2 Radiopharmaceuticals for Clinical SPECT Studies
2.1 Introduction
George de Hevesy in the 1920s coined the terms radioindicator and radiotracer and introduced the tracer principle into biomedical sciences. A radiotracer can be defined as a specific radiolabeled molecule (or probe) that resembles or traces the in vivo behavior of a natural molecule and can be used to provide information about a specific biological process. One of the most important characteristics of a true radiotracer, however, is the ability to study the components of a homeostatic system without disturbing their natural function. Occasionally, the term radioligand is also used in the context of imaging studies. A radioligand is defined as any radiolabeled molecule that binds with another molecule or substance (binder) in a predictable way under controlled conditions.
All radiolabeled compounds or substances used for the purpose of diagnosis or therapy, however, have been defined as radioactive drugs or radiopharmaceuticals by the U.S. Food and Drug Administration (FDA). A radiopharmaceutical is a natural or synthetic chemical compound containing a radionuclide or a radioisotope. While the chemical compound with structural or chemical properties determines the in vivo distribution and physiological behavior of the radiopharmaceutical, the radionuclide provides the desired radiation characteristics for imaging or therapy. From a regulatory point of view, a radiopharmaceutical must be sterile, pyrogen free, safe for human use, and efficacious for a specific indication. FDA-approved radiopharmaceuticals for routine clinical single-photon emission computed tomography (SPECT) studies are listed in ► Table 2.1. Radiopharmaceuticals usually have no pharmacological effects, as they are used in minute quantities. Thus there is no dose-response relationship in this case, which differs significantly from conventional drugs.
2.2 Radiopharmaceutical
2.2.1 Ideal Properties of Diagnostic Radiopharmaceuticals
The radionuclide used to label the chemical compound should
preferably be a pure gamma (γ) emitter with energy in the range of 100 to 250 keV for optimum performance of a gamma camera.
have a physical half-life (t½) suitable for diagnostic use.
result in low radiation dose to the patient and nuclear medicine personnel.
The radiopharmaceutical should
have an in vivo biodistribution providing high target/background ratio.
be easy to prepare with very high (> 90%) radiochemical purity (RCP).
be stable in vivo without metabolite formation or release of free radiochemical impurities.
2.2.2 Mechanisms of Radiopharmaceutical Localization
The uptake and retention of radiopharmaceuticals by different organs and tissues involve many different mechanisms, as summarized in ► Table 2.2. The mechanisms of radiopharmaceutical localization may be substrate nonspecific (not participating in any specific biochemical reaction) or substrate specific, depending on the chemistry of the radiopharmaceutical. As the radiopharmaceutical may undergo metabolism in vivo, the observed biodistribution (based on imaging) would include the distribution of the intact parent radiopharmaceutical and also of its radiolabeled metabolites. In addition, the patient’s medication and several other factors may significantly alter the biodistribution of the radiopharmaceutical.
2.3 Radionuclides Used for SPECT Radiopharmaceuticals
The most common radionuclides used for diagnostic SPECT studies are summarized in ► Table 2.3. Technetium-99m (99mTc) is the most ideal radionuclide for SPECT radiopharmaceuticals because it decays by isomeric transition (IT) to 99Tc, with γ photons of 140 keV in high abundance (89%). Among the radionuclides that decay by electron capture (123I, 67Ga, 111In, 201Tl), 123I has an ideal γ energy (159 keV) with a high photon abundance (83%). The three metallic nuclides (67Ga, 111In, and 201Tl) have longer half-lives (~3 days) and deliver more radiation dose to the patient than 99mTc- or 123I-labeled radiopharmaceuticals. It is important to remember that the y photons from 201Tl are in low abundance and not useful for imaging studies. However, 201Tl decays to 201Hg, and the low-energy characteristic X-rays (~80 KeV) emitted as a result of this process are useful for imaging.
