15 Trauma and Fractures
Conventional radiography remains the primary diagnostic imaging modality for assessing fractures and dislocations. However, the role of computed tomography (CT) in fracture evaluation increased greatly with the introduction of helical multislice scanners, allowing shortening of examination time and display of images in multiple planes with superb resolution or three-dimensional reconstruction, respectively. Compared with conventional radiography, CT is overall superior to visualize skeletal pathology, but drawbacks of the latter imaging modality include greater expense, increased radiation dose, and both motion and metal artifacts.
The radiologic diagnosis of an acute fracture is usually not associated with any problems. A sharply demarcated fracture line is the hallmark of an acute fracture. Occasionally, however, a frank fracture line cannot be demonstrated in nondisplaced fractures even when conventional images in several projections are taken. In this condition, high-resolution CT usually allows arrival at the correct diagnosis by demonstration of a cortical break or disruption of the normal spongiosa pattern that could not be appreciated with the conventional radiographic examination. Because of its high sensitivity, magnetic resonance imaging (MRI) however, is the image modality of choice for identifying occult fractures for which correct early diagnosis is essential (e.g., femoral neck and scaphoid fractures).
CT is useful in the evaluation of all complex fractures, but the most common indications include fractures of the spine, scapula, and pelvis, fractures of long tubular bones extending into an articular surface, and fractures/dislocations involving carpal and tarsal bones. In all these locations, fractures are missed or underestimated in up to 50% of cases when conventional radiography is compared with CT, resulting not infrequently in a change of the patient’s management based on the additional information provided by CT. CT is often inevitable in the evaluation of the reduction of complex fractures and dislocations and is of considerable value in the assessment of complications in fracture healing.
Fracture healing begins with an inflammatory response resulting in the organization of the fracture hematoma by invasion of fibrovascular tissue. Bone resorption along the fracture margins becomes evident and in undisplaced fractures may allow at this stage (several days after the injury incidence) an unequivocal radiographic diagnosis. Periosteal and endosteal callus formation usually becomes visible 2 to 3 weeks after injury and is first evident as a thin periosteal reaction and irregular mottled calcifications about the fracture, increasing with time in density and finally developing bone texture. The healing process of a noncomplicated fracture from injury to consolidation takes one to several months. Fracture healing progresses more rapidly in oblique or spiral fractures, in a single fracture, and in younger patients. The healing process is slower in larger bones, in transverse fractures, in the presence of multiple fractures, in osteopenia, and with increasing age of the patient. Malunion refers to a fracture that is healed with significant fracture fragment displacement and/or angulation.
A delayed union is found with poor reduction, incomplete immobilization, in the presence of infection, in vitamin C and/or D deficiencies, and in areas of preexisting bone disease (pathologic fractures). Infections are particularly common in compound (open) fractures, where extensive soft tissue damage is caused by either a fracture fragment piercing through the skin or by an object (e.g., a projectile) penetrating from the outside.
Assessing partially united and nonunited fractures is especially improved by CT when compared with conventional radiography. Nonunion (Figs. 15.1, 15.2) is characterized by failure of fracture healing 6 to 9 months after injury. The fracture margins are well delineated and often sclerotic, and a frank area of intervening translucency is present. Nonunion may result from the same complications associated with delayed union or by interposition of soft tissue between the fracture fragments. Hypertrophic nonunion is commonly caused by continued motion at the fracture site. In these cases, the fracture line persists or excessive and prolonged bone resorption at the fracture margins occurs. Eventually the bone ends become sclerotic, and there is a varying degree of non-bridging external callus formation. Atrophic nonunion is thought to result from extensive bone death. The radiographic appearance is that of a persistent fracture line without demonstrable callus formation (Fig. 15.3). Nonunion may eventually progress to pseudoarthrosis formation.
