Figure 17.1 Normal scalp anatomy. Anatomic representation illustrating the various anatomic layers of the scalp, using the mnemonic SCALP (Skin, Connective subcutaneous tissue, Aponeurotica, Loose avascular connective tissue, Pericranium). (Reprinted with permission from AMIRSYS Publishing, Inc.)
Cranium
The cranium, or skull, consists of the cranial vault and skull base (Fig. 17.2) (4–8). The cranial vault is formed from membranous ossification and is made up of bony plates, each of which is separated from the other by sutures and fontanels of connective tissue. The calvaria include an inner table, an intermediate diploic space and an outer table; the calvarial bones do not become separate plates until late infancy. The inner table grows only in response to brain growth, whereas the outer table primarily responds to external forces (e.g., molding, postural factors). The shape of the vault is determined by development of the cerebrum and by external factors. The sutures are remnants of the original membranous cerebral capsule and permit progressive ossification by direct osteoblastic activity during expansile brain growth (4, 6). The inner table is reshaped by the osteoclastic–osteoblastic cycle. The bony edges of the sutures are tapered with relatively straight inner table margins. Outer table interdigitations develop beyond the neonatal period. The Mendosal suture is closed physiologically in 33% of infants by 3 months of age and in 100% by 9 months. The Mendosal suture normally fuses prior to birth (around 14–18 weeks’ gestation) or in the first months of life. Roughly 16% of adults have been shown to have a persistent Mendosal suture (9). The others persist into late adolescence or early adulthood and usually are inconspicuous radiographically. Fontanels are broader areas of connective tissue located at the junctions of the major and minor sutures (4–6, 8). The anterior fontanel closes by 2 years of age; the smaller posterior fontanel closes by 3–6 months. The paired anterolateral fontanels close at about three months, whereas the posterolateral fontanels persist until two years of age. As the cranial circumference enlarges, the mean thicknesses of the calvarium and dura increase (10).
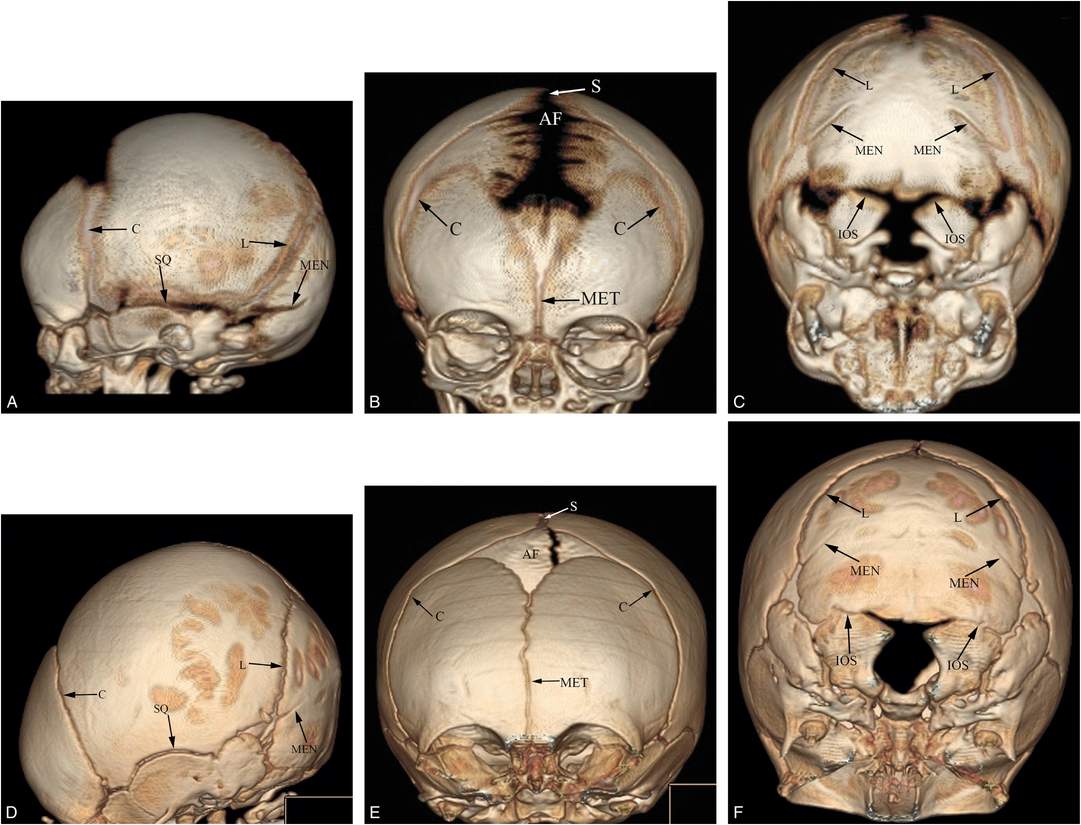
Figure 17.2 Normal cranial anatomy. Three-dimensional CT models of the skull viewed from the left side, front and base in one-month-old (A–C) and three-month-old infants (D–F). The principal sutures include the sagittal (S), coronal (C), lambdoid (L), squamosal (SQ), metopic (MET), and mendosal (MEN). Also note the interoccipital synchondroses (IOS) between the paired exoccipital and supraoccipital bones. AF = anterior fontanel.