2.3.1 Production of Radionuclides
Radionuclides used for SPECT studies are produced in a nuclear reactor or a cyclotron. The nuclear reactions commonly used to produce radionuclides are shown in ► Table 2.4. In a nuclear reaction, when the atoms of a stable element (target) are bombarded by a subatomic particle (bombarding particle or projectile), the nucleus absorbs the subatomic particle and becomes unstable or excited (parent nucleus). Spontaneous decay results in the emission of radiation and/or particle(s) to form a product, which is usually a more stable nuclide. The equation for a nuclear reaction is written as follows:
where T represents the target nuclide; P is the projectile, the incident or bombarding particle; R represents the radiation (subatomic particle or γ photons) emitted by the compound nucleus; and, finally, Y represents the product unstable radionuclide. The P and R in parenthesis, written as (P, R), represent the nuclear reaction. A number of reactions, such as (p, n), (p, α), (d, n), and (n, γ), are some of the common nuclear reactions used to produce artificial radioisotopes.
2.3.2 Nuclear Reactor Produced Radionuclides
When a large fissile atomic nucleus, such as uranium-235 or plutonium-239, absorbs a thermal neutron (energy < 0.25 eV), it may undergo nuclear fission (f). The heavy nucleus splits into two or more lighter nuclei (the fission products), releasing kinetic energy, gamma radiation, and free neutrons. A portion of these neutrons may later be absorbed by other fissile atoms and trigger further fission events, which release more neutrons, and so on. This is known as a nuclear chain reaction. A nuclear reactor is a device used to initiate and control a sustained nuclear chain reaction. The fuel cells containing enriched 235U (as 235UF6 or 235UO2) pellets are surrounded by a moderator (graphite or heavy water) to slow down the energetic fission neutrons. Control rods capable of absorbing neutrons but not undergoing a nuclear reaction (such as cadmium and boron) are used to sustain the chain reaction.
When the reactor is in operation, the nuclear reaction (n, f) produces a number of neutron-rich fission fragments (Z = 28–65), which decay by β ‒, or γ-emission. Radionuclides such as 99Mo and 131I accumulate in the fuel rods to enormous activities (thousands of gigabecquerels [GBq]). All the fission fragments can be separated chemically and purified to yield no-carrier-added (NCA), high-specific-activity radionuclides.
A nuclear reaction in which a neutron is captured or absorbed by the nucleus of a stable atom leading to a nuclear transformation is called neutron activation. The most common nuclear reaction is (n, γ), in which the target nucleus with a mass number A captures a neutron to become an unstable excited radioisotope of target nucleus with a mass number A + 1. The excited nucleus emits a gamma photon, and the product radionuclide decays by β-emission to reach a ground state. As the product nuclide is an isotope of the target element, the product cannot be separated and purified to avoid the contamination of the carrier. As a result, radioisotopes produced using (n, γ) reaction are generally very low in specific activity.
It is possible to produce carrier-free, high-specific-activity nuclides in a reactor. Certain (n, γ) reactions produce a short-lived radioisotope of the target element, which decays by β-emission to another unstable radioactive nuclide with relatively longer half-life compared to that of the intermediate. 131I can be produced by neutron activation of enriched 130Te isotope.
2.3.3 Cyclotron-Produced Radionuclides
A cyclotron is a particle accelerator in which charged particles (protons, deuterons, and α particles) are accelerated using a high-frequency alternating potential difference between two hollow D-shaped sheet metal electrodes called “dees” placed inside a vacuum chamber in a magnetic field. The resulting high-energy charged particle beam is directed toward a target (atoms of a stable element), chosen in such a way that the desired radionuclide is produced. Most modern-day medical cyclotrons are called negative-ion machines because they generate positive particle beams with high energies (10–75 MeV) and currents (50–150 μA). When the target atoms are bombarded by a positive charge, the product is a proton-rich (neutron-deficient) radionuclide of a different element that decays by electron capture or positron emission. As a result, the radioisotopes produced in a cyclotron have high specific activity and are carrier free. Common radionuclides produced in a cyclotron are summarized in ► Table 2.3.
