idea of lumen image improvements is based on minimization of the blood scattering noise either by temporal averaging or sequential subtraction of image intensities in consecutive image frames,95 or averaging of the power spectrum of RF signals from scattered ultrasound.96–98 Texture properties of specular ultrasound signals are sometimes utilized to improve image segmentation in a variety of ultrasound image data.99–103
An intravascular ultrasound border detection approach which identifies wall and plaque borders that uses simulated annealing was reported by Herrington et al.88 In their approach, an operator interactively identifies an approximate border and a resampled image is formed using the approximate border to define a region of interest. An edge operator was applied to the resampled image and the closed boundary that represented the best compromise between identifying a smooth boundary and minimizing the sum of the inverted edge strengths along the boundary was determined. Using this procedure, borders between blood and plaque or between media and adventitia may be individually identified. Dhawale et al. use interactively-defined boundary points to guide a dynamic search for the border between media and adventitia and the border between blood and plaque.104
We have previously reported an approach using a heuristic graph search for identifying external and internal laminae and plaque borders.17 This method used an adaptive model-based approach in which the strength of the model varied around the vessel circumference as a function of local image quality. The method was validated in two distensible phantoms, each of which was imaged under several pressure conditions. Lumen cross-sectional areas correlated very well with distending pressure in both phantoms. Although this method yielded promising results and important insights in phantoms, it did not incorporate sufficient a priori information to perform well in more realistic imaging situations.
No fully automated method for detection of coronary wall and plaque borders in intravascular ultrasound images has been published to date. Intravascular ultrasound images are quite noisy and often contain catheter and other artifacts. Without contextual information from image frames adjacent in space and time, single-frame intravascular images are difficult to analyze even for the most experienced humans. To be successful, automated methods need to incorporate substantial a priori knowledge about ultrasound imaging physics and arterial anatomy. The ability to use information from temporally and spatially adjacent images could also prove advantageous.