The skull base is developed by endochondral ossification and forms the floor for the cerebrum, brainstem, and cerebellum (4–6, 11, 12). Growth of the chondrocranium parallels that of the remainder of the skeleton and is relatively independent of brain growth. Synchondroses are cartilaginous tissues that separate the endochondral bones of the skull base. All synchondroses close in early childhood except for the spheno-occipital synchondrosis (14 years of age), situated between the sphenoid body and the basiocciput (11, 12). This suture usually closes from lateral to medial in adolescence, earlier in females than in males.
There is rapid infantile brain growth, with corresponding calvarial enlargement, from 25% of adult brain weight at birth to 50% by 8 months, 75% at 2.5 years, 90% by 7 years, and nearly 100% by 12 years of age (6, 13). At birth, the cranium dominates over the face and the neck, not only because of the rapid early brain growth, but also because of the relatively late growth and maturation of the facial bones and cervical spinal column. The normal craniofacial ratio, as determined on a lateral skull film, a lateral computed tomography (CT) scout projection image, or a midsagittal magnetic resonance imaging (MRI), decreases with age. In the premature neonate it is 5:1 and in the term neonate 4:1; by 2 years of age it is 3:1 (6).
General considerations
Cranial injury constitutes an important component of the child abuse spectrum (7, 14–26). Silverman drew attention to Tardieu’s description of a skull fracture in an intentionally injured child reported in 1860, well before the first clinical radiograph was produced (7, 27, 28).
When pediatric skull fractures are studied in general, those due to abuse constitute a relatively small proportion (18, 21–37). Johnstone and associates studied 409 children in the Edinburgh area under 13 years of age admitted to the hospital after a head injury and found that only 3% of skull fractures were due to abuse (28). However, this percentage rises dramatically when the study group is limited to infants and toddlers. Hobbs found that 29 of 89 children (33%) under 2 years of age with skull fractures had suffered abuse (21). Leventhal and colleagues studied 93 skull fractures in children under 3 years of age, of whom 80% were less than 1 year old (29). They found that 27% were due to abuse. Meservy and colleagues studied 134 children under 2 years of age with skull fractures and found that 39 (29%) were due to abuse (30). In a systematic literature review between 1982 and 2000, Kemp and associates found that once major trauma was excluded, the probability of abuse in infants and toddlers with skull fracture was 0.3 (0.19–0.46) (32). This probability estimate was little changed in the same group’s recent review (38).
The prevalence of skull fractures in all cases of abuse varies widely depending on the study population (15, 16, 22, 25, 26, 39–41). Merten and others found 67 skull fractures in 712 abused children (15). A 10-year study of all hospitalized cases reported to the National Pediatric Trauma Registry between 1988 and 1997 found that 13.7% of abused trauma victims under 5 years of age had skull fractures versus 19.2% of the accidentally injured children (39). The mean age of the abused group was 12.8 months versus 27.5 months in the unintentionally injured children. In Loder and Bookout’s study of only abused children with fractures, in whom the average age was 16 months, the prevalence of skull fractures was 35% (25). Karmazyn and others found a prevalence of 22% (67/309) for skull fracture in children undergoing skeletal surveys (SSs) for suspected abuse (41). Rubin and associates studied a select group of 65 children under 2 years of age at high risk for abuse with normal neurologic exams at admission and found that 74% had skull fractures (40). Barber et al. found 138 skull injuries in 567 infants (24%) on SSs performed for suspected abuse. Twenty-six (19%) had unsuspected skeletal injuries (42).
