3 Supratentorial Malformations
3.1 Introduction
Multiple malformations of development of the supratentorial parenchyma constitute some of the fundamental, as well as challenging and possibly confusing, aspects of pediatric neuroradiology. Understanding these entities requires some appreciation of embryology and genetics.
The mature cerebral cortex has six neuronal layers, which are formed through a complex developmental process. Cells originate within the germinal matrix of the cerebral cortex, which is located along the margins of the lateral ventricles (“neuronal proliferation”), after which time they move to the periphery (“neuronal migration”) and then form an organized cortex (“neuronal organization”). Abnormalities in each of these stages of cortical development can result in characteristic abnormalities. Although the sequence of proliferation, migration, and organization of cortical neurons is stepwise for a given neuron, each of these three processes will at most times and to some degree be taking place simultaneously with the others.
3.2 Malformations from Abnormalities in Neuronal Proliferation
Neuronal proliferation occurs early in gestation, with the three-part brain from week 4 differentiating into five secondary vesicles during week 6, with subsequent histogenesis. In the most simplistic manner of description, abnormalities in proliferation can result in too many cells, too few cells, or abnormal cells.
The prototype abnormality with the occurrence of too many cells is hemimegalencephaly, a condition in which there is hamartomatous/dysplastic overgrowth of an entire cerebral hemisphere (Fig. 3.1). The lateral ventricle in the abnormal hemisphere is typically larger than the ventricle in the contralateral hemisphere, and the abnormal hemisphere has altered blood flow resulting in accelerated myelination. Other abnormalities, including heterotopia and polymicrogyria, can also occur in hemimegalencephaly. Hemimegalencephaly can present with intractable seizures, and may require functional hemispherectomy for seizure control. Although the overgrowth in the condition typically involves an entire hemisphere, subhemispheric variants can occur. Furthermore, because the areas of overgrowth have many cells that are dysplastic, hemimegalencephaly overlaps with cortical dysplasia.
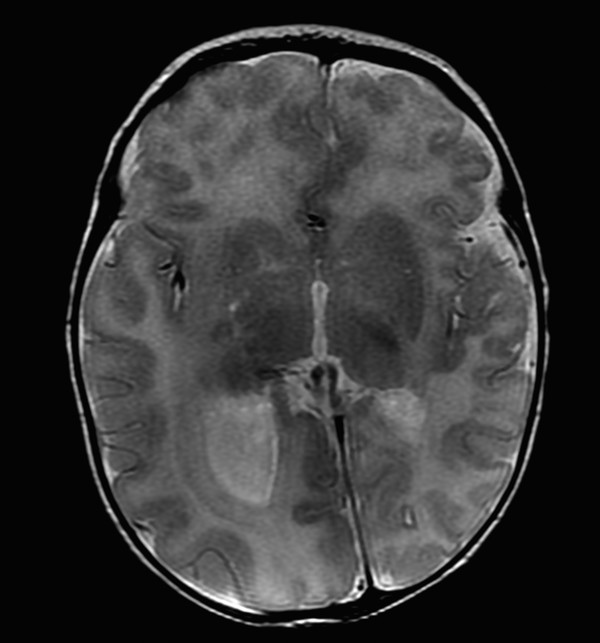
A focal area of proliferation of abnormal cells will result in cortical dysplasia. Focal cortical dysplasia (FCD) is divided into three types, each of which is further subdivided. Type I cortical dysplasia involves abnormal radial lamination (type IA), abnormal tangential lamination (type IB), or both (type IC). 1 Type II cortical dysplasia has dysmorphic neurons. 1, 2 Subtype IIB (also called Taylor-type dysplasia) has dysmorphic neurons with balloon cells, with a characteristic transmantle sign with a T2/fluid-attenuated inversion recovery (FLAIR) signal abnormality that tapers as it extends inward from the blurred cortex toward the superolateral margin of the lateral ventricles (Fig. 3.2). The abnormality is pathologicaly identical to that of the tubers in tuberous sclerosis complex. A less well-defined T2/FLAIR signal abnormality is seen in type IIA FCD. Although there are always exceptions, type I FCD shows up best on T1 W imaging and type II FCD shows up on T2 W and FLAIR images. Type III cortical dysplasia is associated with another lesion, which can be hippocampal sclerosis (IIIA), a tumor (IIIB), a vascular injury (IIIC), or an early-acquired parenchymal injury (IIID).