Specific Activity
Specific activity is defined as the amount of radio-activity per unit mass of an element, molecule, or compound, where the mass is the combined mass of the radioactive and the nonradioactive (stable or cold) species. Units of specific activity include mCi/mg, Ci/mmol, or GBq/μmol. Since 1 mol represents 6.02 × 1023 atoms or molecules (Avogadro’s number), one μmol consists of 6.02 × 1017 atoms or molecules. The theoretical specific activities of several radionuclides are shown in ► Table 2.3.
Carrier Free
Carrier free means that the radioactive species is not contaminated with a nonradioactive counterpart, which is known as a carrier. When Radionuclides are produced using a cyclotron, the target element is converted into a different element (with a higher atomic number). As a result, cyclotron-produced radionuclides are theoretically carrier free. In reality, it is very difficult to eliminate the contamination of natural carbon, fluorine, or other trace metals during the synthesis procedure. Thus a more appropriate concept is NCA, which means a stable, nonradioactive species is not intentionally added. In certain cases, carrier may be added intentionally during radioisotope production to facilitate chemical and biochemical reactions. Such preparations should specifically be reported as carrier added.
2.3.4 Radionuclide Generators
A radionuclide generator is a device used to produce a daughter radionuclide from a parent radionuclide via radioactive decay, and the daughter radionuclide is then separated from the parent radionuclide. In this serial radioactive decay process, the daughter is continuously produced by the decay of the parent while the daughter activity is also decaying. At equilibrium, the parent and daughter activities appear to decay with the same rate, even if the two radionuclides have different half-lives. Two different equilibrium conditions (transient or secular) may exist, depending on how short lived the daughter radionuclide is compared to the parent. If the parent is short lived compared to the daughter, then there is no equilibrium at all. If the parent and daughter radionuclides are different elements, they can be separated chemically, and the daughter radioactivity can be obtained in high specific activity. Such a parent-daughter (mother-daughter) radionuclide pair is ideal to build a generator system to produce daughter radionuclide.
99mTc Generator
99Mo is one of the fission products of 235U fission in a nuclear reactor. It is known as fission moly and is produced with a very high specific activity compared to 99Mo produced by neutron activation. Most commercial generators are made with fission moly. The generator is based on a solid column method in which 5 to 10 g of preheated alumina (Al2O3) is loaded into a plastic or glass column. The 99Mo activity (2–32 Ci) in the form of molybdate ion ((MOO42−)) is adsorbed on the column. The column is thoroughly washed to remove undesirable contaminants. The amount of 99Mo activity on the column, along with the date and time of calibration (TOC), is provided for each generator. Commercial generators are sterilized and well shielded with lead or depleted uranium.
Once the generator is washed and calibrated, at time T0, there is no 99mTc activity on the column. As 99Mo (t½ = 66 hours) decays, 99mTc activity (t½ = 6 hours) is produced and builds up in the column as a function of time. Unlike molybdenum, technetium does not bind to alumina; it is immediately converted to 99mTc pertechnetate ion (99mTcO4−), which is the most stable chemical form of technetium, with an oxidation state of +7. Typically, >75% of 99mTc activity can be eluted with 3 to 10 mL of physiological saline solution.
Small amounts of 99Mo activity may occasionally break through (leakage or partial elution) from the column into the 99mTc pertechnetate solution. The maximum 99Mo contamination allowed is 0.15 μCi/mCi or 0.15 kBq/MBq of 99mTc pertechnetate solution at the time of elution. Each 99mTc dose to a patient should not contain > 5 μCi of 99Mo activity. As a chemical impurity, Al ion concentration should be < 10 to 20 μg/mL of eluent.
Both 99Mo (13%) and 99mTc decay to long-lived 99Tc, which, in turn, decays slowly by β-emission to stable 99Ru. Since 99mTc and 99Tc are isomers and chemically the same element, the presence of 99Tc may act as a carrier in the preparation of 99mTc radiotracers. When the generator is eluted once a day (every 24 hours), the number of 99mTc atoms is 27% of total 99Tc atoms. If the generator is eluted after 4 days, the number of 99mTc atoms is 5% of the total 99Tc atoms.