When studies examine the relative frequencies of various inflicted fractures, the skull is found to be one of the most common sites of osseous injury, ranging from 7 to 30% of all fractures (14, 23, 25). Seventy-five of 899 (8.3%) fractures noted on SS by Karmazyn and associates involved the skull (41). Of the 931 fractures noted on 311 infant SSs by Barber et al., 138 injuries (15%) involved the skull (42). When only infant fatalities with skeletal injury are studied, as many as 41% of children have skull fractures, but because rib and metaphyseal injuries predominate, the percentage of fractures involving the skull is relatively small (7%) (24).
Despite the high prevalence of skull fractures with abuse, there is poor correlation between the presence of fracture and associated intracranial injury (ICI). In an early study employing CT and radiography, Tsai and associates identified skull fractures in 17 of 45 abused children (38%) with head injury (16). ICI was present in 65% of patients with skull fractures and 78% of those with normal skull radiographs. Zimmerman and colleagues studied 26 abused children with central nervous system (CNS) injury detected on CT and found only 6 skull fractures (23%) (17). Cohen and associates found that 11 of 37 abused children (30%) with head injury had skull fractures (19). Except for epidural hemorrhage (EDH) and brain laceration, which were noted only with skull fracture, the patients without fracture suffered the same range of intracranial injuries as those with fracture. Patients without fracture had a higher incidence of subarachnoid hemorrhage (SAH) (85%) than those with fracture (45%). In Merten and others’ study of 93 abused children with craniocerebral injury, skull fractures were identified in 56% of abused children with ICI (15). O’Neill and colleagues reported 32 patients with inflicted intracranial injuries and found 15 patients (47%) with demonstrable fractures (26). Subdural hematomas (SDHs) in 6 patients were associated with skull fracture; in contrast, 12 patients with SDHs had normal skull radiographs.
More recently, Fickenscher and others (43) reported on 58 children less than 20 months of age who underwent neuroimaging for possible abusive head trauma (AHT). Thirty-one subjects had evidence of abuse and 27 had accidental injuries. Seven of the 11 abused children with skull fractures were symptomatic. In a comprehensive review employing a US database, Leventhal and associates studied 18,822 children less than 36 months of age with traumatic brain injury (TBI) and/or fractures (44). They found that in the first year of life, isolated skull fractures without evidence of a TBI were almost always found to be the result of accidental falls. When infants presented with a TBI and skull fracture only, most cases were also attributed to an accidental fall. Importantly, they also found that in infants with TBI and no skull fracture, as well as in infants with TBI with skull fracture and other nonskull fractures, the cause was usually attributed to abuse.
Biomechanics
The biomechanics of skull fracture is driven primarily by the unique structure of the pediatric cranial vault and the material property changes in the cranial bone throughout early development. Infant cranial bone is composed of a single cortical layer that is much more flexible than adult bone and can deform eight times more than the cranial compact bone layer of an adult skull (45, 46). With age, the cranial bone not only gets thicker (Table 17.1), but the bone material also gets stronger and is able to withstand higher forces before fracture (45, 47–50). Interestingly, parietal bone material is significantly stronger and stiffer than occipital bone material (45). This means that given two bone samples of the same size from the parietal and occipital bones, the occipital bone would bend more and fracture earlier than the parietal bone. In an infant, however, the occipital bone is approximately 1.5 times thicker than the parietal bone (51). This increases the overall structural rigidity of the occipital bone and results in additional force required to bend the bone. A high structural rigidity with a low fracture tolerance suggests that the occipital bone is likely to fracture before significant bending occurs. The thinner parietal bone with a higher fracture tolerance is likely to bend more before failure.
