4 Irreversible Electroporation Ablation: Mechanism of Action and Devices
The process of electroporation dates as far back as the mid-1700s.1 Only recently, however, has the biomedical potential of irreversible electroporation been considered for various applications including tissue ablation. It was in the 1980s that the term electroporation was coined.1 Electroporation is a technique that uses an electric field to produce a transient disruption of the lipid bilayers of the cell membrane. Under the electric field, membranes rearrange themselves to form temporary pores through which intracellular contents can freely communicate with the extracellular environment.2 The electric field also provides a local driving force that propels larger or polar molecules and ions, which the membrane would normally be impermeable to, into the cell. This technique, reversible electroporation (RE), uses relatively low energies and has been used to introduce a variety of vectors into cells, ranging from DNA and viruses to macromolecules and enzymes.2 RE does not induce direct cell death; the alterations in membrane permeability are transient. Irreversible electroporation (IRE) is the use of the same concept to permanently create innumerable heterogeneous nanoscale pores in the cell membrane by exposing cells to notably higher electric energies.2 Conversely, the altered intracellular environment ultimately induces cell death via both apoptosis and coagulative necrosis.2 IRE has gained increasing attention in the field of interventional oncology as a new minimally invasive ablation technique. This technique is fast and easy to apply, does not require the use of adjuvant drugs, does not cause damage to the vasculature, and is not affected by local blood flow, making it a very specific method of tissue ablation with great potential for cancer therapy.
♦ The Science of Irreversible Electroporation
Although RE has emerged as a useful methodology in biomedical technology and in medicine over the past three decades, there has recently been a shift toward the use of IRE for tumor ablation. Similar to RE, in IRE a strong external electric field is applied to cells to increase transmembrane potential and induce the formation of permeable pores in the membrane. Permeabilization occurs only when the potential difference exceeds a critical threshold value that depends on cell type and is usually between 200 and 300 mV/cm.3,4 Unlike RE, however, the electric pulse strength and duration in IRE surpasses the threshold value to permanently damage the plasma membrane.5 This ultimately leads to cell death because the membrane is unable to return to its homeostatic status, and the nanoscale pores induced by the electric field are permanently opened.6
Cells are highly compartmentalized with a lipid bilayer plasma membrane that regulates intra- and extracellular solute transport. When an external direct current electric field is applied to a cell, it passes around the cell and not directly through it.7 This makes the intracellular current density less than the extracellular current density, creating a voltage potential difference across the plasma membrane of the cell.8,9 When the transmembrane potential hits a certain voltage threshold, the cell becomes unstable and the plasma membrane undergoes breakdown or structural rearrangement to create nanoscale pores in the membrane; thus, electroporation is observed.8,10 Once the membrane is permeabilized, the cell must put in an increasing amount of energy to maintain the transmembrane ionic concentration differences. Conductance of the plasma membrane shoots up in value and if the adenosine triphosphate-dependent protein ionic pumps are unable to compensate for the diffusion of ions through the pores in the plasma membrane, then the cell becomes energy depleted. The cell will then undergo biochemical arrest followed by cell death.8 The irreversibly permeabilized cells then remain in situ to be removed by the immune system.11
The precise mechanism of cell death in IRE-induced ablation is still under debate. However, both necrosis and apoptosis may play a role in IRE-induced cellular destruction.12,13 Recent data suggest that increased apoptotic markers may be found in post-IRE ablated tissue.12 Apoptosis induces innate cellular regeneration and requires less time for tissue healing and recovery than does necrosis. Additionally, apoptosis causes less fibrosis in the ablated area than does necrosis, which would help prevent further damage to ablated organs. Further studies are needed to elucidate the precise mechanism of cell death in IRE-induced ablation to advance our understanding of IRE and its capabilities.
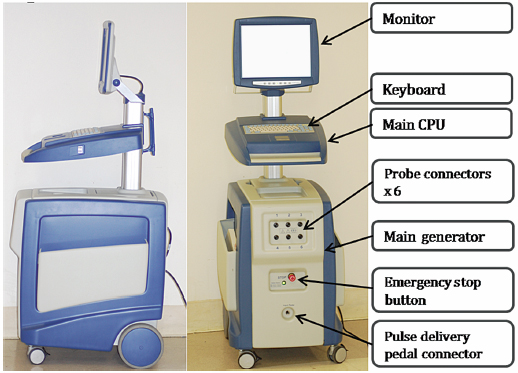
♦ Ablation Systems
Ablation Equipment and Generator Setup
This protocol is based on using NanoKnife® generators ( Fig. 4.1 ) and probes (AngioDynamics Inc., Queensbury, New York). There are two main types of IRE probes: monopolar and bipolar. The monopolar system ( Fig. 4.2 ) requires placement of two probes into or around the target. The electric current travels between the tips of the two probes to create an electric field, which results in ablation. Each monopolar probe is a 19-gauge needle with a maximum depth of penetration of 15 cm. A 1.5-cm spacing between the two probes with 2500 V results in approximately a 2 × 3 × 3–cm ablation zone. Up to six monopolar probes can be placed simultaneously, allowing for the development of overlapping treatment zones, which can result in a much larger total ablation area. The bipolar system ( Fig. 4.3 ) requires the placement of a single probe. It contains two poles within its distal portion, which creates IRE ablation. The bipolar probe is a 16-gauge needle with a maximum depth of penetration of 18 cm. With 2500 V, it will create approximately a 2 × 2 × 3–cm ablation zone.