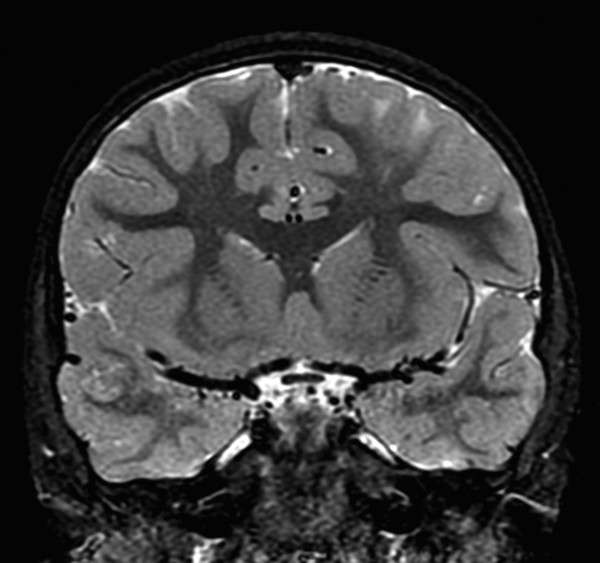
3.3 Malformations of Neuronal Migration
If a developing neuron fails to migrate appropriately to the periphery of the brain, the result is a heterotopic focus of gray matter. The area of heterotopia is most commonly seen as a nodular focus (or foci) of gray matter along the lateral margin of one of the lateral ventricles (Fig. 3.3), an entity known as periventricular nodular heterotopia (PVNH). This heterotopia is most common along the margins of the trigones of the lateral ventricles, and next most common in the anterior body/frontal horns. The lesions of PVNH can be multifocal and are commonly bilateral. Isolated PVNH is commonly an incidental finding, perhaps in patients with headaches or trauma, but the ectopic gray matter of PVNH can be the source of seizures. PVNHr is often best seen on T1 W imaging, although any imaging sequence may show abnormalities in contour along the margin of the lateral ventricles. Isolated foci of PVNH may be most conspicuous on DWI because it follows the diffusion characteristics of gray matter, in contrast to the adjacent white matter and CSF.
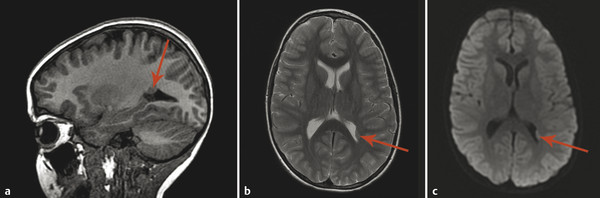
If the heterotopic gray matter of PVNH migrates partway toward the periphery of the brain, it will produce a visible subcortical deposit (Fig. 3.4). Subcortical heterotopia often occurs in conjunction with areas of PVNH. At times, multiple foci of subcortical heterotopia form a confluent to near-confluent path of gray matter from the ventricular margin to the periphery of the brain, representing transmantle heterotopia, which can be difficult to distinguish from a closed-lip schizencephalic cleft.
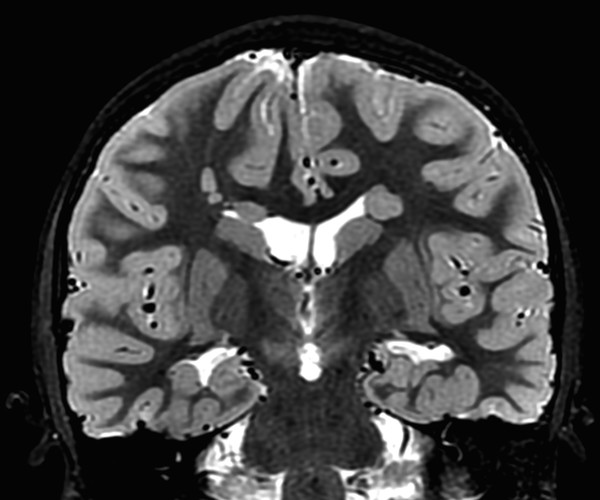
A continuous rim of heterotopic gray matter can sometimes be seen paralleling the cortex of the cerebral hemispheres, representing band-type heterotopia (Fig. 3.5). Band-type heterotopia is typically bilaterally symmetric and can involve the entirety of the cerebral hemispheres or only portions of them (most commonly, posteriorly if the heterotopia is incomplete). Band heterotopia can occur with lissencephaly-spectrum disorders (Fig. 3.6).