Parietal | ||||
---|---|---|---|---|
Age | Frontal (mm) | Anterior (mm) | Posterior (mm) | Occipital (mm) |
20–40 weeks gestation | 0.78 ± 0.20 (19)*# | 1.16 ± 0.40 (7)# | ||
Term (>40 weeks) – 6 months | 4.12 ± 0.43 (32)▪ | 0.96 ± 0.50 (39)#▪ | 1.14 ± 0.64 (36)^▪ | 1.32 ± 0.35 (37)#▪ |
7–11 months | 4.95 ± 0.35 (32)▪ | 0.76 ± 0.33 (35)#▪ | 1.83 ± 1.02 (37)^▪ | 1.54 ± 0.88 (34)#▪ |
1–2 years | 5.9 ± 0.51 (32)▪ | 1.84 ± 0.38 (32)▪ | 2.37 ± 0.88 (50)^▪ | 2.88 ± 0.34 (32)▪ |
3–5 years | 6.48 ± 0.81 (32)▪ | 3.16 ± 0.39 (32)▪ | 3.34 ± 0.87 (42)^▪ | 4.22 ± 0.50 (32)▪ |
6–7 years | 5.70 ± 1.43 (32)▪ | 3.68 ± 0.5 (32)▪ | 3.50 ± 1.10 (42)^▪ | 5.00 ± 0.53 (32)▪ |
8–11 years | 4.76 ± 1.13 (32)▪ | 4.31 ± 0.95 (53)^▪ | 5.63 ± 0.64 (32)▪ | |
12–15 years | 4.20 ± 0.76 (32)▪ | 4.89 ± 0.95 (53)^▪ | 6.14 ± 0.79 (32)▪ | |
16–18 years | 4.15 ± 0.8 (32)▪ | 5.29 ± 0.83 (34)^▪ | 6.40 ± 0.88 (32)▪ |
Superscript indicates references that contributed to the averages:
▪ Roche (1953),
* Kriewall et al. (1981),
^ Loder (1996),
# Coats and Margulies (2006).
Data in table compiled from (a) Roche AF. Increase in cranial thickness during growth. Hum Biol. 1953;25(2):81–92; (b) Kriewall TJ, McPherson GK, Tsai AC. Bending properties and ash content of fetal cranial bone. J Biomech. 1981;14(2):73–9; (c) Loder RT. Skull thickness and halo-pin placement in children: the effects of race, gender, and laterality. J Pediatr Orthop. 1996;16(3):340–3; (d) Coats B, Margulies SS. Material properties of human infant skull and suture at high rates. J Neurotrauma. 2006;23(8):1222–32.
Table reprinted with permission from Margulies SS, Coats B. Experimental injury biomechanics of the pediatric head and brain. In Crandall J, Myers B, Meaney D, Schmidtke S, eds. Pediatric Injury Biomechanics: Archive & Textbook. New York, NY: Springer; 2013, pp. 157–89.
One important component of the pediatric calvarium is the membranous suture that connects the bony plates. Cranial suture is able to widen to twice its size before failure, and failure is more likely to occur at the suture–bone junction rather than within the suture material itself (45). The combination of thin bone attached with this weak extensible material results in an infant skull that is highly deformable, especially with parietal impact (52). The material properties of cranial suture do not change with age (45), which means that the pediatric calvarium only gets stronger as cranial bone grows and replaces cranial suture.
Prediction of crack propagation in the skull is very difficult because it is highly dependent on the microstructure of the bone. One unique characteristic of the pediatric skull is the visible trabecular fibers that radiate outward from the ossification centers of each bony plate (Fig. 17.3). When a force is applied to the cranial bone along the direction of these trabeculae, the skull is four times stiffer than when the force is applied perpendicular to the trabeculae (53, 54). This means that infant cranial bone will bend more easily between the trabeculae rather than across the trabeculae. Thus, fracture caused from bone flexure is most likely to originate and run between the trabeculae rather than across them.
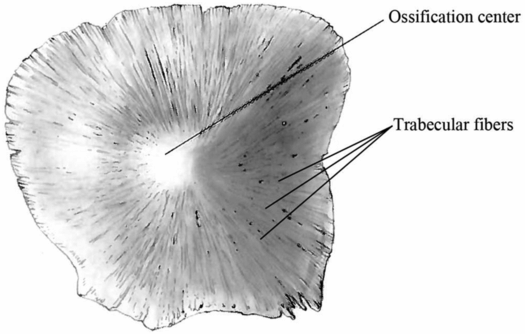
Figure 17.3 Infant skull trabecular fibers. Schematic of an infant parietal bone that illustrates the trabeculae radiating outward from the ossification center of the bone. These fibers are not present in adults and are important to understanding the biomechanics of skull fracture in infants. (Image modified with permission from Scheuer L, Black S. The Juvenile Skeleton. New York, NY: Elsevier Academic Press; 2004.)
