and Ashutosh Dash2
(1)
Nuclear Security and Isotope Division, Oak Ridge National Laboratory, OAK RIDGE, USA
(2)
Isotope Production and Applications Division, Bhabha Atomic Research Centre, Mumbai, India
15.1 Introduction
The introduction of balloon angioplasty represented the first effective strategy for arterial reflow of vessels obstructed by atheromatous plaque and offered reprieve for many patients who would have encountered morbidity and often mortality and who would not have been candidates for coronary bypass grafting (CABG). These complex atherosclerotic structures inhibit myocardial blood flow and thus significantly reduce the oxygenation required for contraction. They consist of lipids, foam cells, and, in later stages, calcium deposits, resulting from genetic, dietary, and other sequelae. With the vessel lumen held open by the stent framework, the plaque is compressed and reflow established. The first such procedure was performed in Zurich, Switzerland in 1997, and since that time coronary angioplasty and implantation of stent frameworks in patients with unstable angina have become a cornerstone of interventional cardiology. Although even approximate statistics are difficult to identify and although the aggressive use of these interventional procedures has declined, it is well established that several hundred thousand procedures are performed just in the USA annually. In spite of this pivotal advance for care of therapeutic intervention in these patients, however, restenosis of the coronary arteries is still a major issue when vessels are obstructed again in both native vessels treated only by angioplasty and stented arterial segments. In fact, it is common for many patients to have multiple stents deployed in the same vessels after the clinical signs of restenosis are again encountered, and there are even reports of patients in whom over 30 coronary stents have been implanted.
Arterial occlusions before and after angioplasty/stenting reflect two different pathologic processes. The restenosis structure is quite different in composition of the atherosclerotic plaques and consists of smooth muscle cell hyperplasia which is stimulated in response to the vessel damage resulting from the balloon inflation and subsequent stent placement. In terms of adequate flow requirements, this is only an academic difference, since the hyperplastic response still results in reduced blood flow to the heart muscle. The enormous health care costs required for intervention of these patients in conjunction with patient inconvenience are important factors which have stimulated the evaluation of the effectiveness of various methods for the inhibition of the subsequent restenosis which so often observed. Although the early intravenous application of radiation was the first widely acknowledged technology to significantly reduce hyperplasia after angioplasty, the issues associated with the use of radioisotopes in such an environments lead to the development of alternative nonradioactive chemical-based methods. The current standard of care for inhibition of coronary restenosis after angioplasty is thus the deployment of “drug-eluting” stents (DES), such as the sirolimus-eluting “Cypher” and the paclitaxel-eluting stents (“Tauxs” stent), which release growth substance inhibitors which inhibit or delay restenosis by inhibiting development of smooth muscle cell hyperplasia.
15.2 Radioisotopes for Intravascular Irradiation (IVRT) of Coronary Vessels
Although the focus of this book is on the use of unsealed radioactive materials for therapy, a brief review of the use of sealed radioactive brachytherapy sources (wires, radioactive stents, etc.) for restenosis therapy is included here as an introduction to the development of the unsealed radioactive source technologies. The first interventional strategy which was effective for restenosis therapy was the use of radioisotopes and related technologies to deliver a sufficient radiation dose to the vessel wall to sterilize those invading cells which release inflammatory substances (chemokines, etc.) which simulate the hyperplastic response as illustrated in Fig. 15.1.