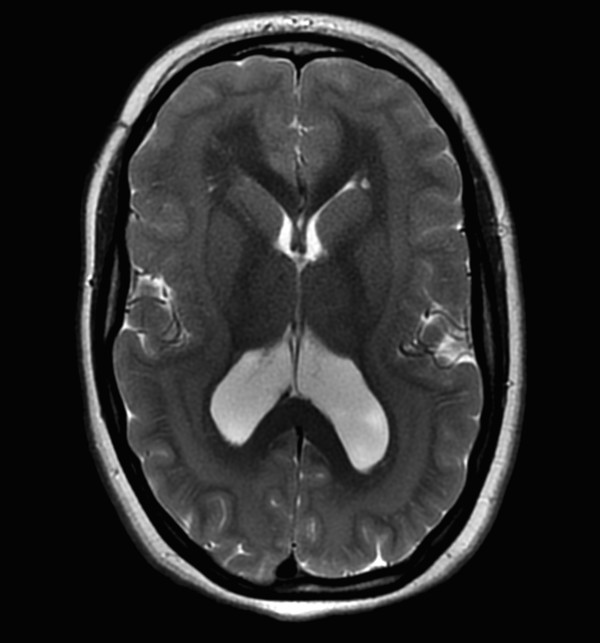
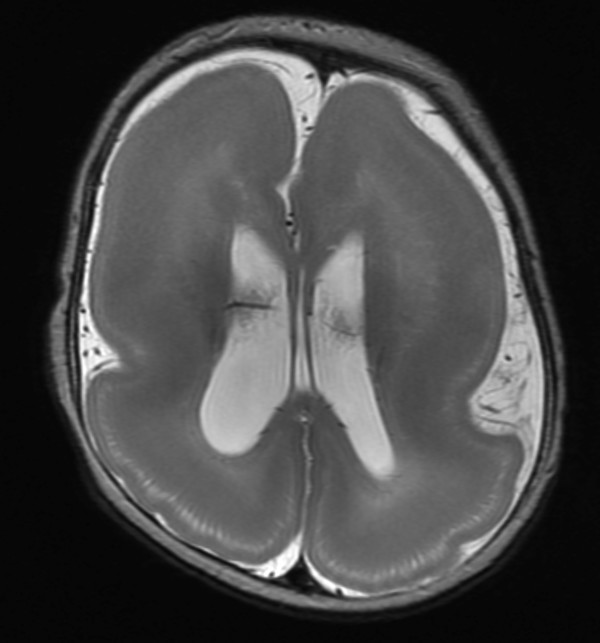
3.4 Malformations of Neuronal Organization
If developing neurons successfully migrate to the periphery of the brain, they must still appropriately organize into a six-layered cortex with a characteristic sulcation pattern. The signaling pathways guiding this organizational process can be impaired by any injury to the parenchyma, such as an in-utero infectious or ischemic process, or by genetic abnormalities. 3 If there is a peripheral parenchymal injury before the completion of neuronal migration and organization, the developing brain will be unable to form the appropriate pattern of gyration and sulcation. The resultant attempts at organization yield multiple smaller gyri (Fig. 3.7), whence the name polymicrogyria. Symmetric patterns of polymicrogyria tend to have genetic causes (Fig. 3.8), but some infections (including in-utero infections, such as with cytomegalovirus [CMV]) can result in bilateral polymicrogyria. Infectious etiologies of polymicrogyria may have associated dystrophic mineralization, with this particularly being true for toxoplasmosis/other/rubella/cytomegalovirus/herpes (TORCH) infections.
