Abstract
The first chapter provides a general overview of the clinical applications and diagnostic utility of breast MRI and the need for specific individual technical protocols for both screening and diagnostic indications. The shortcomings of mammographic screening, particularly for those women at high risk or with dense breasts at mammography, have led to increased use of an abbreviated MRI sequence for breast screening. MRI standards, protocols and practice guidelines for screening are reviewed and discussed. MRI is a functional imaging technique and there is growing interest in establishing functional breast imaging methods using dynamic contrast-enhanced MRI (DCE-MRI) with kinetic analyses for classification of tumor biology and correlation with genomic markers. MRI is well suited for this type of analysis, identifying features that may reflect underlying malignant potential, providing an imaging biomarker that could play a key role in the development of precision medical therapy and personalized cancer care.
The diagnostic applications of breast MRI require a standard full protocol and may need additional advanced sequences according to the clinical indication for each individual patient. Indications for diagnostic MRI include assessment of disease extent at the time of initial cancer diagnosis, postoperative evaluation of residual disease following cancer excision, identification of recurrent tumor and monitoring of women undergoing cancer treatment with neoadjuvant chemotherapy. Other applications discussed in this chapter include problem-solving for difficult clinical or imaging findings, nipple discharge, implant assessment and use of MR-Guided biopsy.
1 Breast MRI: Overview
1.1 Introduction
Breast imaging has played an important role in the rapid development and change in breast cancer management over the past decades, not only by the introduction of new technical tools to aid in cancer detection and improve diagnosis, but also by the use of these tools to provide diagnostic information necessary to guide therapy. Over past years, the role of the radiologist has greatly changed; supervision of breast cancer screening programs using multimodal techniques and increased participation in multidisciplinary conferences have resulted in improved care for women diagnosed with breast cancer. When an abnormality is detected at screening, or when a patient is referred with clinical symptoms, the radiologist performs a diagnostic evaluation, discusses the pertinent findings with the patient and her family, and is usually the first physician to decide if a biopsy is necessary based on imaging findings. The whole gamut of interventional procedures, using stereotactic, ultrasound, or magnetic resonance imaging (MRI) guidance, provide accurate and timely diagnoses of nonpalpable image–detected lesions, with the additional advantage of limited patient morbidity when compared to surgical excision. When a malignant or uncertain finding is diagnosed, the radiologist will communicate the biopsy results with the patient and, in many instances, guide her to treating physicians for further management. New imaging techniques have benefitted patients, not only by allowing detection of an increased number of small cancers, but also by providing important diagnostic information essential for treatment, the goal being to provide optimal treatment for each individual cancer patient.
Mammography has been the tried-and-true breast imaging method for many decades, providing over 80% sensitivity for breast cancer detection in postmenopausal women 1 and even greater sensitivity in the symptomatic population, when combined with breast ultrasound. It would be logical therefore to ask why another imaging modality such as breast MRI is needed. It is well recognized that the sensitivity of mammography is substantially lower in the dense breast, less than 50% even when full-field digital mammography (FFDM) is used, as shown in the Digital Mammographic Imaging Screening Trial (DMIST). 2 Mammography suffers from inherent limitation of image contrast; many suspect lesions are therefore indeterminate and require further imaging evaluation and biopsy. Other important shortcomings of mammographic screening include recognized observer limitations as well as a propensity for detection of lesions with less aggressive histology, which may result in underdiagnosis of biologically aggressive disease. The failure to detect some high-grade breast cancers with mammography is driven largely by the degree of breast tissue density, but also by the very nature of rapidly growing, biologically relevant cancers that exhibit imaging features that are indistinguishable from normal breast tissue. Failure to diagnose these cancers at mammographic screening will result in progression of disease and diagnosis of advanced-stage interval cancers. Breast ultrasound is now commonly used for screening, and although this technique will identify some additional mammographically occult cancers, identification of ductal carcinoma in situ (DCIS) is challenging, and ultrasound suffers from low specificity in the screening setting. 3 , 4 Breast cancer continues to represent a major cause of cancer death in women; therefore, continued search for improved breast cancer screening methods is needed.
Against this background of mammography and ultrasound imaging, breast MR technology has improved steadily over the last three decades in large part due to research collaboration between radiologists, technologists, physicists, and industry scientists. Continued focus on research and educational efforts aimed toward improving diagnosis and patient care have resulted in increased use of breast MRI as a valued clinical resource for women’s health care. The main driver for the increasing adoption of breast MRI in clinical practice is its extremely high sensitivity, approaching 100% for cancer detection. Multiple studies have shown that standard dynamic contrast-enhanced MRI (DCE-MRI) for breast imaging achieves the highest sensitivity of any imaging modality, identifying cancer regardless of radiographic breast density, stage (DCIS or invasive), tumor type, or postsurgical changes. 5 , 6 , 7 , 8 Criticisms of low specificity in the early years of breast MRI might be expected with such a sensitive modality, but with current techniques and protocols, the specificity and positive predictive value (PPV) for malignancy can exceed that of breast ultrasound and mammography. Recognition of the salient MRI features of benign and malignant disease, and the specific morphologic and kinetic characteristics associated with various malignant subtypes, allows radiologists to provide important diagnostic information that can guide therapy. DCE-MRI protocols at both 1.5 and 3.0 Tesla provide excellent spatial resolution and accurate analysis of lesion morphology. Dedicated software providing semiquantitative analysis of lesion kinetics and display of its internal enhancement characteristics can improve diagnostic specificity. Measures of the initial rise and delayed phase of contrast enhancement within lesions are displayed as standard signal-intensity-versus-time-intensity curves (TICs). These computer-aided, semiquantitative, kinetic analysis tools have the advantage of being relatively simple to implement into routine practice and are widely used in the United States.
1.2 Breast MRI as a Biomarker
Traditionally, breast cancer treatment was determined by two major factors: (1) tumor histology, assessed by classifications based on grade and morphology such as ductal, lobular, mucinous tubular etc., and (2) the TNM staging method, based on cancer size, nodal status, and presence or absence of distant metastases. During the last 10 years or so, molecular subtyping of breast cancer has assumed a more important role in treatment planning and cancer classification has moved beyond the basic histologic assessment of prior years, to encompass treatment stratification based on tumor biology and gene expression profiles. There is a growing interest in establishing functional breast imaging methods, using DCE-MRI and its kinetic and morphologic analyses, for classification of tumor biology and correlation with genomic markers. MRI is a functional imaging technique and is well suited for this type of analysis. If MRI is to fulfill this role, the semiquantitative MRI methods used in current practice that document change in signal intensity over time will not be sufficient, and protocols that provide quantitative measurements of concentration of contrast media in tissues will be needed. Quantitative kinetic analysis depends on intrinsic lesion characteristics, acquisition parameters, and concentration, all of which play a role in signal enhancement. This type of analysis is necessary if we want to move beyond the binary choice, “is the lesion in question benign or malignant?” to ask whether a detected tumor has features that may reflect underlying biology and play a key role in precision medicine and personalized cancer care.
There is thus a need for further development of quantitative MRI biomarkers for prediction and prognosis. The goal of an imaging biomarker is to improve risk stratification, provide the right treatment for the right patient at the right time, reduce the variability in interpretation, and avoid trial and error in treatment. Quantitative imaging methods, however, face a key challenge because of the current lack of image standardization. Further development of acquisition standards that are reproducible and measures of signal analysis that are quantitative and widely available are required. Why are imaging biomarkers important? MRI is able to sample the entire cancer, and quantitative measures display tumor heterogeneity, providing serial noninvasive image data that are complementary to in vitro assay. Clinical trials that monitor drug action during treatment rely on quantitative standardized MRI results. The topic of image standardization and quantitative measures of enhancement will be discussed in detail in Chapter 12.
