© The Author(s) 2014
Yves Sucaet and Wim WaelputDigital PathologySpringerBriefs in Computer Science10.1007/978-3-319-08780-1_66. A Bright Future
(1)
Pathomation, Berchem, Belgium
Abstract
Digital pathology is an idea whose time has come. The hardware keeps getting better year after year, and enough companies now have invested in this new field to gather the critical mass necessary to turn digital pathology into a mainstream technique. But this is not enough. What remains is for digital pathology to significantly impact the way medical diagnoses are made and patients managed on a large scale.
Keywords
Digital pathologyMedical systems biology3D imagingMicro-CTSpectral imagingDigital pathology futureDigital pathology applications6.1 The 5 %/$2.4 Billion Challenge
At the 2013 Digital Pathology Association’s meeting in San Antonio, Texas, in an inspiring keynote presentation, Mark Priebe discussed the concept of a “5 %/$2.4 billion challenge.” The 5 % refers to the number of cases of misdiagnosis to be averted, and the $2.4 billion the cost savings such an accomplishment could achieve.
More specifically, because roughly 1.6 million pathology cases are reviewed every year in the USA, the 5 % translates into 80,000 cases. This 5 % is a conservative estimate of the percentage of pathology cases for which a major misdiagnosis occurs upon first review.
It is well known that many cases exist in which a diagnosis made in one pathology department is not corroborated by another. Swapp et al. [1] in their review of almost 72,000 (71,811) cases assessed at two centers found that there was a major disagreement in the diagnosis in 457 cases, resulting in some change in the management approach, representing 0.6 % of the total. Other estimates of major disagreement have been higher to much higher. In fact, across a host of other studies conducted over the past two decades, estimates of major discrepancies with second opinions have ranged from 1.3 % to as high as 30 %, averaging roughly 10 %. Such changes in diagnosis or disease-stage estimations have not only clinical, but societal cost implications, especially when there is some time lag between the first and second pathology assessments. For example, with cancers, the cost of a change in diagnosis increases as the stage of tumor increases, and the average cost of any change in diagnosis is $30,000 [2, 3]. That $30,000 per case, multiplied by 80,000 cases (conservatively reducing the 10 % mean estimate of major discrepancies with second opinions to 5 % of all cases), accounts for $2.4 billion wasted healthcare expenses, hence Priebe’s 5 %/$2.4 billion challenge.
Five percent—or 80,000 US cases per year representing 2.4 B US dollars in wasted healthcare costs—is a large enough gap to warrant our attention. Note that production = cases = patients = people. The goal is to close the significant gap that currently exists between best demonstrated practices and common practice in the accuracy of diagnoses within anatomic pathology. Enabling technologies like WSI provides a confidential, cost-effective, user-friendly means by which to facilitate external peer reviews and help close this gap.
6.2 New Frontiers
As stated earlier, as far as digital pathology has come over the past 10–15 years, and as many new inroads, it has made, what we have seen so far is, almost certainly, just scratching the surface. Just like photocopiers have recently been developed that not only copy 3-dimensional hard structures, like functional tools, but “print” them half a world away or potentially at an off-world space station [4], something most could not even have envisioned a few years ago, there are so many areas in which digital pathology may revolutionize the practice of medicine and beyond. Below is a brief look at some ground-breaking areas and fields in which digital pathology has already taken a prominent role.
6.2.1 Medical Systems Biology
The emergence of digital whole slide imaging and analysis is not the only way in which the fields of histopathology and pathology are changing. Another area in which digital pathology, including digital imaging and analysis, is demonstrating increasing value is in the very new but rapidly emerging field of medical systems biology. Largely since the year 2000, systems biology has emerged an inter-disciplinary field of study that focuses on complex interactions within biological systems, using a holistic approach to biological and biomedical research. One of the primary objectives of systems biology is to model and discover how cells, tissues, and organisms function as a system, including the workings of metabolic and cell signaling networks [5], rather than studying them at a given fixed moment in time, as is traditional under a standard microscope. It then makes heavy use of mathematical and computational models to generate a better and more systematic understanding of the interactions between various bodily systems.