Fracture healing in osteogenesis imperfecta is complicated by pseudoarthrosis formations with a higher incidence than in normal bone. Pseudarthrosis is also a common feature in neurofibromatosis, where it is most often found in the lower two thirds of the tibia. Pseudoarthrosis occurs also in fibrous dysplasia, which often demonstrates bone changes radiographically similar to neurofibromatosis. The two disorders can, however, often be differentiated by their skin manifestations. The café-au-lait spots in fibrous dysplasia are irregularly outlined, whereas they are smoothly outlined in neurofibromatosis. Furthermore, the presence of cutaneous fibromas is characteristic for the latter condition. “Congenital pseudarthrosis” involving the distal tibia and/or fibula shaft is a rare condition that may or may not be related to neurofibromatosis or fibrous dysplasia (forme fruste?).
A fracture may be complete or incomplete. The former implies complete disruption in the continuity of a bone. In an incomplete fracture, only some of the bony trabeculae are completely severed, whereas others are bent or remain intact. Incomplete fractures occur predominantly in elastic bones of children and young adults.
A dislocation is a complete disruption of a joint with the articular surfaces no longer in contact with each other. A subluxation is a less severe disruption of a joint in which some articular contact remains. Traumatic, habitual, pathologic (secondary to joint disease), paralytic, and congenital dislocations are differentiated.
Depending on their radiographic appearances, fractures are classified into different types (Fig. 15.4). Fracture alignment refers to the relationship of one fragment to another. Unless stated differently, the fracture displacement always refers to the distal fragment with regard to the proximal one. A fracture may be displaced in the transverse (horizontal) or longitudinal (vertical) plane, angulated, and/or rotated. Displacement in the transverse plane may be medial or lateral and anterior or posterior. Displacement in the longitudinal plane results in either fracture distraction or impaction. When the fracture fragments are completely separated, overriding of the fracture fragments with corresponding foreshortening of the bone (bayonet deformity) may occur. Fracture angulation may be medial (the distal fragment is angulated toward the midline, and the apex of the fracture is lateral, corresponding to varus deformity) or lateral (the distal fragment is angulated away from the midline, and the apex of the fracture is medial, corresponding to valgus deformity) and anterior (the apex of the fracture is posterior) or posterior (the apex of the fracture is anterior). Fracture rotation may be internal (distal fracture fragment rotates medially) or external (distal fracture fragment rotates laterally).
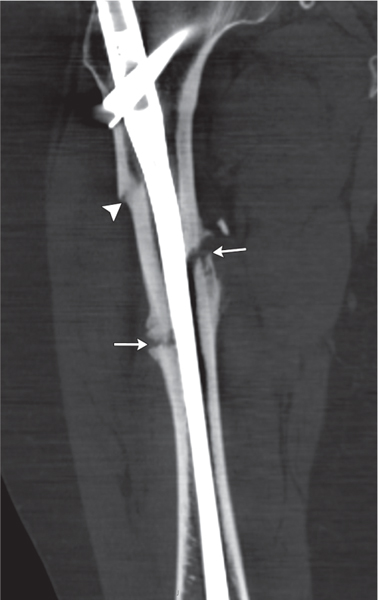
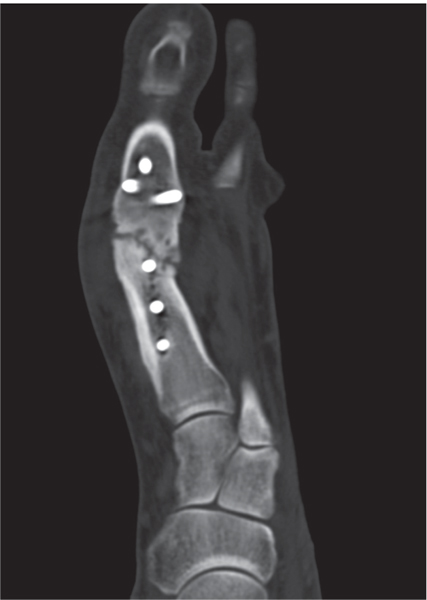
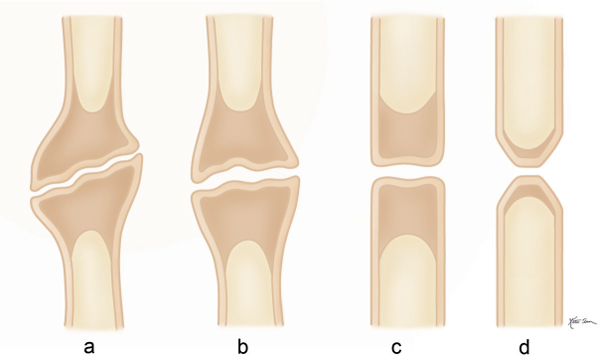
Fractures in children may present with special features. Greenstick fractures are incomplete fractures of the relatively soft growing bone perforating only one cortex and ramifying within the medullary cavity. Bowing fractures present as bending of the radius, ulna, or fibula without evidence of a bony break. Comparison radiographs of the opposite side are often required for correct diagnosis. Torus (buckling) fractures produce a buckling of the metaphyseal cortex in children and osteopenic adults (Fig. 15.5).