1.3 Clinical Utilization of Breast MRI in the United States
Overall use of breast MRI has increased over the past decade with 445,434 contrast-enhanced breast MR procedures performed in the United States in 2014. Although the number of breast imaging facilities declined by nearly 7% from 2002 to 2011, facility accreditation has increased. A modest expansion of breast MRI studies is expected in the next few years, driven partly by an increasing number of new breast density notification laws that have resulted in additional supplemental screening procedures for women with dense breasts. These laws have created a growing demand for new technologies that improve diagnostic accuracy. 9
An observational study reported in 2014 collected MRI and mammography data from 8,931 breast MRI examinations in women aged 18 to 79 years, during a time period of 2005 to 2009. 10 Data were acquired from five national Breast Cancer Surveillance Consortium registries. The overall rate of breast MRI nearly tripled from 2005 to 2009 from 4.2 to 11.5 examinations per 1,000 women, with the most rapid increase from 2005 to 2007 (p = 0.02). The list of MR studies by indication for the examination showed that the most common clinical indication was diagnostic evaluation (40.3%), followed by screening (31.7%). Women who underwent screening breast MRI, when compared with women who received mammography alone, were more likely to be younger than 50 years, white non-Hispanic, and nulliparous. Women in the MRI screening group were more likely to have a personal history of breast cancer, a family history of breast cancer, and extremely dense breast tissue (all p < 0.001). The proportion of women screened using breast MRI at high lifetime risk for breast cancer (> 20%) increased during the study period from 9% in 2005 to 29% in 2009. The authors concluded that the use of breast MRI for screening in high-risk women is increasing and that there is a need to improve appropriate use, by including more women at high risk who may benefit from screening breast MRI.
Another paper looked at overall rates of breast MRI use, by clinical indication and average annual percent changes, in a retrospective cohort study at a not-for-profit health plan and multispecialty group medical practice in New England. 11 The study enrolled 10,518 women aged 20 years and older in the health plan for at least 1 year. Each woman had at least one breast MRI between January 1, 2000, and December 31, 2011, and breast MRI counts were obtained from claims data. The results showed that breast MRI use increased more than 20-fold from 6.5/1, 000 women in 2000 to 130.7/10,000 in 2009, and use then declined and stabilized to 104.8/10,000 by 2011. A published commentary on these two studies by Hwang and Bedrosian noted that in the Wernli study, 10 fewer than 5% of the women at greater than 20% risk were actually screened, indicating that screening with MRI was overused in women who did not meet screening guidelines, while underused in those who could benefit the most. 12
A study of diagnostic MRI, recently reported among 10,766 older women, compared the frequency and sequencing of breast imaging and biopsy use for the diagnostic and preoperative work-up of women with newly diagnosed breast cancer. SEER-Medicare data from 2004 to 2010 were used and results indicated that 20% of women underwent MRI in the diagnostic/preoperative period, 60% received a mammogram and ultrasound, and 20% a mammogram alone. MRI use increased across study years, tripling from 2005 to 2009 (9–29%). Interestingly, among women receiving preoperative MRI, 26% underwent a subsequent biopsy compared with 51% receiving a subsequent biopsy in the subgroup without MRI. 13
1.4 Practice Guidelines
The American College of Radiology (ACR) Practice guidelines recommend benefits of breast MRI targeted to select patient populations. These recommendations include screening studies for early cancer detection to improve survival, and diagnostic studies for evaluation of extent of disease, better diagnosis for improved treatment planning, and improved prediction for early assessment of response to therapy. It is apparent from published data that both for screening and diagnostic indications, a large variability in recommendations and utilization for breast MRI studies exists, despite existing clear guidelines regarding the use of MRI for screening and for clinical management of patients with breast cancer. This problem is clearly evident in the variability in use of diagnostic MRI for preoperative work-up of women with a new cancer. These discrepancies in recommendations for MRI exist not only in older women but in young women as well. There is a need to increase standardization of referrals to optimize clinical management and care for all women. 14
The need for improved screening methods for women at average to intermediate risk has come into focus in recent years, due to the passage of breast density legislation in over half of the U.S. states, requiring that women be informed about the possible benefit of additional screening methods. Historically, the main barriers to MRI use have included limited availability, high costs, long examination times, and limited exposure of the benefits of MRI to the medical community and to patients. In an ideal world, cancer screening should be accurate, inexpensive, and widely available; however, many radiologists use lengthy breast MRI protocols lasting 30 minutes or more and costs are high in the United States. Counseling of high-risk women at the time of a recommendation for MR screening is beneficial, and many cities today have genetic counseling clinics for this purpose. Referring physicians and radiologists may also use a risk assessment model for patient guidance.
ACR guidelines recommend MRI screening for high-risk women at a greater than 20% lifetime risk of breast cancer starting at age 30. MRI is the best method for detection of early node-negative breast cancer, and many clinical trials conducted in the United States and Europe showed significantly improved cancer detection in high-risk women, finding small cancers that were occult on clinical, mammographic, and ultrasound examinations (Fig. 1‑1). 5 , 7 , 16 , 17 , 18 , 19 , 20 , 21 , 22 , 23 In the United States, 28 (56%) states have already passed breast density legislation and the number is growing. State laws now require that women be informed if their breasts prove to be heterogeneously or extremely dense at mammography suggesting that supplemental breast cancer screening could be considered. Given that approximately 50% of women younger than 50 years and 33% of women older than 50 have dense breasts at imaging, the need for improved screening methods is clearly needed.
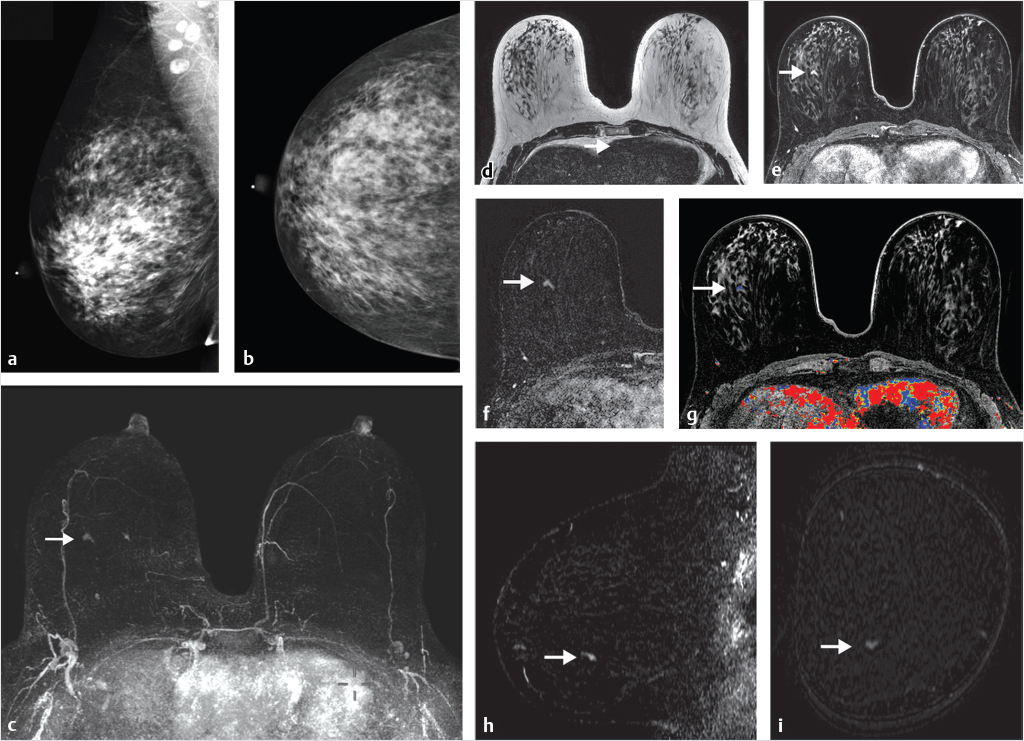
1.5 Screening MRI
As use of breast MRI has matured, there is now evidence to suggest that the indication for breast MRI will dictate the type of MR protocol to be used. Just as many years ago mammography services were divided into a screening or a diagnostic indication, this same distinction could now be applied to MRI examinations. An abbreviated screening examination could be used for cancer detection in asymptomatic women, and a full standard diagnostic study could be used for other indications such as the evaluation of a clinical problem when conventional imaging is uncertain and assessment of disease extent in patients with newly diagnosed breast cancer. Radiologists could therefore develop and implement dedicated acquisition protocols for screening and diagnosis, and create new patient and imaging workflow.