One recent example of how digital pathology has facilitated this process has been using 3-dimensional tissue cultures, combined with semiautomated standardized histology and automatic slide imaging and analysis to study wound healing. To accomplish this, in their study of re-epithelialization following a punch biopsy, Safferling et al. [6] created a technical analysis pipeline of 3-dimensional organotypic wound models, standardized immunohistology, fluorescent whole slide imaging, image analysis, multiplex protein analytics, and computational systems biological modeling. Ninety-two 3D full-thickness skin-wound models, consisting of keratinocytes and fibroblasts, were tracked in time via two-step time-lag fluorescence staining. This allowed the investigators to visualize epidermal wound-healing spatiotemporally and in three dimensions, specifically assessing cell proliferation, migration, and differentiation, so as to derive a consistent theory about how these three processes are intertwined.
6.2.2 Three-Dimensional WSI
Three-dimensional whole slide imaging is another rapidly emerging field of pathology. As a gross over-simplification, 3D imaging is accomplished by stacking multiple focus planes. Advantages over traditionally paraffin-embedded 3D sections are numerous, but include being much simpler and less time-consuming. Advantages of 3D over 2D imaging are that it allows for better delineation of the spatial distributions and structural interactions of various tissue elements [7]. WSI technologies and rendering software have now improved to the point that 3D reconstruction of large structures at a microscopic scale from hundreds of serial sections is now possible. However, there are numerous challenges to this, which include dealing with section registration, tissue quality, the effects of tissue processing and sectioning, and the huge amount of data that can be generated [8]. However, processing complex tissues and managing huge datasets is something that pioneers in the digital pathology field have been improving at steadily. And the potential benefits of 3D digital imaging, in terms of understanding and classifying disease, are too great to be ignored.
In one just-published study, for example, Onozato et al. [9] attempted to better define histological subtypes of lung adenocarcinoma by studying four formalin-fixed human lung cancer resections. After paraffin-embedded tissues were sectioned using a Kurabo-Automated tissue sectioning machine, serial sections were automatically stained and scanned with a whole slide imaging system. The resulting image stacks were 3D-reconstructed using Pannoramic Viewer software (http://www.3dhistech.com). On inspection, the investigators found that two of the four specimens contained islands of tumor cells detached in alveolar spaces, a finding that had not been described in any preexisting adenocarcinoma classifications. Moreover, these tumor cell islands extended deep into the tissue, interconnected with each other and with the main tumor via a solid pattern that was surrounded by the islands. These findings caused the investigators to question whether the islands of tumor cells should be classified into a solid pattern within the current classification scheme, a change that could have prognostic implications [10].
In another example of the benefits of 3D over 2D WSI, it may be that certain low-power morphologic features that help distinguish benign from malignant follicular lymphoid proliferations may be enhanced by 3D analysis [8]. Three-dimensional reconstructions from WSIs also would facilitate estimates of coronary vessel narrowing, since 2D sections do not indicate the angle of the vessel relative to the section plane [8].
6.2.3 Spectral Imaging
What spectral imaging can offer over conventional methods is improved detection, validation, separation, and quantitation [11]. For example, a researcher can record the spectrum of auto-fluorescence and the spectrum of his desired signal or stain. With this spectral information, an image can be separated to reveal any auto-fluorescence, and false-positives can be prevented.
Spectral imaging can be divided into multispectral imaging, hyper-spectral imaging, and ultra-spectral imaging, according to its spectral resolution, and the number, width, and contiguousness of bands [12]. This technique can acquire reflectance, absorption, or fluorescence spectra for each pixel in the image, which can be used to detect biological and pathological changes in tissues that cannot be identified with traditional gray- or color-imaging methods [12].