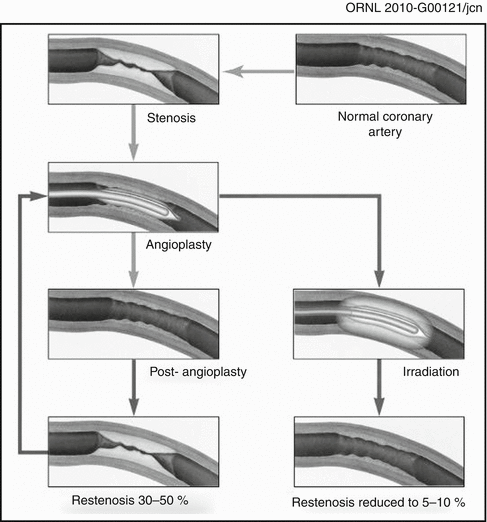
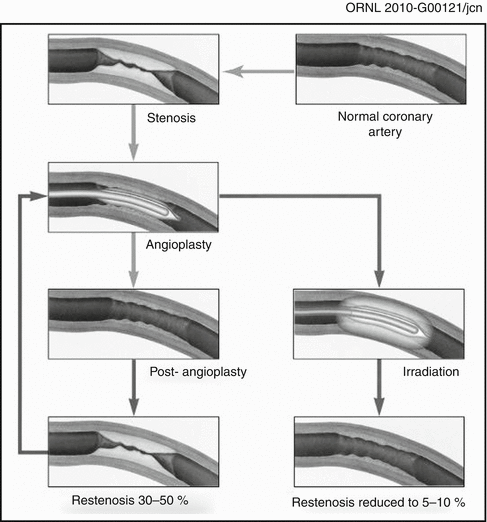
Fig. 15.1
Schematic illustrating the effect of post-PTA irradiation for inhibition of hyperplasia of coronary vessels. Post-balloon angioplasty of coronary stenosis often leads to restenosis resulting from smooth muscle cell hyperplasia resulting from the wound healing response
A large number of trials focused on the use of radioactive seeds or sources or even radioactive-coated balloons, and even the intraluminal advance of X-ray generating devices was evaluated in both preclinical studies and clinical trials. Many different radioisotopes decaying by photon, electron, positron, and X-ray emission and various source configurations had been evaluated in both animal models and human trials (Table 15.1). Interesting, even a series of short-lived positron-emitting radioisotopes (18F, 68Ga, 11C, 13N, 15O) had been evaluated for possible effectiveness for restenosis therapy in phantom experiments, and from these studies, 68Ga was selected as the most attractive positron-emitting candidate for this application (Stoll et al. 2001a, b). In addition, the use of other related technologies such as the use of catheter-based X-ray generating devices and photodynamic therapy such as the “PhotoPoint™” device (Waksman et al. 2008) had been evaluated also for vulnerable plaque. Once it had been established in clinical trials that the use of intravascular radiation could effectively inhibit, or at least significantly delay, restenosis, this field moved very quickly with the development, evaluation, and clinical introduction of many different technologies.
Table 15.1
Examples of radioactive solid source strategies which have been evaluated for the transluminal vessel wall therapy for inhibition of arterial restenosis
Radioisotope | T1/2: principal therapeutic radiation | Chemical form | Treatment focus | Reference |
---|---|---|---|---|
Solid sources, wires, seeds, etc.: sealed sources/radiation oncology | ||||
Beta/gamma-emitting sources | ||||
Cerium-144 | 284 days 0.318 MeV | Wire source | 30 patients: in-stent restenosis | Bonvini et al. (2003) |
Cobalt-55 | 17.53 h 17.5 h | 10 patients | Cervinka et al. (2004) | |
Holmium-166 | 26.8 h 1.854 MeV | Coated balloon | Porcine restenosis study | |
Iridium-192 | 74.02 days 316 keV | Wire sources Cordis/best | ||
Phosphoru-32 | 14.29 days 1.71 MeV | Coated balloon/catheter Stent: Guidant Wire | Rabbit ileal model 32 patients 332 patients | Herlein et al. (2000) |
Rhenium-186 | 90.64 h 1.076 MeV | Stents | ||
Rhenium-188 | 16.9 h 2.12 MeV βmax | Coated balloon Self-expanding stent | Sheep model Labeling/stability | Nowak et al. (2001) |
Strontium-90 /Yttrium-90 | 28.6 years 2.283 MeV (from 90Y) | Ribbon, coil: Novoste | ||
Tungsten-188 | 69 days 2.12 MeV (from 188Re) | Wire coil “WRIST” study | 30 patients: in-stent restenosis | Dilcher et al. (2005) |
Yttrium-90 | 64.1 h 2.283 MeV | Solid source with afterloader | 23 swine | Waksman et al. 2000 |
Xenon-133 | 5.245 days 0.346 MeV | Gas-filled balloon | Porcine model |
15.2.1 Solid Radioactive Sources for Vessel Irradiation
The strategies using sealed radioactive sources, which include various radioactive ribbon coils and seeds, as well as ion-implanted stents and radiation-generating devices, are used under the domain of radiation oncology in conjunction with interventional radiology. The first such radioisotope studies reported—which are discussed in more detail in the next section—for the prevention of restenosis after percutaneous transluminal angioplasty (PTA) used radioactive 192Ir sources for treatment of peripheral vessels (Liermann et al. 1994). One early patent (GE Healthcare) covered the use of low-energy X-ray emitting radioisotopes, such as 159Dy, 145Sm, 169Yb, 125I, and 103Cd (Lewis et al 2006). The radioactive sources are advanced either manually or using HDR afterloader devices. Because under-irradiation stimulates the hyperplastic response, irradiation of the stenoses ends is important, and the overlap of the radioactive source with these sources (5–10 mm) is accomplished to preclude the edge, or the so-called candy wrapper effect, where restenosis occurs at the proximal and distal edges which have not been adequately irradiated (i.e., under-irradiated with insufficient radiation dose), which at least to some extent precludes the effectiveness of the intervention.