Trauma to the bone in children and adolescents often involves the cartilage (growth) plate, as long as the epiphyses are not closed. These injuries can be classified into different types using the Salter–Harris method (Fig. 15.6). A Salter–Harris type III fracture in the lateral distal tibia epiphysis is referred to as a juvenile Tillaux fracture (Figs. 15.7 , 15.8). A triplane (triplanar) fracture of the distal tibia in adolescents represents a variation of a Salter–Harris type IV injury (Figs. 15.9 , 15.10). Two, three, or four fragments (parts) may result, with two fragments being most common.
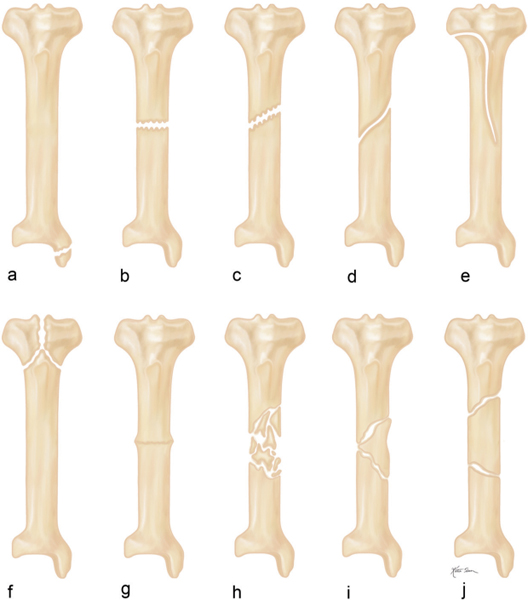
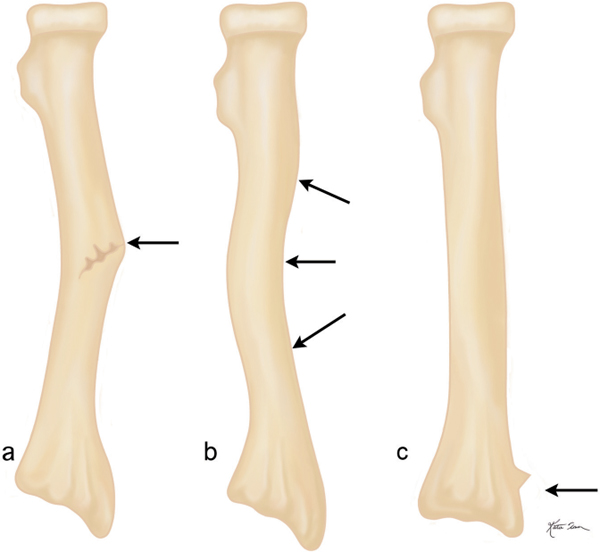
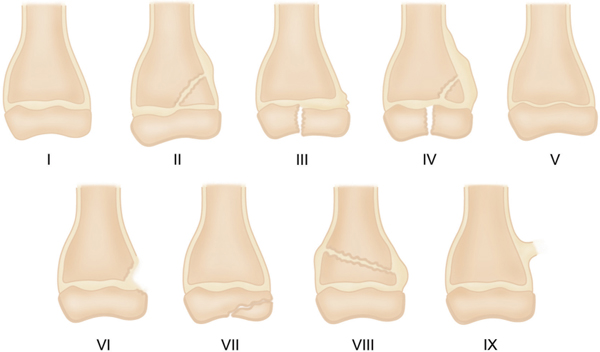
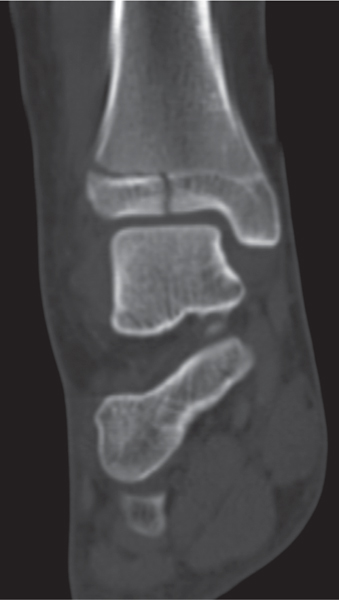
A tibial tubercle avulsion fracture in the immature skeleton is divided by the Ogden classification system into three types: avulsion through the secondary ossification center, extension of the fracture into the proximal tibial epiphysis, and extension through the tibial epiphysis into the knee joint (Fig. 15.11).