The following brief steps are used to set up the IRE/ NanoKnife Generator for any typical solid tumor ablation:
Boot-up the system. Only the Microsoft Windows XP operating system platform is available at this time. Complete self-testing, including initialization, test charge, and test delivery, is required. If any of these self-testing steps fails, the generator will not function.
Enter the subject/patient data. The ID number is absolutely required for a log purpose. This automatically generated log is very useful in troubleshooting any technical failure with the generator.
Enter the probe selection: monopolar versus bipolar versus multiple monopolar.
Enter the treatment parameters (pulse parameters):
Number of probes
Voltage (1500–3000 V/cm)
Pulse length
Number of pulses = 90 pulses
Delivery of test pulse
Delivery of IRE ablation
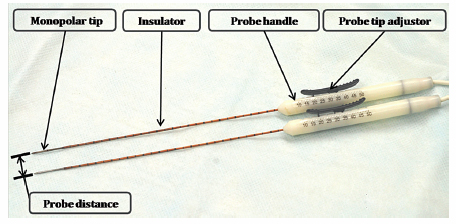
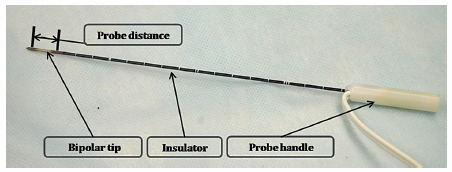
Ablation Protocols
Using the Lesion Estimator (from NanoKnife software version 2.1.0), the following pretreatment protocol can be planned: to ablate a 1-cm-diameter tumor, at least, a voltage of 2500 V/cm with 1-cm spacing between two monopolar probes is required ( Fig. 4.4 ). As can be seen in Figs. 4.5 and 4.6 , a smaller voltage or large distance between the probes can create a suboptimal ablation zone. This lesion estimator has been very accurate in predicting the actual lesion sizes in both preclinical and clinical studies. Essentially, any sized tumor can be planned in pretreatment and ablated using IRE, multiple probes, and the IRE lesion estimator.
Based on our studies and the manufacturer’s recommendation, we recommend using a minimum electric voltage of 1500 V/cm with at least a 1.7-cm probe distance and 90 pulses for a single ablation session. Depending on the number of pulses, the size of ablation will change accordingly: more pulses will create a larger ablation zone.
Figure 4.7 demonstrates an ablation protocol using a bipolar probe. With a single bipolar probe, it will create an ablation zone of 3.0 × 1.5 × 1.5 cm. To ablate a 1-cm-diameter tumor, it is optimal to place the bipolar probe in the center of the tumor and ablate it once. Any tumor size larger than 1 cm will require more than single ablation session or overlapping of probes.
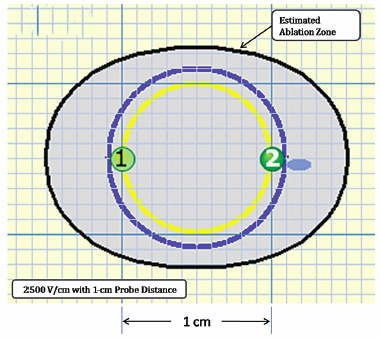
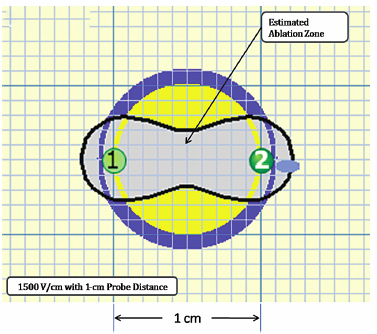
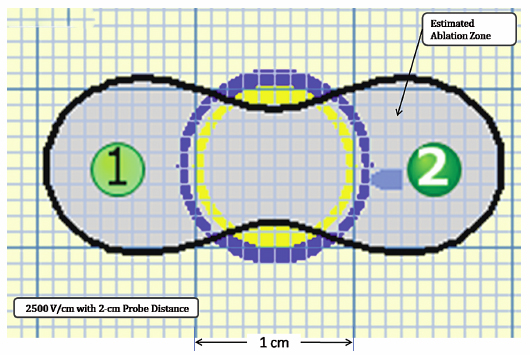
Figure 4.8 ) demonstrates that a maximum number of probes can be placed simultaneously. Six probes can be placed and ablated concurrently to create a large ablation zone. This ablation protocol will create an ablation zone of 3.0 × 4.7 × 3.2 cm. Using a conventional 90-pulse protocol, the ablation time for using this six-probe configuration is approximately 11 minutes. This is an optimal protocol with which to ablate a tumor with a diameter of approximately 3 cm.