I think it is fair to say that the basic MRI acquisition protocols for breast imaging have not changed greatly over the last two decades, although hardware and software improvements have led to greatly improved image quality over time. Most clinical breast MR practices today do not distinguish between screening and diagnostic indications. In general, a screening study could consist of an abbreviated acquisition protocol performed within a time limit of 3 to 10 minutes, and a basic diagnostic acquisition within 15 to 20 minutes. Screening MRI studies must be efficient and affordable with short imaging time. Designated magnet availability and well-organized patient workflow can deliver short screening studies at a lower financial cost. It is possible that, in the future, dedicated breast MR systems optimized on a scale for population-based screening will be developed and used extensively in breast practices for screening. Dr Kuhl and colleagues have successfully pioneered an early proof-of-concept study involving interpretations of an abbreviated breast MRI protocol (AB-MR) and have shown equivalent sensitivity for cancer detection in women at mild and moderate risk when compared to a full standard breast MRI. 24 Other early studies involving interpretations of an AB-MR protocol have demonstrated equivalent sensitivity for cancer detection when compared to a full standard MRI, with only minimal decrease in specificity. 25 , 26 , 27 , 28 , 29 Further research on the outcome of screening results using an abbreviated protocol is needed.
1.5.1 Technical Considerations
Standard Protocols
As we look at the key technical components of any breast MRI protocol, abbreviated or standard, it is clear that an excellent T1-weighted (T1w) dynamic series with injection of a gadolinium-based contrast agent is essential for cancer detection. These contrast agents act by reducing the T1 in the microenvironment, increasing the signal in a T1w acquisition, so that regions or lesions with increased blood flow and permeability accumulate contrast media to a greater extent than the surrounding tissue, resulting in higher signal increase. The technical details outlined in the Breast Magnetic Resonance Imaging Accreditation Program sponsored by the ACR require both a T2w sequence and a multiphase T1w series. The dynamic series must include a precontrast T1w series, an early phase (first) postcontrast T1w series to be completed within 4 minutes of contrast injection, and a late phase T1w series with features matching the precontrast T1w series. 30 Measures of the rate of uptake and washout of contrast media within breast lesions have been shown to contain diagnostically useful information and the shape of the TIC is widely used in the classification of enhancing lesions. 31
Screening Protocols
The ACR Accreditation Program does not provide a specific protocol for screening indications. With this in mind, an AB-MR screening protocol could be constructed by shortening the dynamic component of a diagnostic examination to 2 to 3 minutes, acquiring data in the initial phase of enhancement only. An AB-MR study should fulfill the following requirements: standardized contrast administration based on kilogram weight using a power injector, total scan time of shorter than 10 minutes (including localizer), one pre- and one or two postcontrast gradient echo (GRE) fat-suppressed T1w axial acquisitions, with in-plane resolution of 1 mm or less, slice thickness of 3 mm of less. Addition of an axial T2w sequence with in-plane resolution matching the GRE sequences and 3 mm or less slice thickness is useful for improved specificity. The T1w dynamic sequence in diagnostic protocols would remain the same, and the traditional acquisition of dynamic data for 5 to 7 minutes following contrast injection and acquisition of DCE data in both the initial and delayed phase, as specified in the ACR Accreditation Program, would remain.
In the near term, however, a new focus on adaptation and modification of the basic DCE T1w sequence has yielded promising results. Implementation of a short sequence for screening, using an abbreviated dynamic sequence (AB-MR), and use of ultrafast perfusion imaging in the initial dynamic phase are both relatively new methods, adapted for screening, and have already begun to be implemented in some radiology practices. A new ECOG/ACRIN trial “Comparison of Abbreviated Breast MRI and Digital Breast Tomosynthesis in Breast Cancer Screening in Women with Dense Breasts (EA1141)” opened in October 2016, and patient recruitment is now completed for the initial round. Details of the protocols used for screening and diagnostic indications will be provided in ensuing chapters.
1.6 Diagnostic MRI: Clinical Applications
Diagnostic applications of breast MRI will be discussed below and include assessment of disease extent at time of initial cancer diagnosis, postoperative evaluation of residual disease following cancer resection, identification of recurrent tumor, and monitoring of women undergoing cancer treatment with neoadjuvant chemotherapy. Other applications include problem solving for difficult clinical or imaging findings, nipple discharge, implant assessment, and use of MR-guided biopsy. 14 High-quality images are essential for accurate diagnoses of breast disease. This goal is challenging in MRI because all current breast protocols require DCE-MRI sequences, accurate contrast administration, and optimal standard acquisitions. Sensitivity for cancer detection is already very high, and various new innovative acquisition protocols, such as diffusion-weighted imaging (DWI) and fat suppression methods using short TI inversion recovery (STIR) and high spectral and spatial resolution (HiSS) imaging, raise the future possibility of improved diagnostic specificity and cancer detection, without the use of contrast agents. These advanced imaging techniques will be discussed further in ▒Chapter 11░.
1.6.1 Preoperative Breast MRI
Can preoperative breast MRI help reduce reoperation rates by providing careful mapping of disease extent? It has been shown in many studies that the use of multimodality imaging, including mammography, ultrasound, and especially MRI along with the additional use of percutaneous biopsy techniques, can produce a precise map of the extent and localization of breast disease for surgical guidance. Although no large studies have proved a survival advantage with use of preoperative MRI and questions remain as to reduction of re-excision and recurrence rates, the potential benefit of preoperative MRI is valuable. MRI can identify foci of invasive disease with greater sensitivity than other imaging methods allowing precise identification of multicentric and multifocal, invasive and noninvasive disease that would otherwise go undetected. (Fig. 1‑2). Identification of additional foci of cancer may lead to a more extensive lumpectomy than originally planned, or even to mastectomy. Surgeons need to know if there is any malignant nipple involvement because if tumor is present, resection of the nipple–areola complex is usually needed. MR can identify DCIS lesions exhibiting linear enhancement extending into the subareolar region close to the nipple, which are occult at mammography (Fig. 1‑3).