2.4 Preparation of Radiopharmaceuticals
2.4.1 General Methods of Radiolabeling
Depending on the type of radioisotope chosen and the method used to prepare a radiopharmaceutical, two different methods are generally used, as summarized in the following:
Isotope exchange: A radiopharmaceutical can be prepared by direct exchange (isotopic substitution) of one or more stable atoms of an element in a molecule with one or more nuclides of a radioisotope of the same element. The radiolabeled molecule and the unlabeled molecule are chemically identical and behave in vivo in a similar manner. This method of radiolabeling is generally used to prepare radioiodinated radiopharmaceuticals in which the stable 127I atom is replaced by a 123Ior131I atom.
Introduction of a foreign element, such as a radiometal: A radiopharmaceutical can be prepared by the introduction of a foreign element or radionuclide in a chemical compound. Most of the radiopharmaceuticals are prepared based on this method. This method involves attaching a radionuclide, such as 99mTc and 111In, to an organic molecule known as a chelating agent. One or more atoms (such as 16O, 14N, and 32S) in the chelating agent donate a pair of electrons to the radiometal atom to form coordinate covalent bonds. As a result, the chemical and biological properties of the radiometal–chelate complex are different compared to the chelating agent. Certain peptides and macromolecules, such as monoclonal antibodies, can be labeled with radiometals based on the metal chelation method described previously. This technique, however, requires conjugation of a bifunctional chelate (BFC) to the peptide or protein first, and then subsequent chelation of the radiometal by the BFC molecule. The radiometal is not directly incorporated into the peptide or protein molecule.
2.4.2 99mTc Radiopharmaceuticals
Chemistry of Tc-99m
99mTc is a second-row group VII transition metal and capable of multiple oxidation states (- 1 to + 7). In aqueous solution, the pertechnetate anion, 99mTcO4− is the most stable chemical species, with a + 7 oxidation state. Because its size and charge are similar to those of iodide (I‒), the in vivo distribution of pertechnetate is similar to that of the iodide ion. As pertechnetate is chemically stable and inert, it cannot bind directly to any organic molecule or chelate. Following reduction by appropriate reducing agents, 99mTc pertechnetate can be transformed into lower oxidation states that are chemically more reactive. Several reducing agents have been investigated, with stannous chloride (SnCl2) being the most widely employed for preparing complexes of Tc(V) and Tc(I), while boron hydrides are used to prepare organometallic Tc(I) complexes. During reduction by the stannous ion (Sn2+) in an appropriate buffer and pH, the presence of a ligand stabilizes Tc in its lower oxidation state. In a specific Tc complex, the oxidation state of Tc, however, depends on the chelate and pH. As a transition metal, Tc can adopt a large number of coordination geometries, depending on the donor atoms and the type of chelating agent. Several donor atoms, such as N, S, O, and P, geometrically arranged in a chelating molecule can form coordination complexes with technetium. A number of ligands (e.g., DTPA, MAG3, DMSA), iminodiacetic acid derivatives (e.g., diisopropylacetanilido iminodiacetic acid [DISIDA] and bromine-substituted acetanilido iminodiacetic acid [BrIDA]), and phosphates and phosphonates (e.g., PYP, MDP, and EHDP) have been labeled with 99mTc and routinely used for diagnostic imaging studies in nuclear medicine.
99mTc-labeled radiopharmaceuticals are generally prepared using cold lyophilized kits typically formulated with a coordinating ligand (or a chelating agent); a reducing agent (stannous chloride); and adjuvants, such as ancillary chelating agents, buffers, and antioxidants. The optimum formulation is determined for each specific type of cold kit.
99mTc pertechnetate eluted from a generator is used to prepare all other 99mTc-labeled radiopharmaceuticals. Preparation of certain 99mTc radiopharmaceuticals requires 99mTc pertechnetate containing minimal amounts of 99Tc atoms. It is important to remember that 99mTc is never “carrier free.” Eluting the generator twice, with the second elution made only a few hours after the first elution, will provide optimal labeling yields and a more favorable ratio of 99mTc to 99Tc.