Early work on adult skull fracture theorized that significant bending of bone could result in fracture lines that initiate at locations other than the site of impact and then travel back to the site of impact (55). This has been shown not to be the case for most adult skull fractures because no significant bending occurs before fracture (56, 57). Infant cranial bone, however, is much more pliable and may result in fracture away from the impact site. Weber’s series of infant cadaver experiments provides some evidence of this (58, 59). Infant cadavers were dropped 3 ft from a supine position onto various types of flooring. Ages of the cadavers ranged from newborn to nine months (median: three months). Each cadaver was dropped only once and fracture patterns were reported in each case. In several of the drops, two lines of fracture were noted (Fig. 17.4A–C). These two lines were often unilateral and ran parallel to each other. In one case, the fracture lines were biparietal, but not connected at the sagittal suture. This is highly suggestive of fracture resulting from flexure of the bone and indicates that fracture can occur in a location other than the impact site. Unfortunately, it is unclear from the study which impact sites on the head most commonly lead to these fracture patterns.
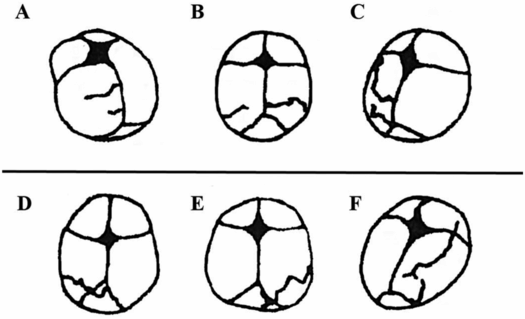
Figure 17.4 Infant cadaver fracture patterns. Infant cadavers (0–9 months old) were dropped from 3 ft onto various flooring surfaces. A–C, Three examples of multiple fractures from a single impact which indicate that flexure of the bone was likely the cause of at least one of the fractures or both. All infants in this set were 2–3 months old. Drops were onto a 2 cm foam mat (A), low-pile carpet over concrete (B), and foam backed linoleum (C). D–F, Three examples of fractures that crossed a suture and continued to propagate on a neighboring cranial bone. All infants in this set were 2–4 months old. Drops were onto harder surfaces such as low-pile carpet over concrete (D) or a stone tile (E, F). (With permission from Weber W. [Biomechanical fragility of the infant skull]. Z Rechtsmed. 1985;94(2):93–101.)
One other finding in this study was that 89.5% of the fracture lines began or ended at the suture; either scenario is not surprising. The maximum flexure of a bone often occurs at its boundaries (60) because it is thinner and less structurally rigid. Bending fracture is therefore most likely to initiate where the material is most easily bent. Similarly, if a fracture starts elsewhere and terminates at the suture, this too can be easily explained by the energy requirements of a fracture. Skull fractures initiate and elongate based on the energy transferred from an impact. As the fracture line propagates, the available energy decreases. When the fracture line reaches a cranial suture, more energy is required to overcome the new material and there is often not enough energy left at this point to continue. Traumatic events that have higher impact energy or are initiated closer to a suture, however, may have enough energy to cross the suture and continue on a neighboring cranial bone. Evidence of this can be seen in three cases reported by Weber (Fig. 17.4D–F). All three cases were dropped onto harder types of flooring (stone tile, low-pile carpet overlaying concrete), which transfer more energy to the skull than do softer materials. In addition to cranial suture, trabeculae and other heterogeneities in the bone require more energy to traverse. One last notable finding from the Weber study was that all the fracture lines appeared to run through the thinnest regions of the skull. It’s important to remember that fracture lines will always try to find the path with the least material resistance.