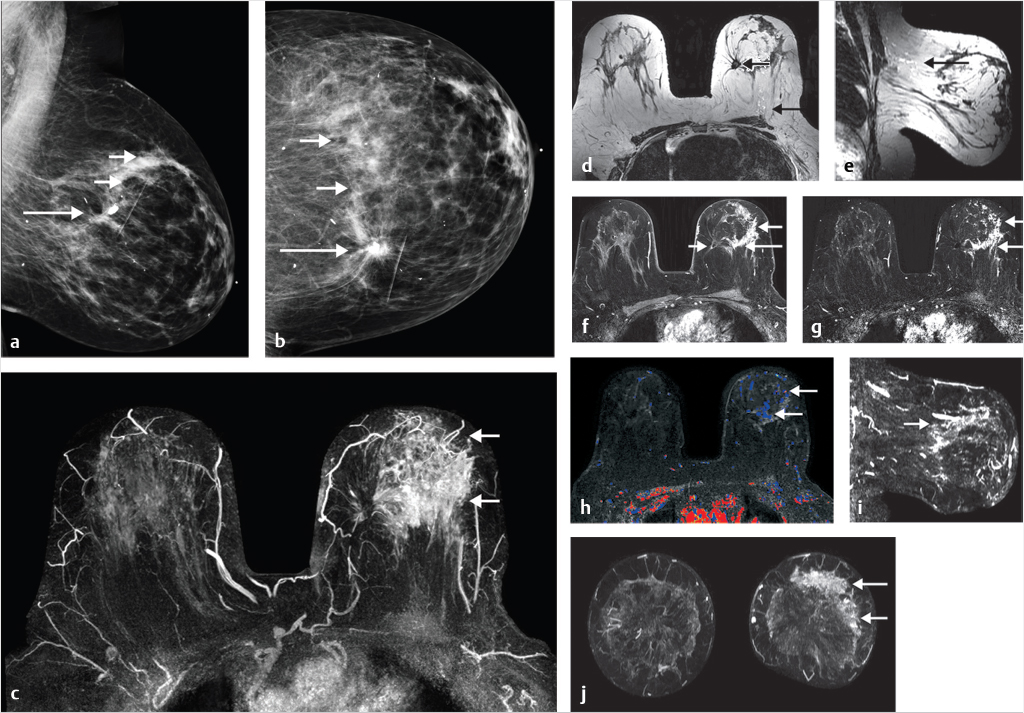
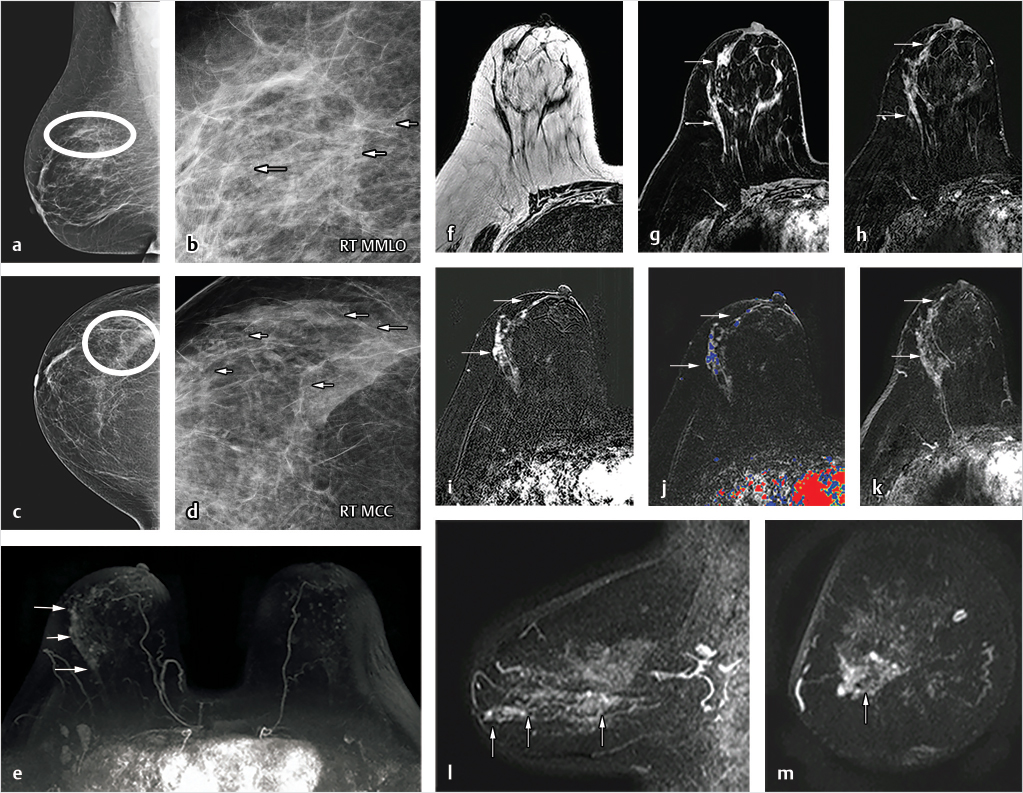
MRI may also demonstrate axillary and internal mammary lymphadenopathy and chest wall invasion, which can affect surgical and radiotherapy management. Historically, some treating physicians have acted on MRI findings and altered surgical plans without prior histologic confirmation. Optimal patient care in most of these cases requires tissue sampling before definitive treatment, when additional disease identified on MRI is located beyond the location of the known malignancy.
Complete assessment of the tumor burden requires measurement of not only the size of the tumor component(s), but also the full volume of the extent of disease, both invasive and in situ. Multifocal disease may consist of in situ cancer only, multiple invasive cancers with intervening normal tissue, or multiple invasive cancers associated with an in situ component (Fig. 1‑4). Accurate tumor measurements are important for surgical and oncologic therapy planning; they are necessary not only at time of initial presentation, but also for monitoring during subsequent chemotherapeutic treatments. Two large studies that carefully examined newly diagnosed breast cancers with serial tumor sectioning at pathology found the majority of tumors to be multifocal (66 and 63%) with tumor foci extending beyond the known index cancer. 32 , 33 It is evident that tumor multifocality identified at MRI cannot be accurately assessed by a single maximum diameter measurement of the cancer in most cases. Newer computer-generated volume and surface area calculations reflect a more accurate estimate of tumor burden. Radiologists can facilitate removal of all malignant tissue and provide surgical guidance, by using image-guided biopsy methods with clip placement to identify additional disease prior to surgical excision. Translation of imaging data to the surgical environment, however, may be challenging, and although knowledge of the true extent of cancer is an important requisite for successful surgery, the literature is varied as to whether identification of additional ipsilateral or contralateral occult malignancy is beneficial.
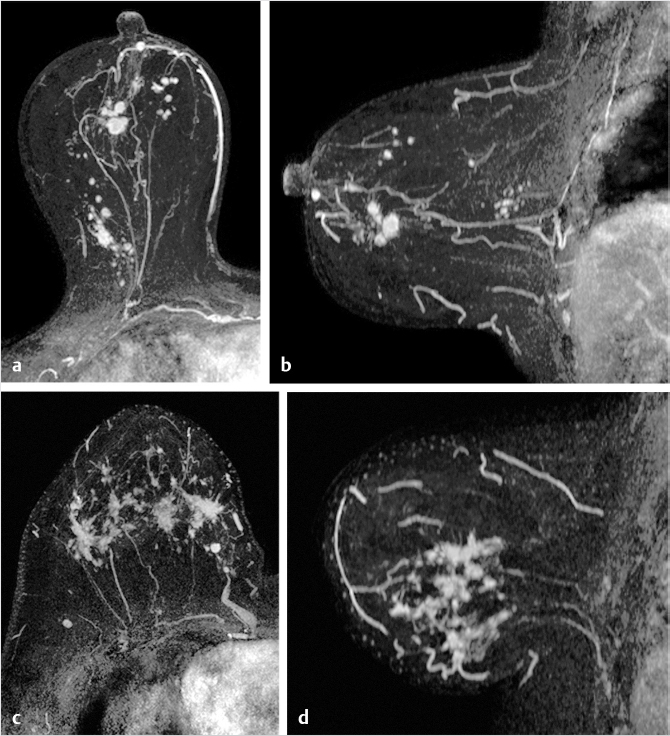
1.6.2 Postlumpectomy with Positive Margins
Breast-conserving therapy with partial mastectomy is one of the most commonly performed cancer operations in the United States and estimates indicate that 60 to 75% of breast cancer cases undergo partial mastectomy as the initial treatment. 34 The challenge for breast imagers, surgeons, oncologists, and pathologists is to adopt evidence-based strategies that aim to conserve the breast if possible, minimize reoperations, and maintain good cosmesis. In any given case, the extent of surgery for tumor removal may range from a simple gross excision to a wide lumpectomy or quadrantectomy.
Ideally, all cancer should be removed at the time of initial surgical excision and failure to achieve appropriate pathological margins at initial operation usually necessitates additional surgery. 35 , 36 , 37 , 38 The appropriate treatment for patients with close histologic margins at tumor excision is not yet completely established, and an extensive literature, mostly retrospective, shows wide variation in definitions of surgical technique, specimen processing, and outcome reporting of acceptable margin status. Definitions such as “no tumor within 1, 2, 5, or 10 mm of the margin” are still used in clinical practice; however, in a recent paper, the Society of Surgical Oncology—American Society for Radiation Oncology guideline defines “no tumor on ink” as an adequate margin. 39 Factors contributing to positive surgical margins include the size and location of the primary tumor, and the presence of an extensive intraductal component (EIC) within an invasive ductal carcinoma (IDC). 32 , 40 , 41 , 42 It is generally accepted that the presence of microscopically positive margins necessitates re-excision surgery in order to decrease the risk of local recurrence. 43 , 44 , 45 , 46 , 47
Reoperation Rates
There are large variations in reoperation rates following cancer excision among surgeons and institutions, and reoperation rates have been shown to be high in the United States. 48 , 49 Current estimates of the frequency of surgical re-excisions range from 30 to 60%, 43 , 44 , 45 , 46 and the burdens of reoperation are considerable, with increased patient morbidity and costs. Additional operations may delay the use of recommended adjuvant therapy and cause psychological, physical, and economic stress for patients, especially when more extensive surgery such as mastectomy is required. Once re-excision surgery is performed, a review of the literature indicates that the frequency of tumor in second surgical excision specimens ranges from 32 to 63%, microscopic residual disease being found in only about 50% of patients overall. 41 , 42 Residual tumor has been shown to be associated with an increasing risk of recurrent cancer in the ipsilateral breast, for both invasive and noninvasive cancer, with some investigators showing increased local recurrence rates, 50 , 51 whereas others have indicated no increase in recurrence rates. 52 , 53 , 54
Today, even with declining reoperation rates, too many women still end up back in the operating room after a partial mastectomy. A recent editorial in the New England Journal of Medicine stressed that the rate of reoperation can and should be reduced, and the authors further point out that even a reduction of 10% in the re-excision rate nationwide could result in reoperation being avoided for 10,000 to 20,000 women annually. 55 The variability in reoperation rates among surgeons and institutions speaks to the important need for professional societies to provide guidelines and further education for surgeons and their trainees.