Sodium Pertechnetate Tc-99 m Injection
99mTc is a sterile solution of 99mTc (and 99Tc) as sodium pertechnetate in 0.9% sodium chloride (normal saline) injection obtained by the elution of the 99Mo → 99mTc generator. The pertechnetate ion distributes in the body similarly to the iodide ion but is not organified when trapped in the thyroid gland. Pertechnetate tends to accumulate in intracranial lesions with excessive neovascularity or an altered blood-brain barrier. It also concentrates in the thyroid gland, salivary glands, gastric mucosa, and choroid plexus. However, in contrast to the iodide ion, the pertechnetate ion is released unchanged from the thyroid gland.
Tc-99 m Albumin Aggregated Injection (99mTc-MAA)
99mTc-MAA is a sterile aqueous suspension of 99mTc labeled to macroaggregates of human albumin. Macroaggregated albumin (MAA) cold kits contain lyophilized material of MAA particles, stannous chloride, and human albumin sealed under nitrogen. Approximately 90% of particles are in the range of 10 to 90 μm, and none can exceed 150 μm. Because the kit is designed as a multidose vial and contains 4 to 6 million particles, the appropriate amount of 99mTc pertechnetate must be added to the vial in order to have a single 3.0 mCi dose of 99mTc-MAA contain 200,000 to 500,000 particles. It is important to remember that the number of particles per dose will increase with time. The number of particles may need to be decreased for pediatric subjects and for those patients who have right-to-left cardiac shunts.
Within 1 to 5 minutes of intravenous injection, > 90% of 99mTc-MAA particles are trapped in the arterioles and capillaries of the lung by a purely mechanical process, which is a function of regional pulmonary blood flow. Particles < 10 μm are taken up by the reticuloendothelial system. Elimination of the labeled particles from the lungs occurs with a biological half-life of approximately 6 hours.
Tc-99 m Sulfur Colloid Injection (99mTc-SC)
99mTc-SC is a sterile colloidal dispersion of sulfur particles labeled with 99mTc pertechnetate and a cold kit containing three components:
A reaction vial containing a lyophilized mixture of 2.0 mg of sodium thiosulfate, 2.3 mg of disodium edetate (Al3 + chelator), and 18.1 mg of gelatin (protective colloid).
A solution A vial with 1.8 mL of 0.148 M HCl.
A solution B vial with 1.8 mL of a buffer containing anhydrous sodium phosphate (44.28 mg) and sodium hydroxide (14.22 mg).
After mixing the contents of the vial with 1 to 3 mL of 99mTc pertechnetate (~ 200 mCi) and hydrochloric acid, the mixture is heated at 100°C for 5 minutes. The vial is cooled and the buffer is added to the vial. During the reaction, elemental sulfur atoms are released to form colloid particles (< 1.0 μm). Gelatin provides a protective coating of the particles and controls the size of particles. Also during the reaction, 99mTc (nonreduced form) interacts with sulfur atoms, forming 99mTc-heptasulfide (Tc2S7). 99mTc-SC filtered through a 0.1- or 0.22-μm membrane filter is used in lymphoscintigraphy to localize the sentinel node in breast cancer and melanoma.
Following intravenous administration, 99mTc-SC is rapidly cleared by the reticuloendothelial system from the blood with a nominal clearance half-life of approximately 2.5 minutes. Approximately 80 to 90% of the injected colloidal particles are phagocytized by the Kupffer cells of the liver, 5 to 10% by the spleen, and the rest by the bone marrow.