The most common type of skull fracture pattern from inflicted or accidental trauma in children is a linear fracture (61, 62). These simple fractures are typically isolated calvarial injuries. In contrast, complex fractures have multiple fracture initiation sites, traverse the trabeculae, and often occur in thicker parts of the skull. Thus, this type of injury requires more energy than a simple linear fracture. One exception to this is when the head is impacted while constrained by a hard surface. Kroman et al. investigated impact fracture patterns from weight drops onto adult cadaver heads that were either supported by a semi-rigid surface (wood board) or by a surface that broke once too much force was created (notched wood board) (57). With the breakable surface, only linear skull fractures were reported. However, when the cadaver heads were placed on the semirigid surface, the resulting fracture at the site of impact was much more complex and included multiple radiating and concentric fracture lines. A single linear fracture was found on the parietal bone contralateral to the impact site. In both scenarios, the energy going into the event was the same. This indicates that less impact force is required to create stellate fractures when the head is constrained compared to when it is unconstrained. An accidental fall is an example of an unconstrained head impact because the head is allowed to rebound following the impact. An object falling on a child’s head while that child is lying on the ground is an example of a constrained head impact.
Imaging patterns
Soft tissue injury
Patients with head injury frequently have associated hemorrhage or swelling of the scalp. The injury may involve the soft tissue layers and spaces, or potential spaces between these layers, resulting in subcutaneous hemorrhage/edema (caput succedaneum), subgaleal hemorrhage, or a subperiosteal hematoma (cephalohematoma) (1, 63). When fractures are present in young infants, there is often a subgaleal hematoma (Fig. 17.5). A substantial amount of blood may be lost into this potential space that can be life-threatening. However, the absence of such swelling seen clinically should not exclude the possibility of an underlying fracture, particularly if the injury was sustained shortly before medical evaluation. Also, early swelling or hematoma formation may hide beneath the hair and fatty soft tissues of the infant scalp, particularly in the occipital region.