Diagnosis of Residual Disease
Clinical examination of the breast after tumor resection is usually limited because of focal thickening, pain, and swelling at the surgical site. Patient discomfort, breast edema, postoperative inflammatory changes, and postsurgical hematoma and seroma fluid collections all may limit the value of mammographic and ultrasound studies in the immediate postoperative period. 56 , 57 Mammography, however, using minimal compression, may detect residual malignant microcalcifications, which may prove useful in guiding re-resection in certain cases. 58 MRI is the primary imaging modality for this task. Findings suspicious for residual tumor include irregular thickening of the seroma wall and adjacent mass or nonmass enhancement (Fig. 1‑5). MRI is very sensitive to the detection of residual disease, despite normal postoperative changes that may limit the positive predictive value and specificity of residual cancer detection in some cases. Limiting factors include contrast enhancement caused by postsurgical inflammatory changes and fat necrosis. 59 , 60 Although the overlapping features of postsurgical changes and malignant lesions remain as limitations, DCE-MRI has proved to be a useful tool for identification and guidance of residual disease. 61 , 62
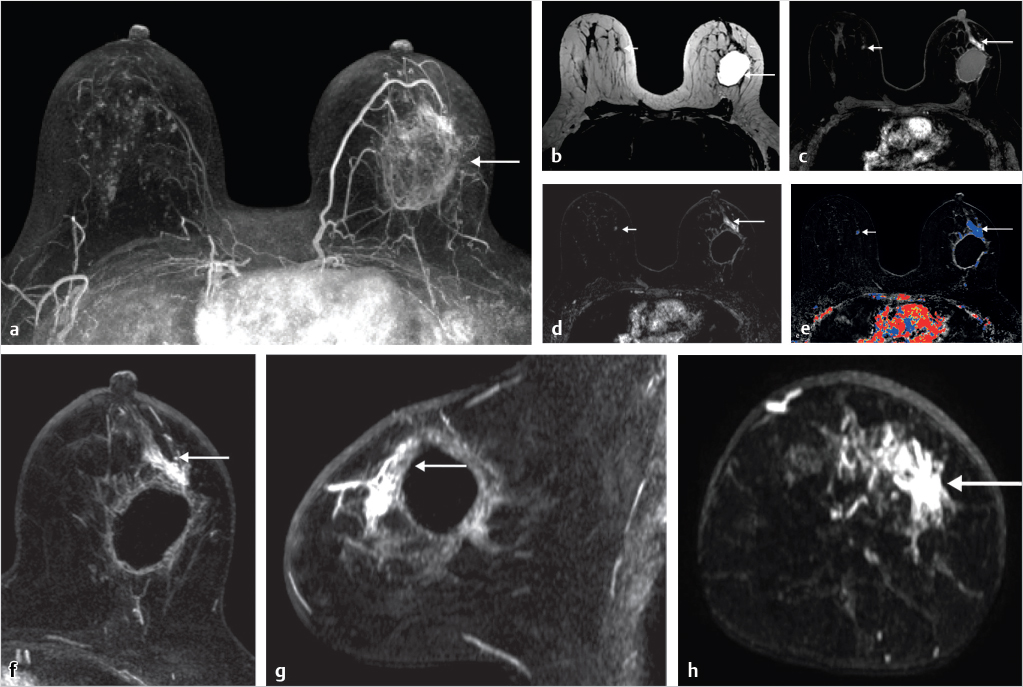
1.6.3 Assessment of Neoadjuvant Chemotherapy Treatment
Neoadjuvant chemotherapy treatment (NACT) before surgery for local regional control is the standard recommended treatment for locally advanced breast carcinoma (stage III B and C). NACT is widely used prior to surgery for invasive cancer, the goal being to improve overall survival by eradication of micrometastases with the additional potential benefit of tumor volume reduction during treatment that might allow for more conservative surgery. Large, long-term clinical trials (NSABP 18 and 27) 63 have shown no statistically significant difference in relapse-free and overall survival between adjuvant and neoadjuvant treatment groups, although a trend toward an increased benefit in women younger than 50 years of age was shown in the NACT group. Patients with stage II and IIIA disease may also receive a benefit because of an increased likelihood of successful breast conservation as a result of a decrease in the size of the index lesion following NACT. 64 Favorable long-term outcomes are associated not only with a decrease in the index tumor size as evidenced on imaging during NACT, but also with successful treatment of axillary lymphadenopathy. Change in tumor size has been shown to be a clinically useful measure of tumor response and is predictive of patient survival.
MRI is the most accurate imaging method for monitoring treatment response during therapy and for assessment of the extent of residual disease at the end of therapy, prior to surgical treatment. In vivo monitoring with MRI is noninvasive and allows physicians to test the effects of treatment and to change the treatment in case of nonresponse. MRI studies are generally repeated three times: a pretreatment study serving as a baseline examination for comparison with posttreatment studies, a second study after one or two treatments, and a third study after the final treatment before surgery. Response to treatment is based on accurate pre- and posttreatment documentation of any decrease or increase in overall tumor size and volume, compared to the pretreatment MRI. There is variability in the imaging appearance of tumors as they respond to treatment. Invasive cancers presenting as masses on MRI often exhibit concentric shrinkage, are easier to measure, and accord good histologic and imaging concordance after therapy (Fig. 1‑6). Other invasive cancers, presenting initially as multifocal lesions or as nonmass enhancement, may exhibit an overall volume decrease with heterogeneous tumor regression following therapy, but without a significant change in maximum tumor diameter (Fig. 1‑7). Assessment of tumor volumes and surface areas using computerized analytical software can provide more accurate comparison measures than traditional linear measure of maximum tumor diameter. 64 , 65 MRI documentation of treatment response is traditionally recorded as follows. Absence of any enhancement at the site of the prior index lesion(s) is recorded as a complete response (CR). Reduction in the index cancer size by greater than 30% is recorded as a partial response (PR). Decrease in the size of the index cancer less than 30% is recorded as no response (NR). MR studies performed after the first cycle of chemotherapy often provide the best opportunity for the radiologist to assess early tumor response. In the event that a tumor shows no response or even progression of disease on imaging after the first treatment, modifications to the treatment protocol can be considered, thus sparing the patient from prolonged and ineffective chemotherapy.
