Chapter 25 Recognizing Some Common Causes of Intracranial Pathology


TABLE 25-1 IMAGING STUDIES OF THE BRAIN FOR SELECTED ABNORMALITIES
Abnormality | Study of First Choice | Other Studies |
---|---|---|
Acute stroke | Diffusion-weighted MR imaging for acute or small strokes, if available | Noncontrast CT can differentiate hemorrhagic from ischemic infarct |
Headache, acute and severe | Noncontrast CT to detect subarachnoid hemorrhage | MR-angiography (MRA) or CT-angiography (CTA) if subarachnoid hemorrhage is found |
Headaches, chronic | MRI without and with contrast | CT without and with contrast can be substituted |
Seizures | MRI without and with contrast | CT without and with contrast can be substituted if MRI not available |
Blood | Noncontrast CT | Ultrasound for infants |
Head trauma | Nonenhanced CT is readily available and the study of first choice in head trauma | MRI is better at detecting diffuse axonal injury but requires more time and is not always available |
Extracranial carotid disease | Doppler ultrasonography | MRA excellent study |
Hydrocephalus | MRI as initial study | CT for follow-up |
Vertigo and dizziness | Contrast-enhanced MRI | MRA if needed |
Masses | Contrast-enhanced MRI | Contrast-enhanced CT if MRI not available |
Change in mental status | MRI without or with contrast | CT without contrast is equivalent |
Normal Anatomy









On an unenhanced CT scan of the brain, anything that appears “white” will generally either be bone (calcium) density or blood in the absence of a metallic foreign body (Table 25-2).

Hypodense (Dark) (AKA Hypointense) | Isodense | Hyperdense (Bright) (AKA Hyperintense) |
---|---|---|
Fat (not usually present in the head) Air (e.g., sinuses) Water (e.g., CSF) | Normal brain Some forms of protein (e.g., subacute subdural hematomas) | Metal (e.g., aneurysm clips or bullets) Iodine (after contrast administration) Calcium Hemorrhage (high protein) |
Mri and the Brain


Head Trauma




Skull Fractures



• Linear skull fractures are the most common and have little importance other than for the intracranial abnormalities that may have occurred at the time of the fracture, such as an epidural hematoma. Fractures of the cranial vault are most likely to occur in the temporal and parietal bones (see Fig. 25-5B).
• Depressed skull fractures are more likely to be associated with underlying brain injury. They result from a high-energy blow to a small area of the skull (e.g., from a baseball bat), most often in the frontoparietal region and are usually comminuted. They may require surgical elevation of the depressed fragment when the fragment lies deeper than the inner table adjacent to the fracture (Fig. 25-6A).
Basilar skull fractures are the most serious and consist of a linear fracture at the base of the skull. They can be associated with tears in the dura mater with subsequent CSF leak, which can lead to CSF rhinorrhea and otorrhea. They can be suspected if there is air seen in the brain (traumatic pneumocephalus), fluid in the mastoid air cells, or an air-fluid level in the sphenoid sinus (Fig. 25-6B).
Facial Fractures



• Orbital emphysema. Air in the orbit from communication with one of the adjacent air-containing sinuses, either the ethmoid or maxillary sinus.
• Entrapment of fat and/or extraocular muscle, which projects downward as a soft tissue mass into the top of the maxillary sinus.

Intracranial Hemorrhage
Epidural Hematoma (Extradural Hematoma)


Layer | Comments |
---|---|
Dura mater | Composed of two layers, an outer periosteal layer which cannot be separated from the skull and an inner meningeal layer; the inner meningeal layer enfolds to form the tentorium and falx. |
Arachnoid | The avascular middle layer, it is separated from the dura by a potential space known as the subdural space. |
Pia mater | Closely applied to the brain and spinal cord, the pia mater carries blood vessels that supply both; separating the arachnoid from the pia is the subarachnoid space; together the pia and arachnoid are called the leptomeninges. |

• They appear as a high density, extraaxial, biconvex lens-shaped mass most often found in the temporoparietal region of the brain (Fig. 25-8).
• Since the dura is normally fused to the calvarium at the margins of the sutures, it is impossible for an epidural hematoma to cross suture lines (subdural hematomas can cross sutures).
Subdural Hematoma (SDH)



• On CT, acute subdural hematomas are crescent-shaped, extracerebral bands of high attenuation that may cross suture lines and enter the interhemispheric fissure. They do not cross the midline.
• Typically, a SDH is concave inward towards the brain (epidural hematomas are convex inward) (Fig. 25-9A).
• As time passes and they become subacute, or if the subdural blood is mixed with lower attenuating CSF, they may appear isointense (isodense) to the remainder of brain, in which case you should look for compressed or absent sulci or sulci displaced away from the inner table as signs of SDH (Fig. 25-9B).
Intracerebral Hematoma (Intracerebral Hemorrhage)