5.4.2. Knowledge-based Approach to Coronary Wall and Plaque Border Detection
The following method for automated segmentation of intravascular ultrasound images developed at the University of Iowa uses global image information and heuristic graph searching5,105 to identify wall and plaque borders. A priori knowledge of coronary artery anatomy and ultrasound image characteristics were incorporated into the method for intravascular ultrasound border detection.
The method has two key characteristics. First, it uses a graph searching border detection approach that identifies a border that is optimal in a global sense. This approach is particularly advantageous in images where local edge gradients are insufficient to reliably identify border positions. Second, the method incorporates a priori information of a variety of types into the border detection process. In particular, to identify the position of the external elastic lamina we search not for a connected series of pixels with large edge gradients but for an expected double-echo edge pattern. This pattern does not rely on the frequently reported trilayer appearance of intravascular ultrasound images of muscular arteries. The trilayer appearance of diseased coronary vessels is thought to correspond with sequential layers of intima and/or plaque, media, and surrounding tissue (adventitia) and may in fact not always be present.23 We are instead exploiting the differences in acoustic impedance between the elastic laminae and the surrounding tissues.23,25
The method searches for edge triplets representing the leading and trailing edges of the external elastic lamina echo and the trailing edge of the internal elastic lamina echo. It has previously been suggested that the local gradients of trailing edges are less useful in locating borders because the trailing edge signal is highly gain-dependent.88
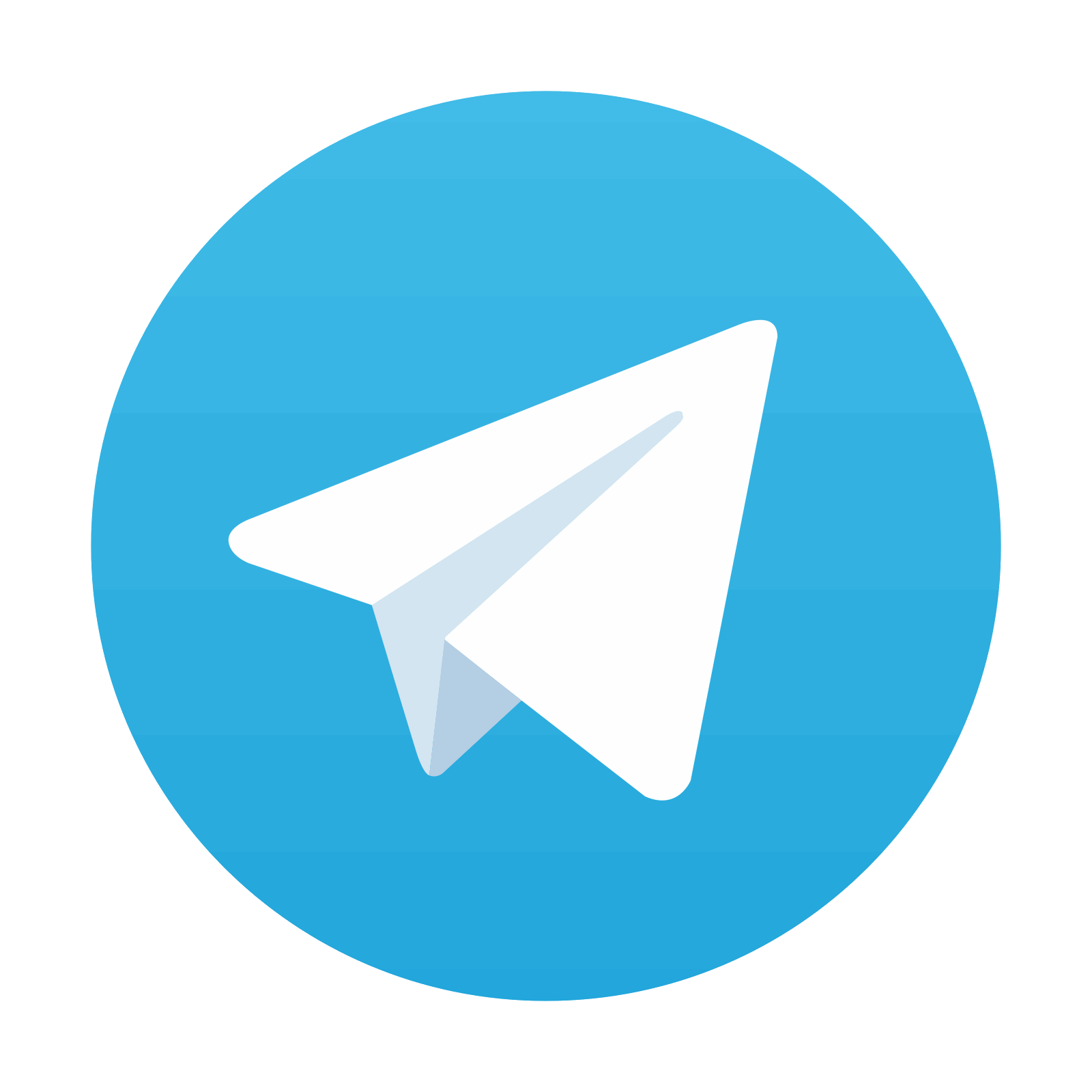
Stay updated, free articles. Join our Telegram channel

Full access? Get Clinical Tree
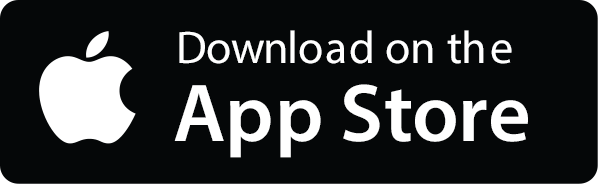
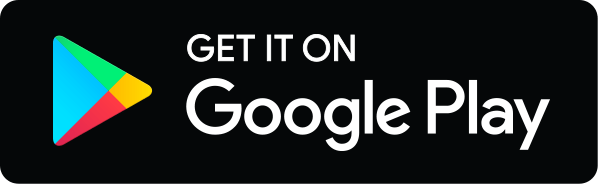