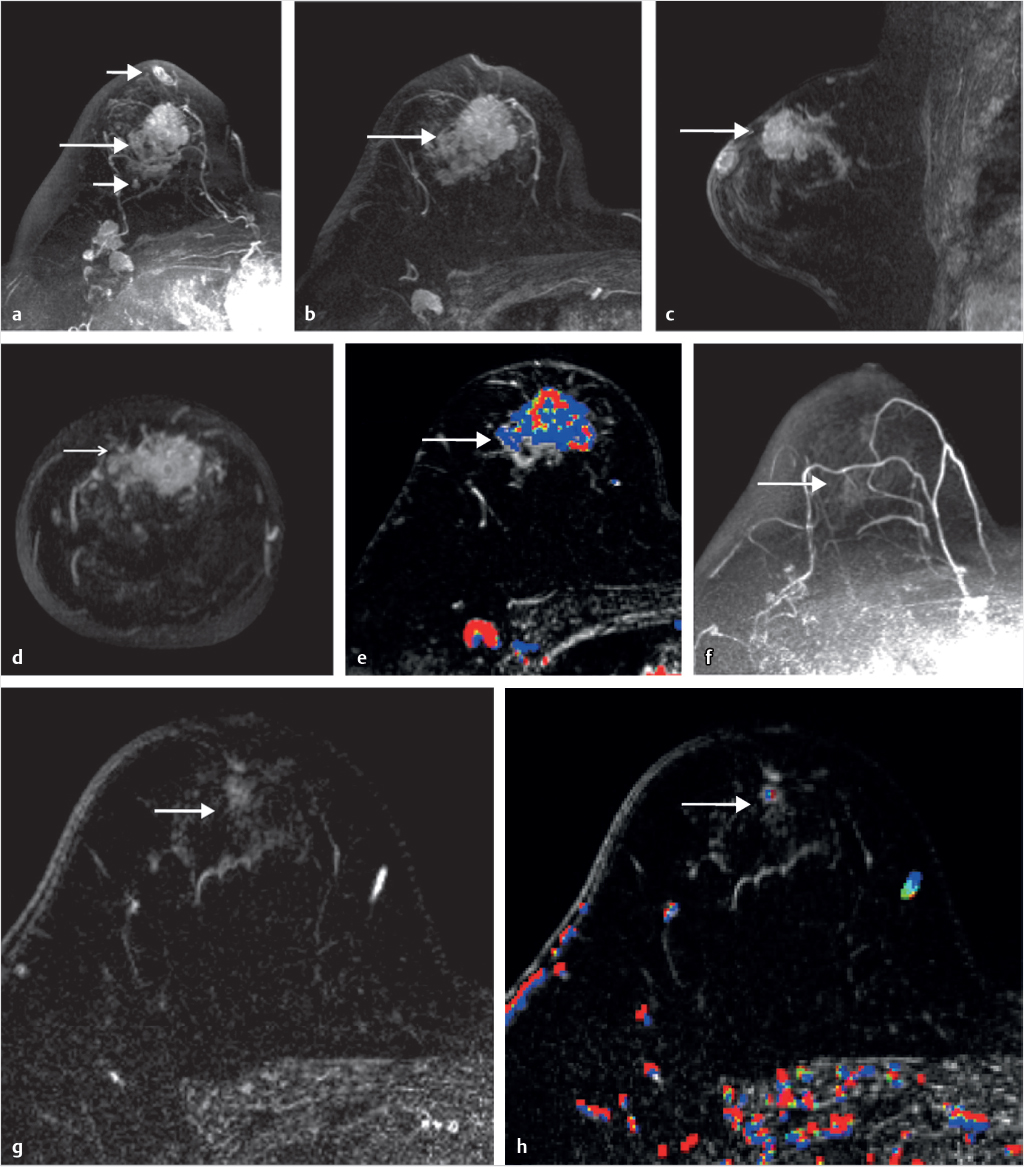
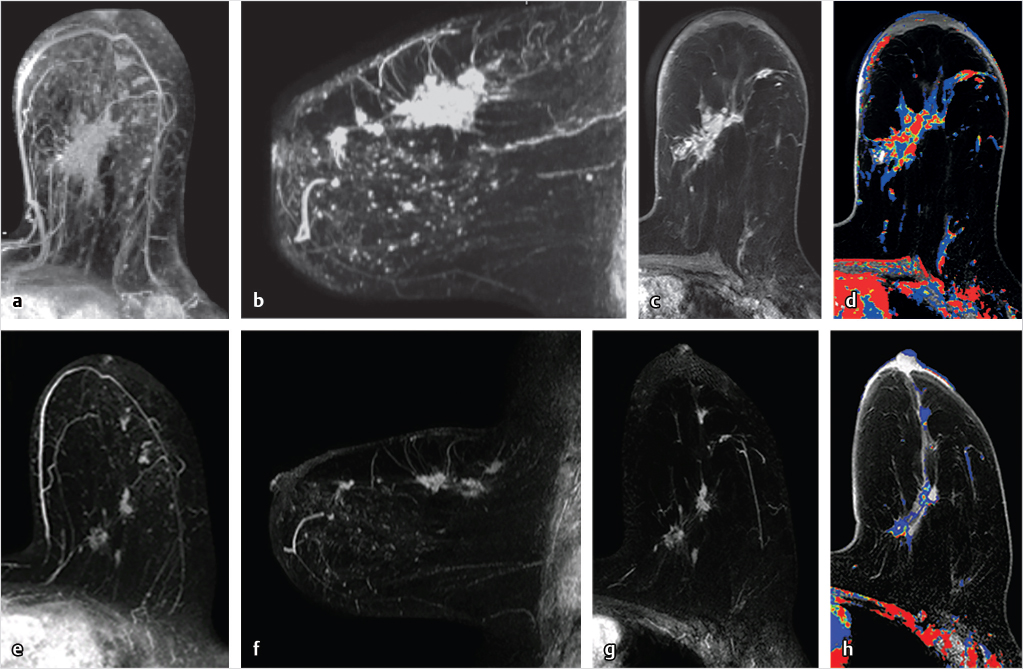
1.6.4 Breast Cancer Recurrence
The main goal of breast conservation is local control. The 10-year rates of local recurrence after breast-conserving surgery (BCS) are already quite low, 6% among patients with node-negative disease 66 and 9% among those with node-positive disease. 67 Furthermore, modern adjuvant therapy for breast cancer has improved so much that it has become very challenging to show an improvement in outcomes as evidenced by local recurrence rates. As previously discussed, current surgical practice mandates that no tumor be present on inked specimen margins at BCS, and re-excision surgery to achieve “no tumor on inked margins” is frequently employed. Furthermore, many patients receive adjunctive systemic chemotherapy and/or long-term antihormonal treatment. Therefore, current advances in treatment of breast cancer are unlikely to reduce recurrence rates much further.
Breast MRI is the preferred method for detection of a recurrent or new malignancy among women who have been treated for breast cancer and is useful when clinical or other imaging studies are suspicious or inconclusive for diagnosis of recurrence. Tumor may be identified either in the native treated breast or in the autologous reconstructed breast following mastectomy. Women with a personal history of breast cancer are at high risk for additional cancer in the ipsilateral or contralateral breast, and increasingly, many of these women undergo MRI screening annually. 68 The breast treated with BCS, radiation therapy, and chemotherapy exhibits a variable decrease in background parenchymal enhancement (BPE) when compared with the contralateral, nontreated breast. This finding may be particularly conspicuous in younger women, and usually persists on subsequent MRI examinations (Fig. 1‑8). Li et al 69 reviewed MRI findings in patients who had undergone BCS with radiation therapy. In their study, they found not only a decrease in BPE in the treated breast, reflecting a decrease in vascularity due to radiation therapy, but also a lesser effect in the untreated breast, suggesting an additional systemic effect from endocrine therapy or chemotherapy.