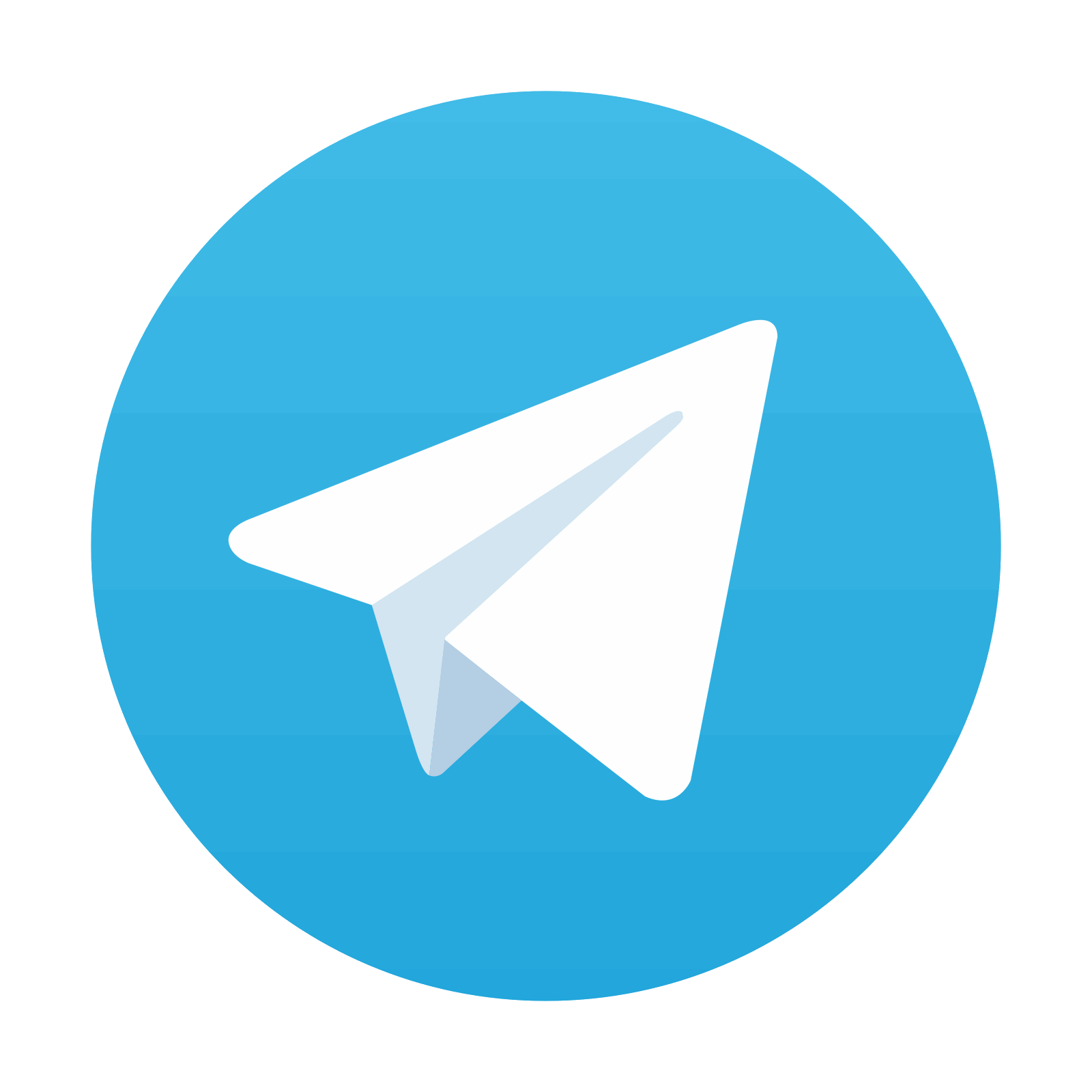
Stay updated, free articles. Join our Telegram channel

Full access? Get Clinical Tree
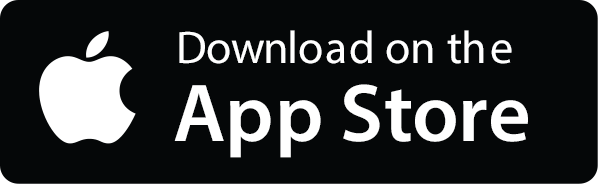
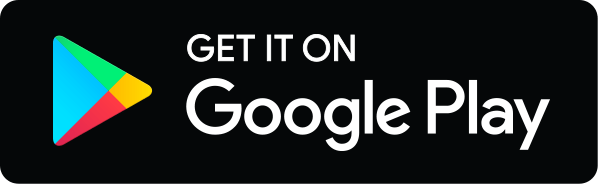