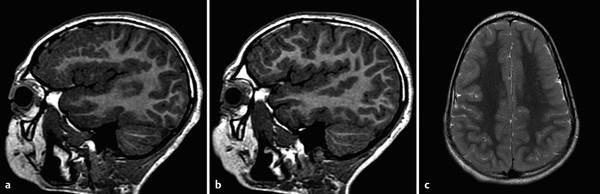
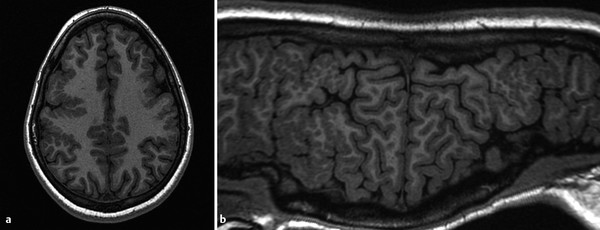
If a transmantle (from ventricle to periphery) parenchymal injury occurs before the completion of neuronal migration and organization, the margins of the parenchymal injury may be lined with gray matter. A cleft will form connecting the subarachnoid space with the ventricular system in an entity known as schizencephaly (Fig. 3.9). The schizencephalic cleft will commonly be lined with polymicrogyric tissue. If the transmantle injury that led to a cleft involved only a small amount of tissue, the margins of the cleft may be directly apposed, yielding the condition referred to as closed-lip schizencephaly. Closed-lip schizencephaly can be difficult to detect, and once detected can be difficult to differentiate from transmantle heterotopia, but there will commonly be a contour irregularity along the margin of the lateral ventricle, serving as a clue to the presence of a cleft. If a large area of parenchyma is injured, the margins of the schizencephalic cleft that are lined with gray matter are not in contact with one another, a condition called open-lip