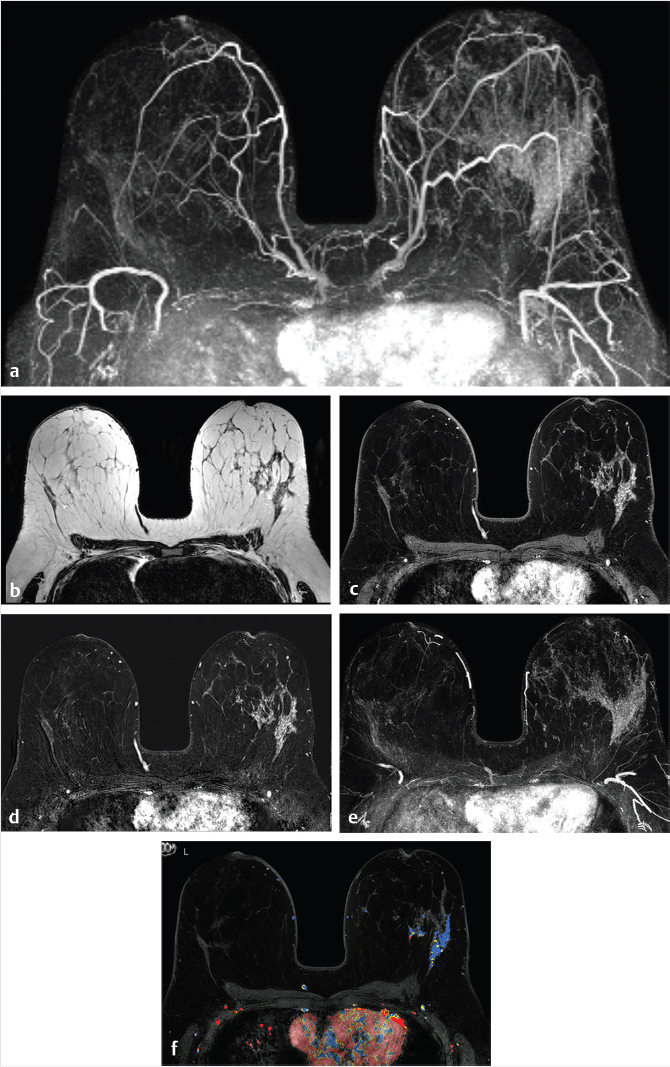
Normal postoperative MRI findings include postradiation edema, skin and parenchymal enhancement, and rim-enhancing seromata at the surgical site, which may remain visible for many months. Benign focal or postsurgical scar enhancement, with or without architectural distortion, is often present at the lumpectomy site in the immediate postoperative period; however, in some cases such changes may be minimal. Postoperative findings usually peak at 3 months after therapy, decrease by 6 months, and completely resolve within 12 to 18 months. Enhancement may remain at the surgical site for several years in some cases signifying the presence of fat necrosis. Recurrent tumor is generally easily identified in the setting of diminished BPE, as a new or increasing mass or area of nonmass enhancement, prompting biopsy (Fig. 1‑9). Fat necrosis may present as an irregular enhancing mass, requiring biopsy for definitive diagnosis (Fig. 1‑10). Signal voids are often noted at the surgical site reflecting the presence of surgical clips (Fig. 1‑11).
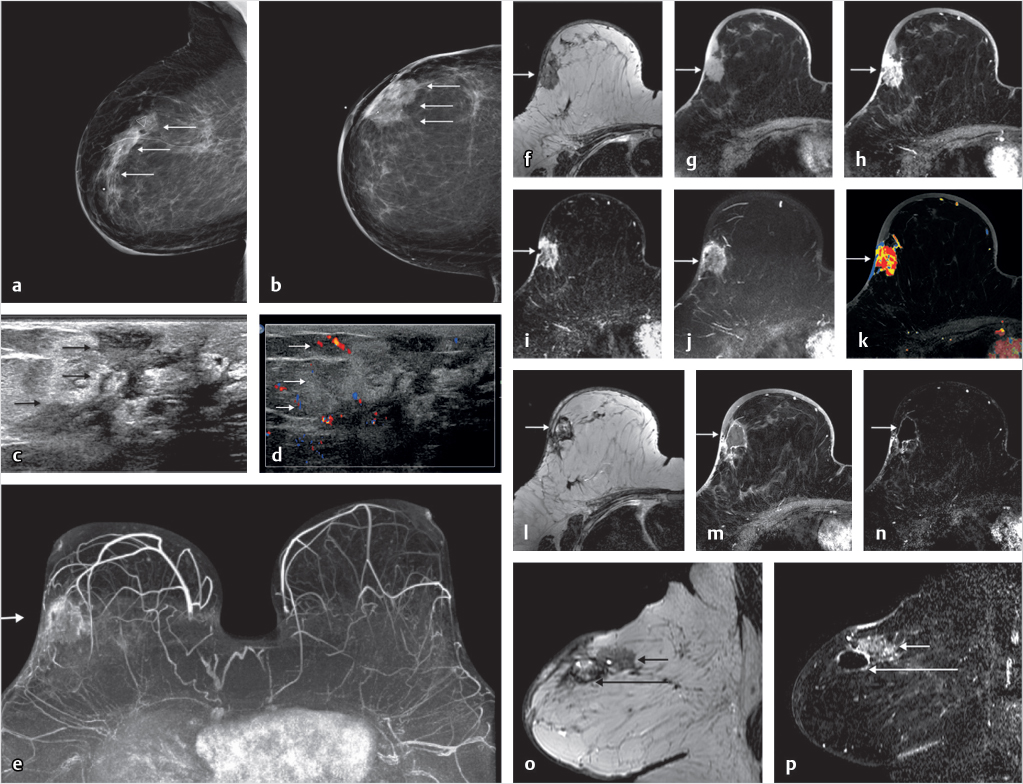
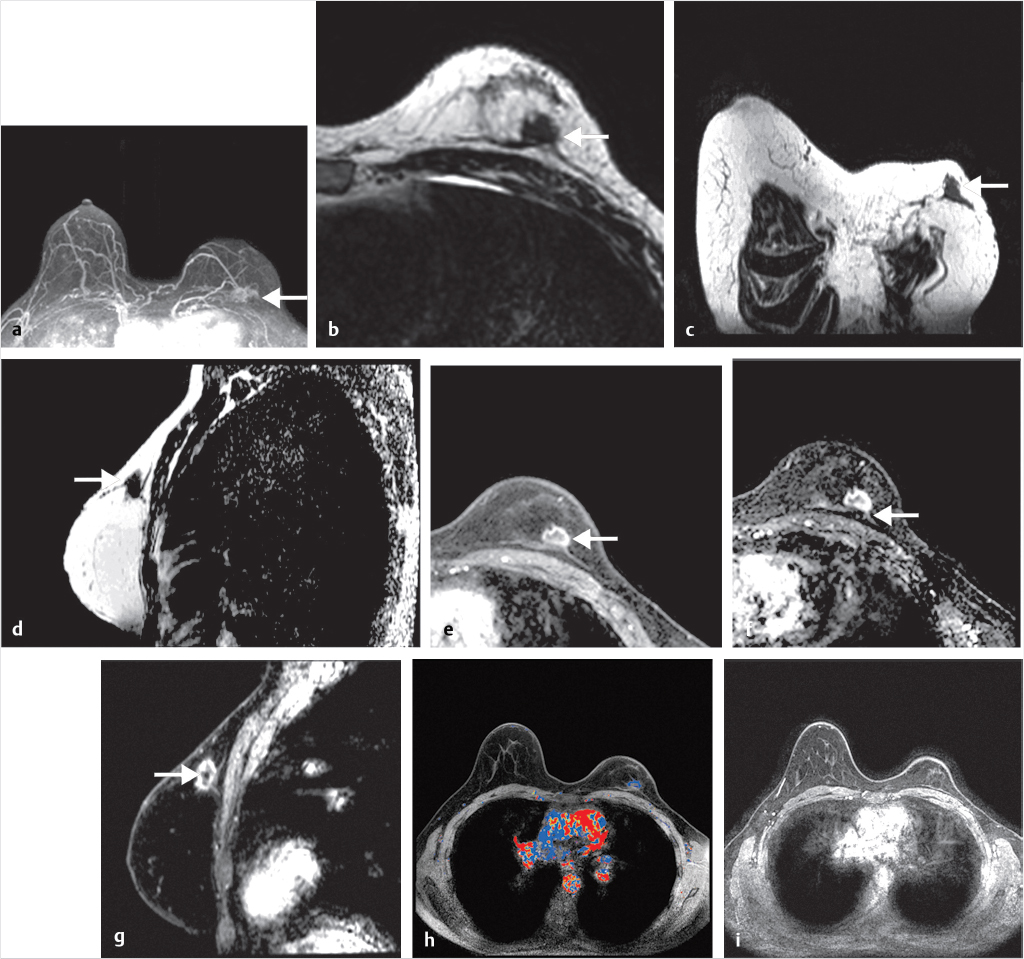
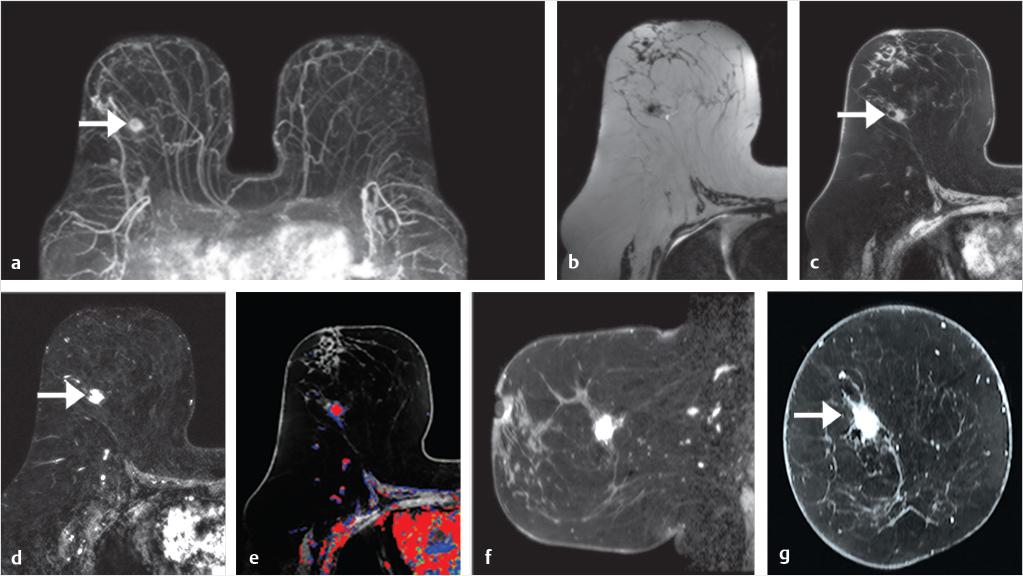
At the present time, it is uncertain as to whether recognition of occult malignancy by use of preoperative MRI could improve local failure rates and improve patient outcomes. Two retrospective studies, Solin et al (n = 756), with 8-year follow-up, 70 and Hwang et al (n = 472), with 4.5-year median follow-up, 71 each compared two patient groups following BCS, one group with and one group without preoperative