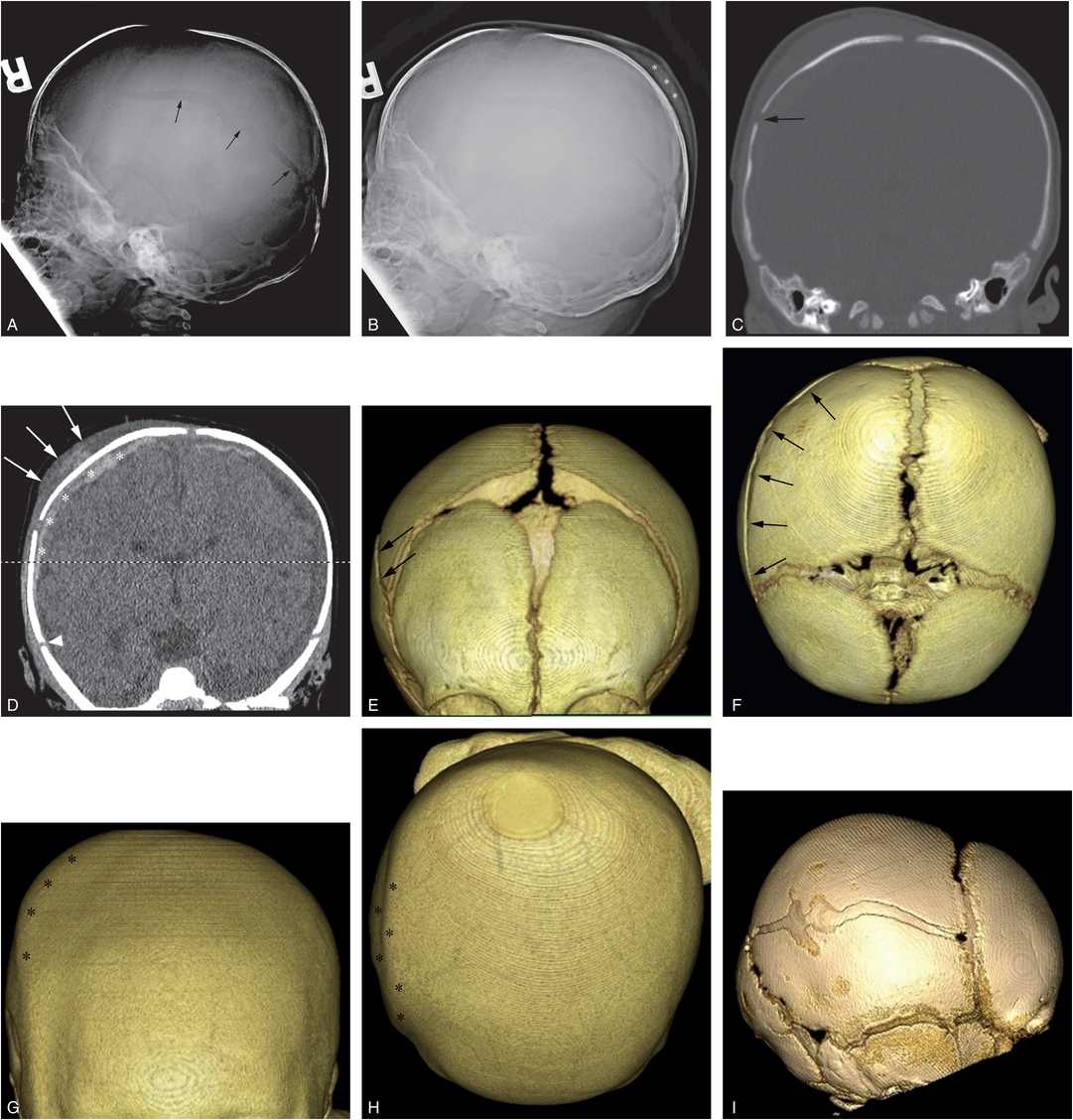
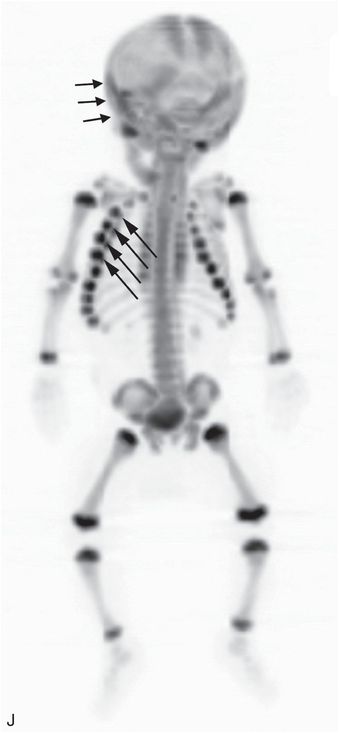
Figure 17.5 Subgaleal hematoma, skull fracture, and extra-axial blood. (See same patient in Fig. 5.34.) A two-month-old infant with seizures and multiple fractures. A, Lateral skull radiograph demonstrates a diastatic parietal skull fracture (arrows). B, The same image with contrast and brightness adjustments demonstrates posterior parietal scalp swelling (*). C, Coronal CT reformat with bone window settings shows the diastatic right parietal skull fracture (arrow). D, The same image with soft tissue settings shows high attenuation scalp swelling (arrows) lying predominantly between the fracture and the sagittal suture, although mild high attenuation soft tissue swelling extends to the right squamosal suture (arrowhead). Note extra-axial convexity blood (*). E–H, The 3D models with bone (E, F) and soft tissue (G, H) settings viewed from the front (E, G) and above (F, H) show the skull fracture (arrows) and the overlying soft tissue swelling (*). The 3D images with a surface display permit correlation of the scalp swelling with the physical examination. I, The 3D model viewed from the right depicts the complex diastatic right parietal fracture. J, The 18F-NaF PET MIP image shows increased tracer uptake in the right scalp and skull region (short arrows), a pattern evident with some, but not all skull fractures. Note the right-sided rib fractures (long arrows). A CML and a vertebral compression fracture were also noted on SS (not shown).
As expected, impact injuries are common in cases of fatal AHT. In a forensic autopsy study of 41 children less than 2 years of age, Brennan et al. found 85% had evidence of blunt head injury. Of these, 37% had skull fractures; 69% had galeal or subgaleal hematomas; and 54% had scalp bruising (64). Duhaime and colleagues found that most fatally abused infants with head injury and no clinical evidence of scalp swelling or bruising had scalp contusion at autopsy (65). It is notable that scalp hematoma tends to be less common in AHT than in accidental head injuries (6.7% vs. 49%) (66). The prospective 2010 study by Vinchon and others demonstrated that the absence of scalp swelling, SDH, and significant retinal hemorrhage (RH) were highly correlated with AHT (67); this is discussed in Chapter 18.