A traumatic epiphysiolysis of the femur head is particularly common in boys between 10 and 15 y of age, although a history of acute trauma is often not available. In these cases of slipped capital femoral epiphyses (SCFE), repeated low-grade trauma is believed to be the triggering mechanism (Fig. 15.12). Regardless of its etiology, SCFE represents a Salter–Harris type I fracture. The displacement of the femoral head in relation to the meta-physis is almost always in a posterior, inferior, and medial direction, and the physis appears blurred and widened. Sequelae of SCFE include remodeling and reactive bone formation in the femoral neck that may create a protuberance on its superolateral aspect known as Herndon hump. It is indistinguishable from the “pistol grip” deformity in femoroacetabular impingement (FAI).
In the pelvis, avulsion fractures at tendinous attachment sites such as the anterosuperior and anteroinferior iliac spines and ischial tuberosity are common before these secondary ossification centers are fused.
Multiple fractures and dislocations in an infant should raise the suspicion of an abused (battered) child syndrome (shaken baby syndrome). More subtle findings in this condition include injuries to the cartilage plate, metaphyseal fragmentation and avulsions (metaphyseal corner fractures), the latter producing a characteristic “bucket handle” deformity, posttraumatic metaphyseal cupping, and cortical thickening.
Selection of the appropriate fracture management requires accurate fracture classification that in complex cases is best accomplished with multiplanar CT. In most instances, the fracture staging system currently used in CT was originally devised for conventional radiography. A generic or universal classification system that can be applied to any bone within the body was designed by the Arbeitsgemeinschaft für Osteosynthesefragen/Orthopaedic Trauma Association (AO/OTA). Each fracture is identified by four numbers and one letter. The first number identifies the bone (e.g., 1: humerus, 2: forearm, 3: femur, 4: tibia, etc.). The second number identifies the location within the bone (1: proximal end, 2: shaft (diaphysis), 3: distal end). The fracture type is identified by a letter (A: two fragments only, B: comminuted, C: highly comminuted or segmental). The third number defines the group the fracture belongs to (fracture groups are different for each fracture type, with a scale ranging from 1 to 3). The fourth number defines the subgroup (most detailed fracture determination differing from bone to bone, with a scale ranging from 1 to 3). A complete description of this classification system is beyond the scope of this text. A simplified AO/OTA classification system may be applied for all shaft (diaphyseal) fractures where three types (1: noncomminuted, 2: comminuted but with contact between the main proximal and distal fragments [e.g. wedge fracture with butterfly fragment], and 3: comminuted but without contact between the main fragments [e.g., segmental fracture]) are differentiated. The fracture pattern (e.g., trans-verse, oblique, or spiral) should also be indicated. Furthermore, in the forearm and lower leg, the presence of subluxation/dislocation at the end of a fractured bone (e.g., proximal and distal radioulnar and tibiofibular joints, respectively) must also be assessed.