MRI. Long-term follow-up found no significant difference in local failure rates between the two groups. The failure rates were low in both studies, with 3 and 2% recurrence rates in the MRI groups, and 4 and 3% rates in the non-MRI groups. Another study compared the rate of recurrent cancer between two patient cohorts, one with a group of 121 patients undergoing preoperative breast MRI, and a second group of 225 patients undergoing surgical excision without MRI. A significant reduction in local recurrences was found in the preoperative MRI group, 1%, compared to 7% in the non-MRI group (p < 0.001). The relatively short follow-up period of 3.4 years only is a limitation of this study. 72 It is important to consider that local cancer recurrence rates are already low (5–10% at 10 years), so that use of potential change in recurrence rates as a metric for determining the clinical efficacy of preoperative MRI is not meaningful. A study with a very long-term follow-up period might be able to elicit some measure of improvement in recurrence rates beyond the already low failure rates. Breast MRI should be a prerequisite for every patient under consideration for treatment with a localized radiation therapy protocol. Confirmation of unifocality at MRI is necessary to exclude areas of multifocal or multicentric disease, which could remain untreated if partial radiation treatment is employed. 73 , 74 , 75 , 76
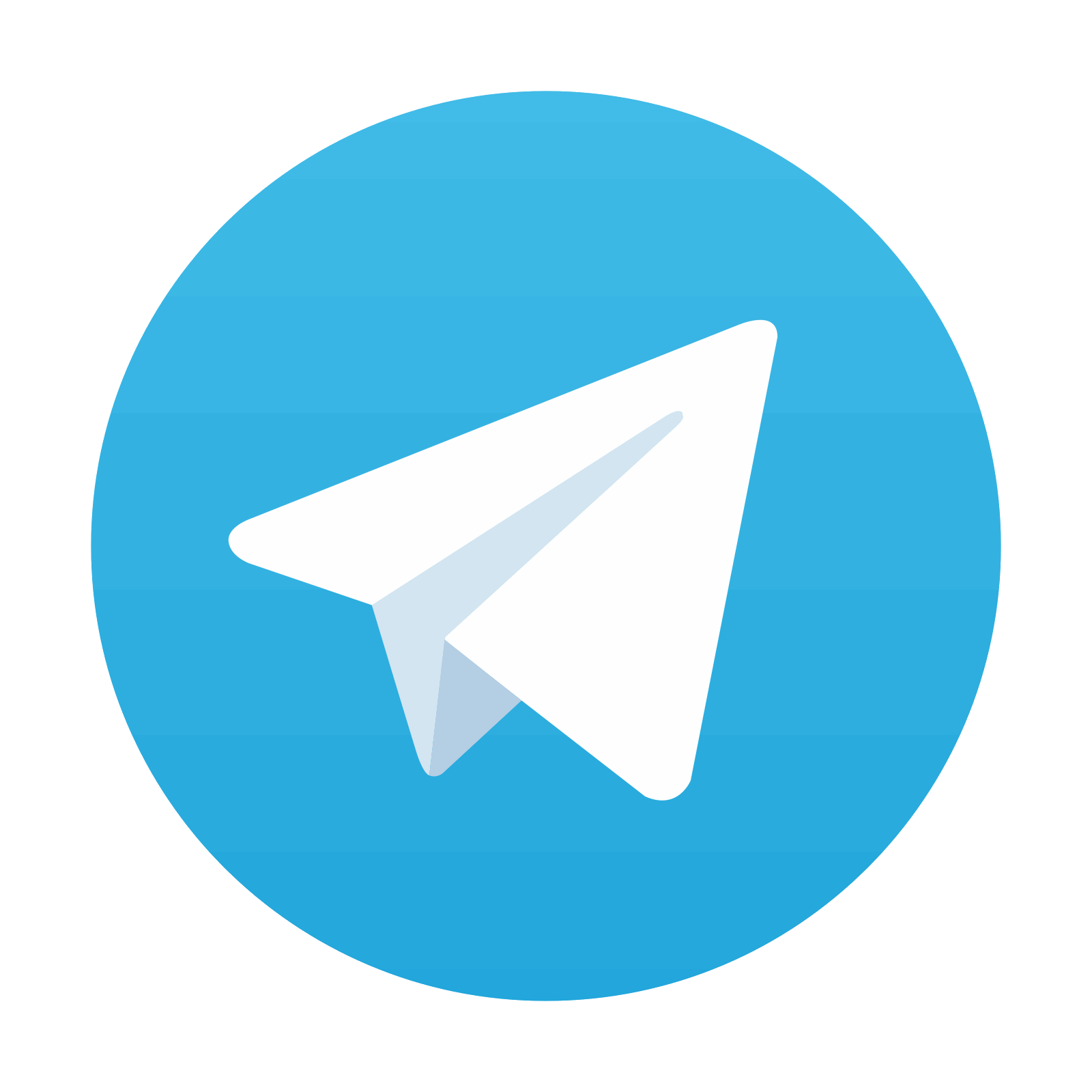
Stay updated, free articles. Join our Telegram channel

Full access? Get Clinical Tree
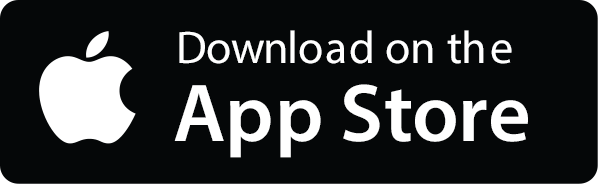
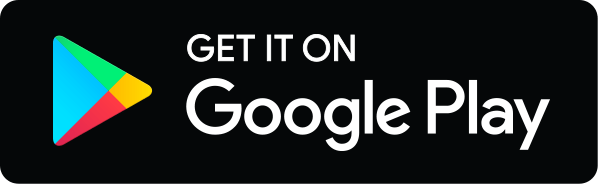