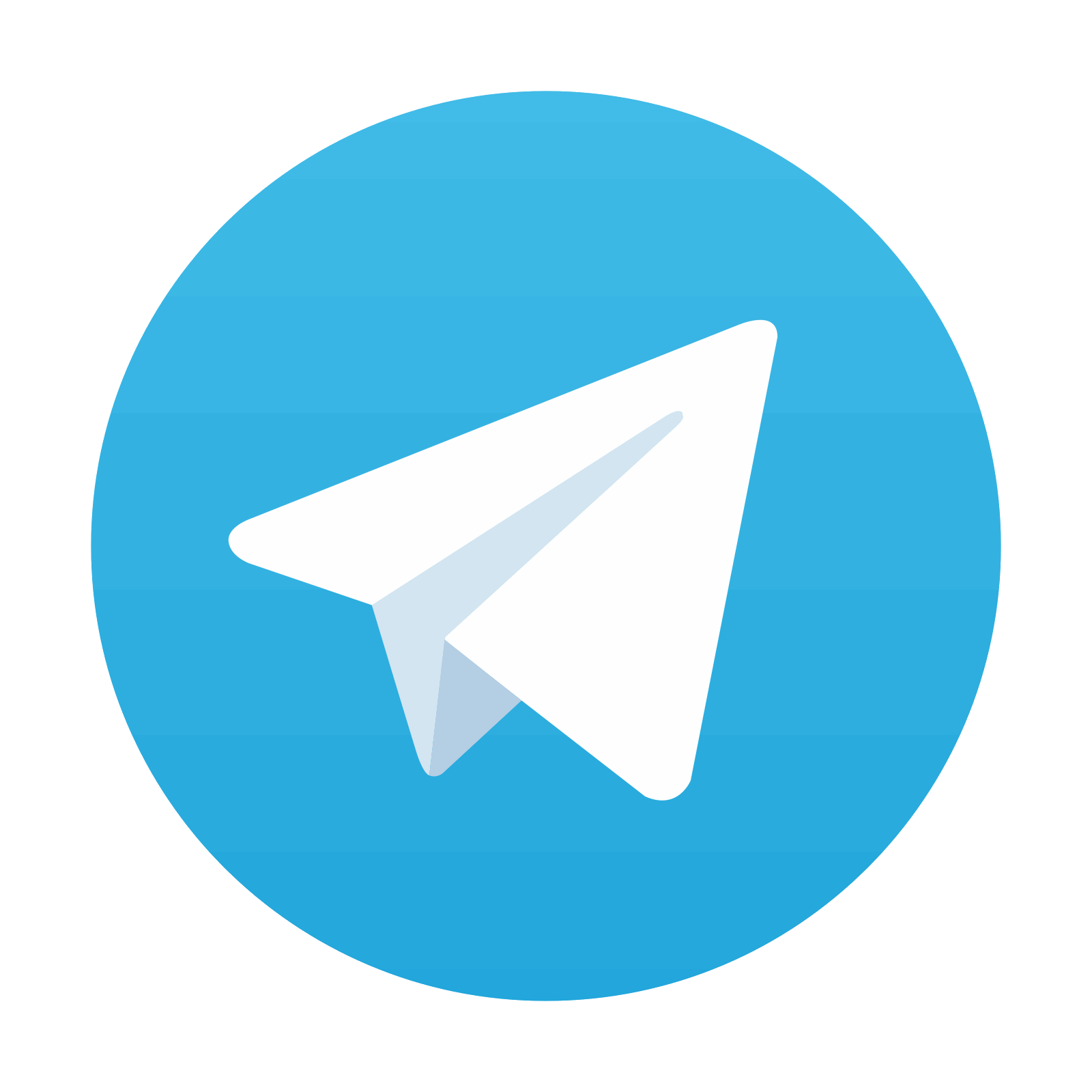
Stay updated, free articles. Join our Telegram channel

Full access? Get Clinical Tree
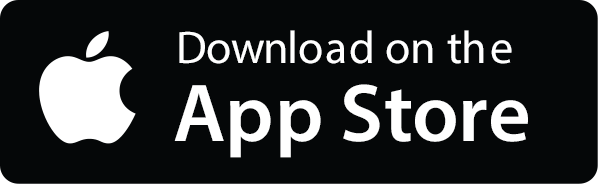
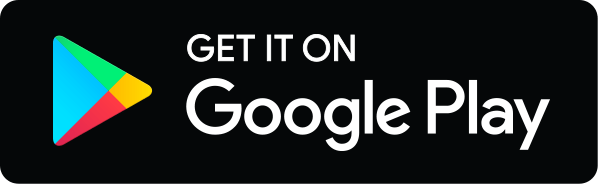