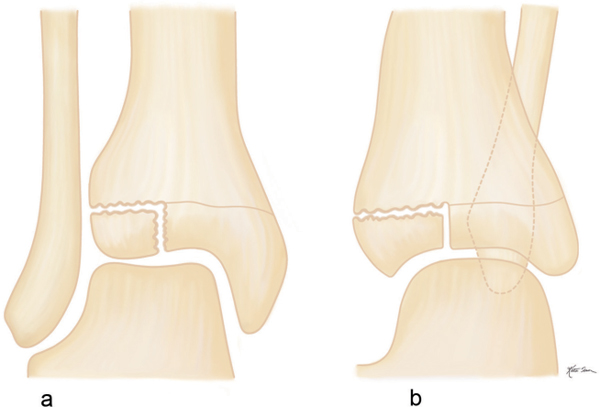
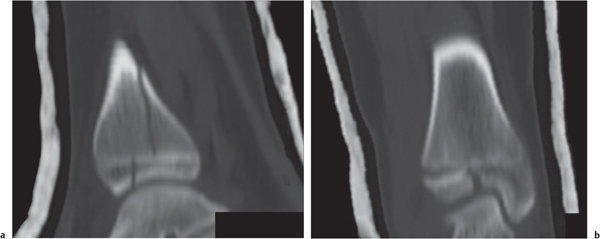
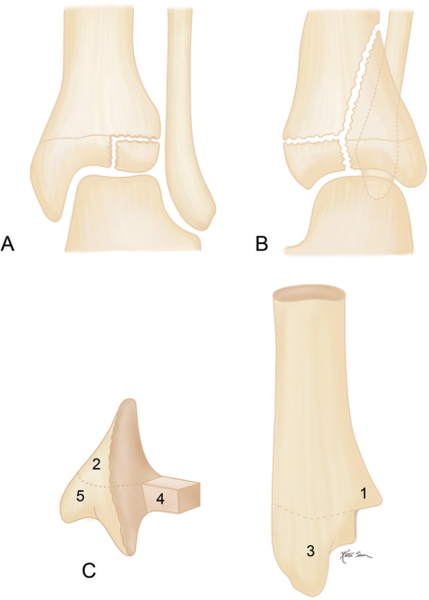
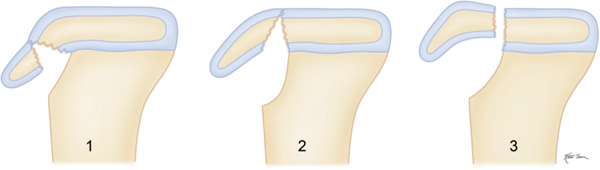
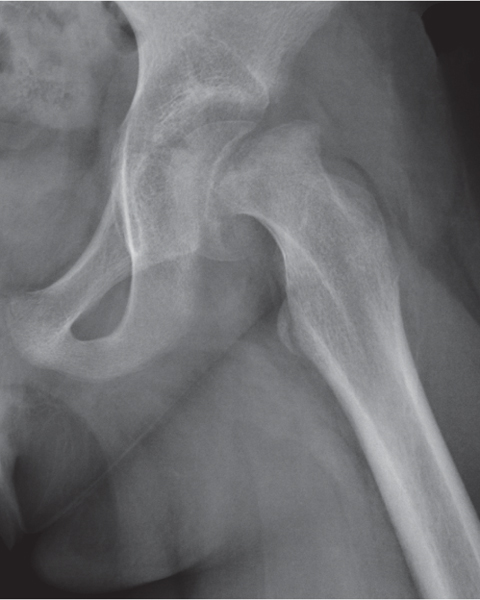
A fracture classification system adapted for specific sites appears more useful in clinical practice. Unfortunately, several different classification systems exist for virtually every fracture location, and there is no consent among orthopedic surgeons on the one to be most relevant for the patient’s management. In the following section, we have limited our discussion to the fracture classification systems that are preferred by the orthopedic surgeons at our institution.