A spectral imaging system has four main parts: collection optics or instruments; a spectral dispersion element; a detector; and a system control and data collection module [12]. The spectral dispersion element (a spectrometer, a filter, or an interferometer) is the heart of the system that enables separation of the light into different wavelengths [12
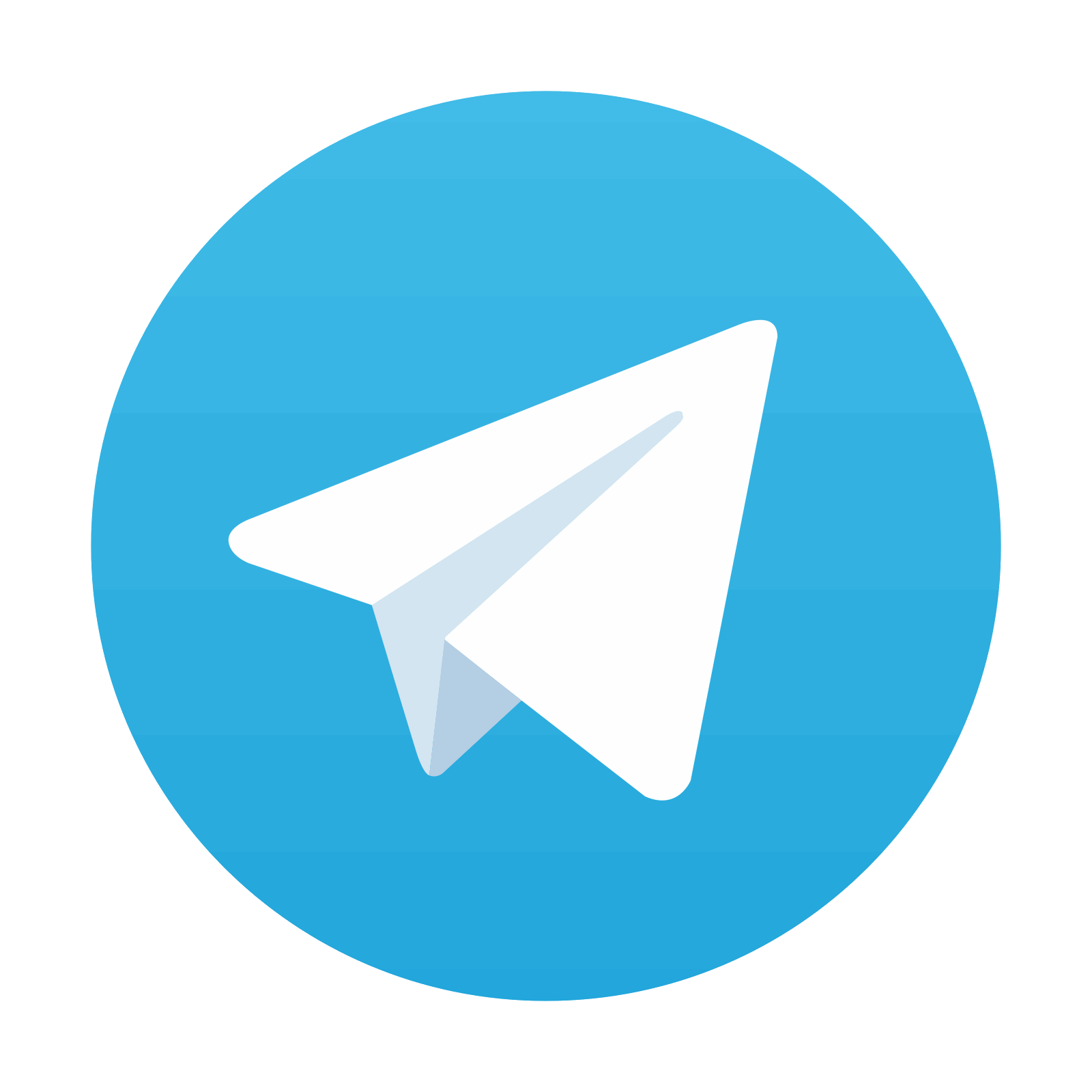
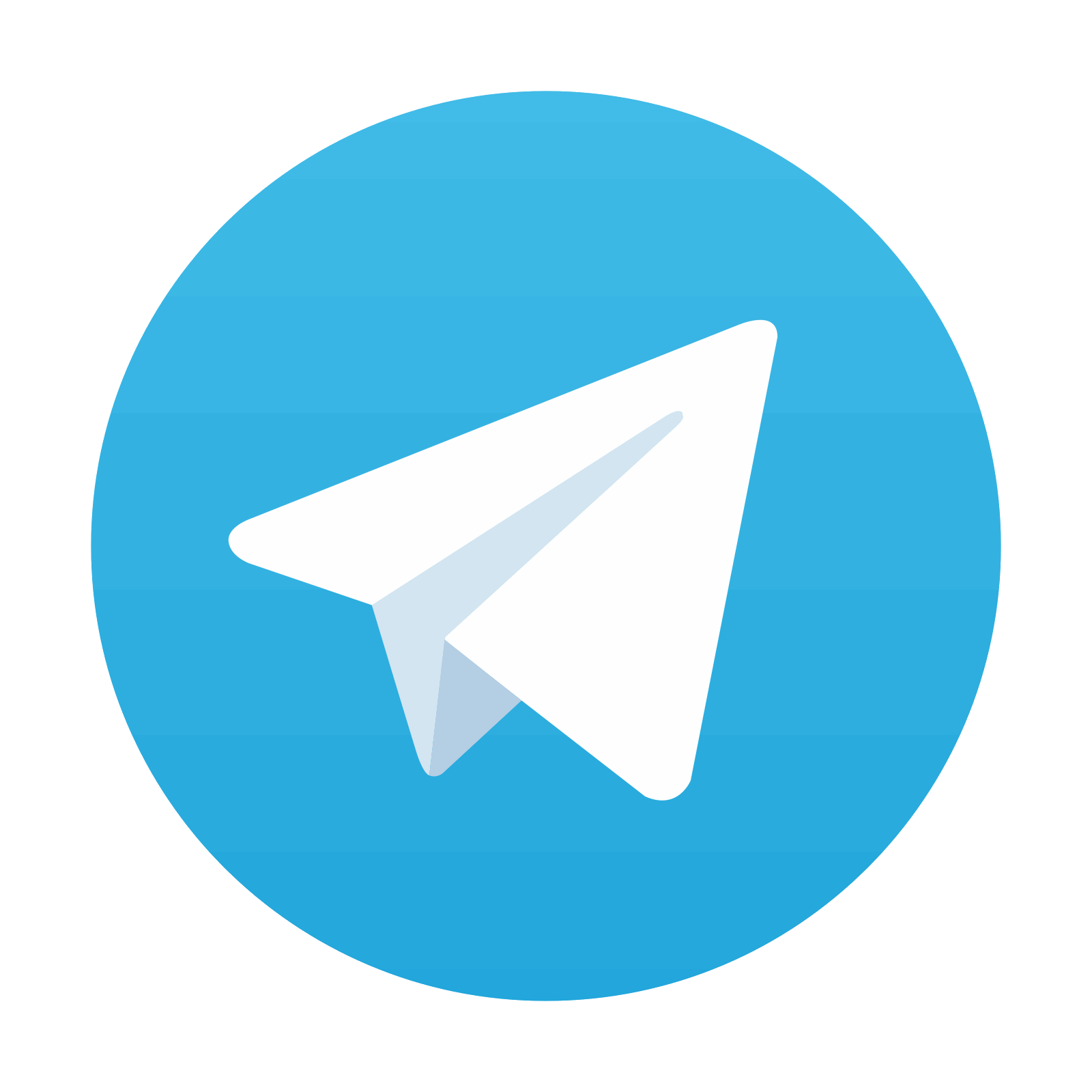
Stay updated, free articles. Join our Telegram channel

Full access? Get Clinical Tree
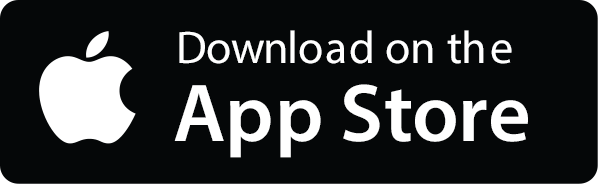
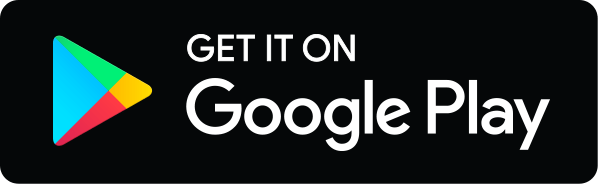