schizencephaly. At times radiologists agonize over whether a given defect is a closed-lip schizencepaly or an open-lip schizencephaly with a very narrow channel; this differentiation is arbitrary and probably has little clinical significance. A narrow channeled open-lip schizencephaly has a small amount of parenchymal injury similar to that of a closed-lip schizencephaly, and accordingly these entities are more similar in prognosis and extent of neurologic sequelae than are two patients each with an open-lip schizencephalic cleft, of which one is very narrow and the other is large. Schizencephalic clefts can be bilateral and do not have to be symmetric, so when a first cleft is detected, close attention is warranted for detecting a possible additional occult closed-lip schizencephaly. Schizencephaly is often an isolated finding, although it is seen with increased frequency in septo-optic dysplasia.
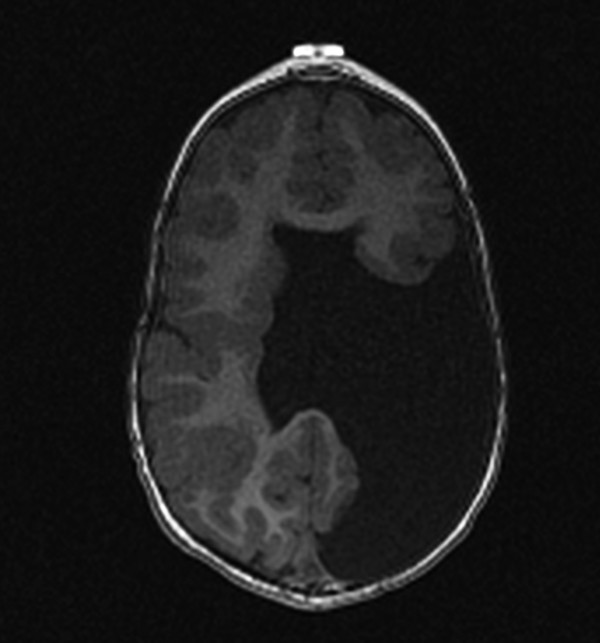
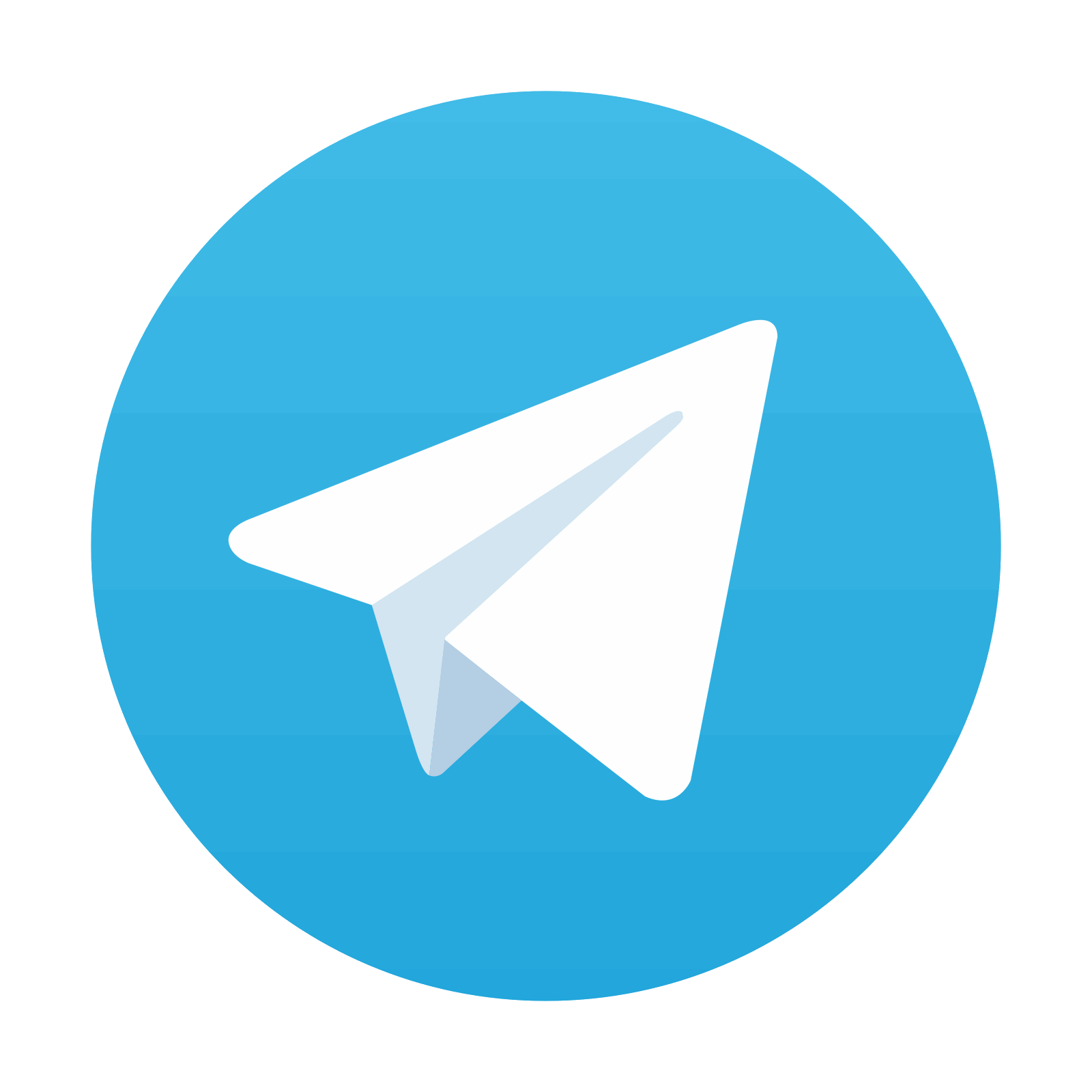
Stay updated, free articles. Join our Telegram channel

Full access? Get Clinical Tree
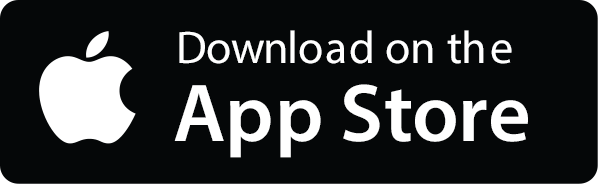
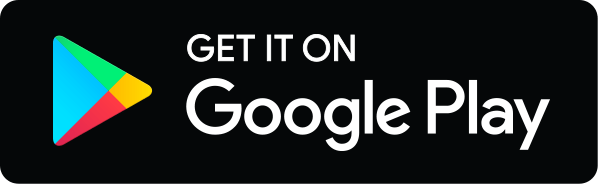