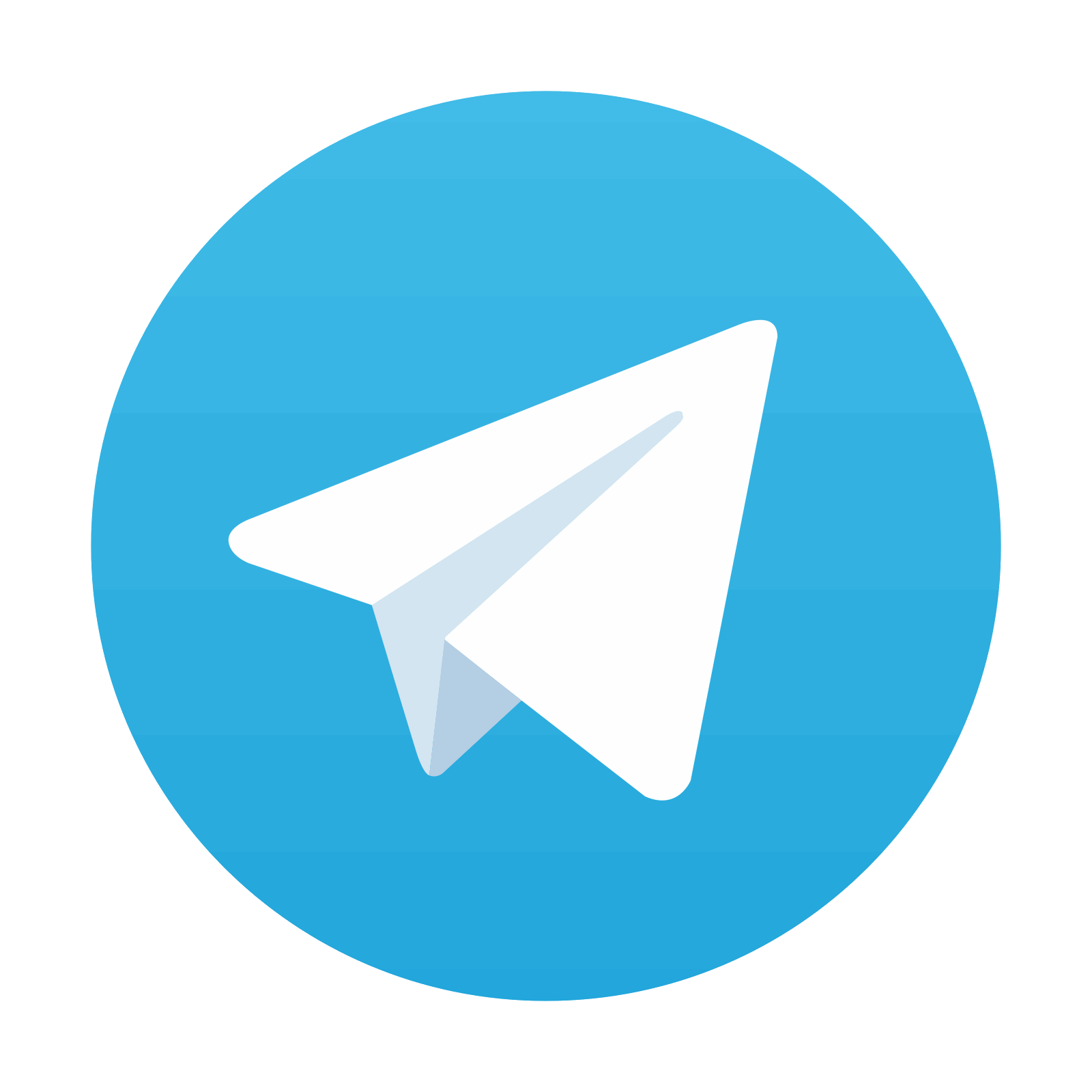
Stay updated, free articles. Join our Telegram channel

Full access? Get Clinical Tree
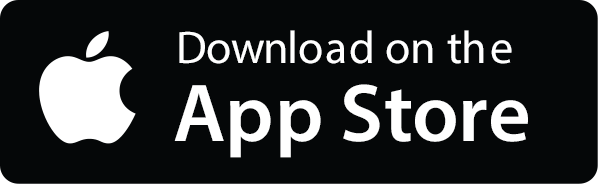
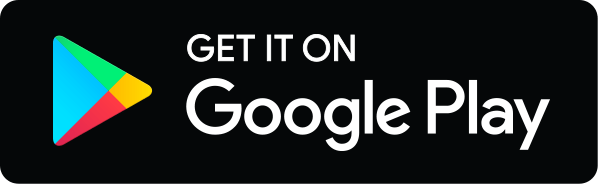