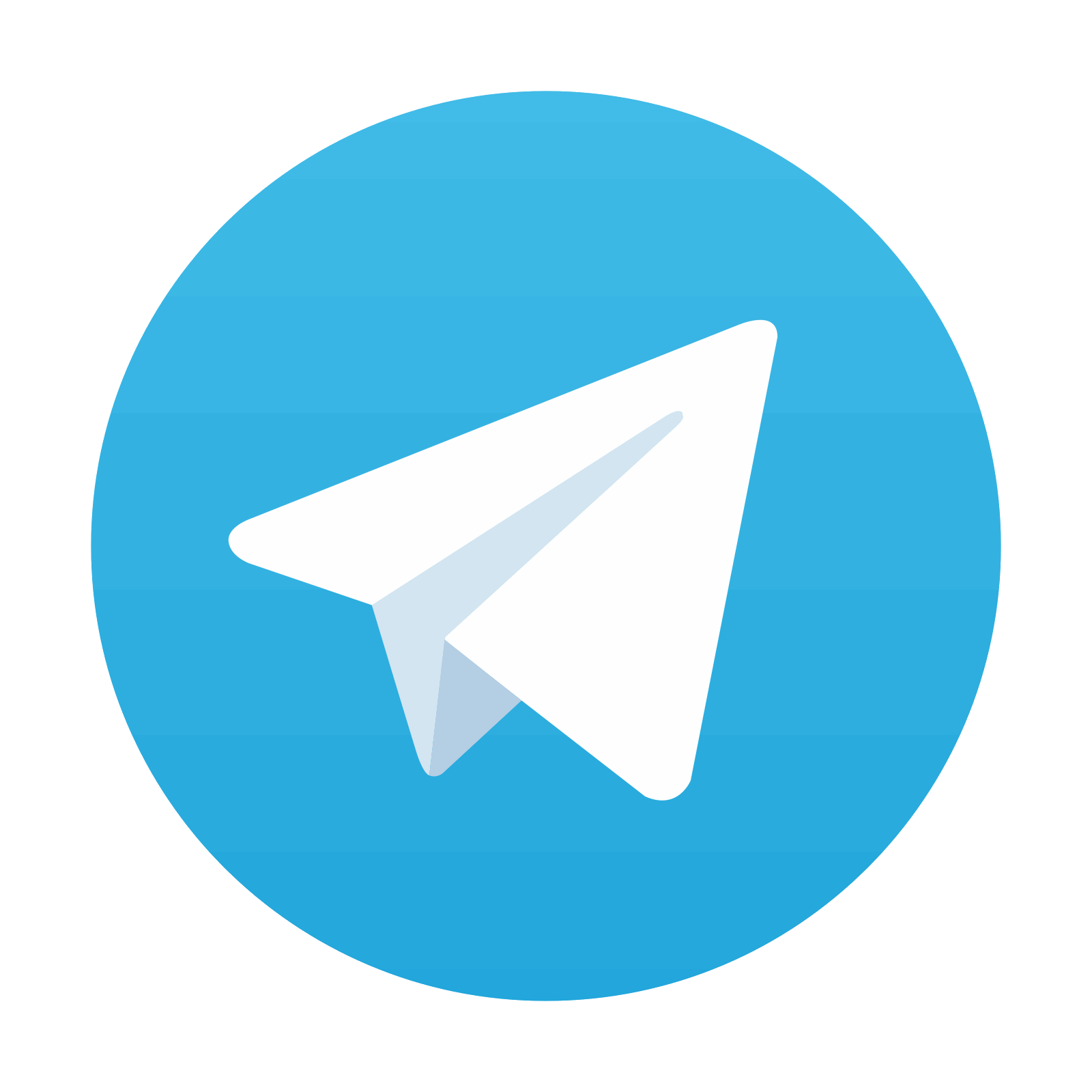
Stay updated, free articles. Join our Telegram channel

Full access? Get Clinical Tree
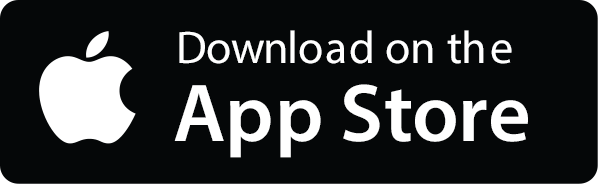
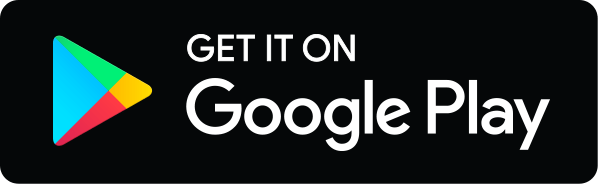