15.2.2 Dosimetry of Vessel Wall Irradiation Is an Important Issue
Dosimetry also plays a pivotal role for this therapy since the appropriate radiation dose must be delivered. While over irradiation can cause unintended damage to vessels and surrounding tissue, under-irradiation can actually stimulate the hyperplastic response. Radiation physicists and dosimetrists have thus represented key members of the therapy teams. In addition, dose rate is a key issue, more so for therapy of the coronary vessels, since symptom limited time constraints on vessel occlusion are an important issue. Radioactive catheter-based beta-emitting sources posed serious challenges since the radioactive source must be centered in the lumen. Centering of the source is required because of the rapid dose falloff or decrease of radiation dose with radial distance of the emitted high-energy beta-particles. For this reason, some sophisticated and complex centering strategies were developed, such as a spiral balloon catheter and a CO2-based centering catheter (Pokrajac et al. 2009). However, liquid-filled balloons offer a convenient self-centering capability requiring no special centering strategy, and routinely available angioplasty balloons can be used for these studies. In this case when liquid-filled balloons are used as the therapeutic intervention, deflation to allow reflow is a common procedure to allow maintenance of adequate oxygenation, and repeated cycling of deflation and re-pressurization/refilling the balloon have been used for delivery of the appropriate prescribed radiation dose to the vessel wall. Although there is some controversy, the target area is the adventitia, and the prescribed dose values are within 18–35 Gy. Dose delivery is of course dependent on source “dwell time” (residence time during occlusion) and dose activity. When occlusive radioactive sources are used, as described later for liquid-filled balloons, delivery of the total prescribed radiation dose is often incremental, by removal of the radioactive source and thus permitting reflow during symptom limited periods, with repetitive irradiations until the total prescribed dose has been delivered.
For the coronary vessels, early studies also demonstrated that the use of 192Ir and 90Y seed/wire-based solid sources also worked well for inhibition of hyperplastic cellular response to damage incurred from coronary balloon angioplasty. These strategies were based on localized catheter-based delivery of high beta and gamma radiation (Waksman et al. 2001). Use of 188W/188Re wire sources for inhibition of coronary restenosis was also evaluated as an interesting candidate for restenosis therapy (Schaart et al. 2002). The 188W decays in situ to the high beta-energy emitting 188Re daughter (see Chap. 7), available from decay of the long-lived reactor-produced 188W parent (T1/2 69 days) (Chap. 5). The use 188Re as a liquid source separated from 188W is described later as a key example of use of radioactive liquid-filled balloons (Sect. 15.2.3).
However, because of the issue of unsymmetrical dose delivery to the vessel wall because of difficulty in centering such sources within the lumen, other self-centering strategies were evaluated which included the use of 32P-coated angioplasty balloons. However, because the exact balloon dimensions required for dilatation are not defined until angioplasty is conducted, maintenance of a spectrum of 32P-coated balloons in advance was a serious disadvantage. The potential use of intracoronary 188Re-labeled stents had also been evaluated (Dinkelborg et al. 2000) and using chelating microfilms (Zamora et al. 2000), but no further reports using these various technique have been published because of the cumbersome requirement for in-house radiolabeling after stent selection following PCTA and the challenges to accurately adjust the activity prescription for optimal radiation dose delivery. Of relevance to the present discussion is the use of radioactive liquid-filled balloons for restenosis therapy, since these substances are prepared and handled in the nuclear medicine facilities and represent unsealed sources. Generally, following placement of the therapy balloon, the radioactive solution is filled by a nuclear medicine physician. Table 15.2 summarizes information on several key liquid-filled systems which have been evaluated for therapy of the coronary arteries following balloon angioplasty.
Table 15.2
Examples of developmental studies with radioactive liquid-filled balloon strategies which have been evaluated for the transluminal vessel wall therapy for inhibition of arterial restenosis used in nuclear medicine/interventional cardiology
Radioisotope | T1/2: principal therapeutic radiation | Chemical form | Treatment focus | Reference |
---|---|---|---|---|
Liquid-filled balloons: unsealed sources/nuclear medicine | ||||
Gallium-68 | 68 min Positron | GaCl3 | Coronaries Animals | |
Holmium-166 | 26.8 h Beta | DTPA Ethylene dicysteine complex (EC) | Rat excretion | Chakroborthy et al. (2001) |
Phosphorus-32 | 14.3 d Beta, | Sodium phosphate/NaCl | Animal urinary secretion Rabbits Rabbits/dosimetry Rabbits/in-stent | Wilczek et al. (2002) |
Rhenium-186 | 90.6 h Beta, | Perrhenate | Dosimetry | McGoron et al. 1999 |
Rhenium-188 | 16.9 h Beta, | EC complex Perrhenate Perr/MAG3?DTPA | Excretion study Gel dosimeter Dosimetry Rats/excretion | |
Samarium-153 | 46.7 h Beta, 0.704 MeV | Liquid | Rabbits Histopathology | Moura et al. (2001) |
15.2.3 Radioactive Liquid-Filled Balloons for Vessel Wall Irradiation
Because of the technical issues associated with optimal source centering, the negative ramifications of rapid radiation dose decrease with radial distance and the effects of overdosing of normal tissue and underdosing of target cells, the development of inherently self-centering liquid-filled balloons was recommended (Knapp et al. 1997a, b, 1998b; 1999a, b, 2001; Weinberger et al. 1999; Weinberger and Knapp 1999) which is initially evaluated using 188Re and later with other candidate (Table 15.2).
The use of 188Re-liquid-filled balloons was envisioned as the most promising approach for uniform vessel wall irradiation, because of in-house availability from the 188W/188Re generator (see Chap. 7), rapid urinary excretion of released chemical species via the urinary bladder, excellent radionuclide properties (half-life, beta radiation, etc.), and optimal radiation dose delivery of liquid-filled balloons. For these reasons, 188Re rapidly gained broad interest and progressed to a large number of clinical trials which demonstrated the simplicity and effectiveness of this technique (Table 15.3). Most reported clinical coronary restenosis therapy studies with liquid-filled balloons have used 188Re, and this technique allows uniform vessel wall irradiation and represents use of an “unsealed radioactive source,” which is thus in the nuclear medicine domain, and the studies are conducted in conjunction with interventional cardiologists (Knapp et al. 1997a, b, 1998b, 1999a, b, 2001; Weinberger and Knapp 1999; 2002). The strategy for use of intra-vessel radioactive sources to inhibit the hyperplastic response from vessel damage from Percutaneous Coronary Transluminal Angioplasty (PCTA) is illustrated in Fig. 15.2 and is based on the delivery of sufficient radiation (i.e., 25–30 Gy to 0.5 mm) to inhibit the release of substances which would stimulate hyperplasia as a wound healing response to the vessel damage. Use of radioactive liquid-filled balloons is a special approach designed to minimize inhomogeneous vessel irradiation. Figure 15.3 illustrates the effective results of the first animal study using 188Re-liquid-filled balloons.