Subgaleal hematomas may be appreciated on skull radiographs; use of appropriate contrast and brightness settings and image presets are necessary to optimize detection of soft tissue swelling (Fig. 17.5). CT is well suited to the evaluation of hemorrhagic collections and edema of the scalp, especially if the swelling is asymmetric. Symmetric collections or edema, particularly if early, minimal, or resolving, may be more difficult to discern as an obvious abnormality unless the soft tissue planes are carefully examined. Window and level adjustment will often allow delineation of the soft tissues of the scalp, face, and neck. Higher Hounsfield attenuation values may indicate the presence of acute or subacute hemorrhage. Mixed attenuation values within a hematoma are commonly seen with acute injuries related to a variety of factors, and should not necessarily point to an older injury.
Focal soft tissue swelling may be difficult to identify, and proper slice selection can identify subtle regions of soft tissue asymmetry pointing to scalp injury (Fig. 17.6). Using the soft tissue surface display of three-dimensional (3D) models provides an image which correlates with the surface contour of the zone of scalp swelling (Fig. 17.5). Although soft tissue swelling usually corresponds to a site of blunt impact, swelling may occur remote from the contact site, particularly if a diastatic fracture or a traumatic suture diastasis propagates well beyond the margins of the impact zone and results in bleeding along the fracture margins. Despite the presence of bilateral skull fractures, swelling may be asymmetric and frequently it is impossible to determine whether there has been one massive impact, or multiple contact injuries. Although blunt injury accounts for most instances of scalp hematoma, similar hemorrhage can occur as a result of hair pulling. The hematoma is typically noted at the site of a large hair braid (68). It should be noted that scalp swelling is not always traumatic in origin. Thakur and Kaplan described an 18-month-old infant with recurrent scalp swelling and abuse was originally considered. Ultimately it was determined that the child had angioneurotic edema and was successfully treated with antihistamines (69). It must be emphasized that in contrast to scalp swelling, facial bruising is a strong predictor of abuse in nonambulatory infants (see below).
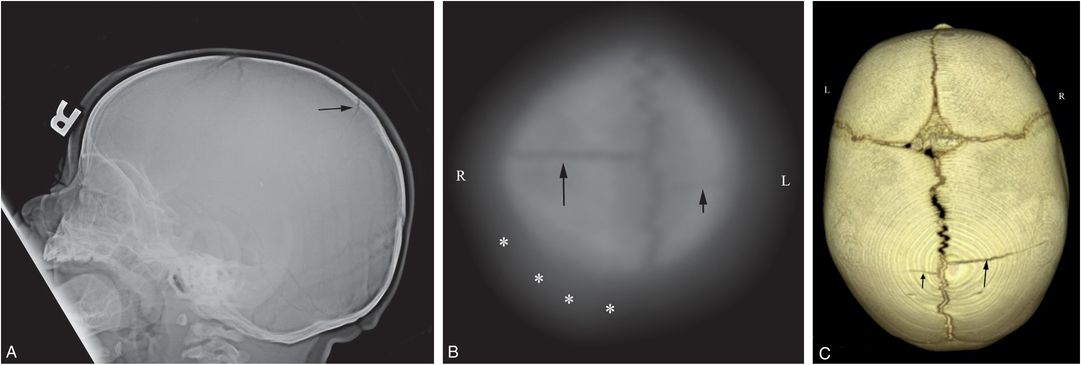
Figure 17.6 Subtle scalp injury overlying linear skull fracture. A 5-month-old, former 33-week gestation triplet with chest and abdominal bruising and bite marks. (See sibling in Fig. 12.16.) The father admitted to squeezing the infant’s chest several days earlier. SS demonstrated multiple rib fractures. A, The lateral view from the skull series shows a right parietal skull fracture (arrow). No soft tissue skull swelling is evident. High vertex axial CT section (B) and 3D model (C) viewed from above demonstrate asymmetric soft tissues (*) and a linear right parietal fracture (long arrow) extending to the sagittal suture. A second short linear lucency is evident in the left parietal vertex extending to the sagittal suture just posterior to the right-sided fracture (short arrow). It is impossible to determine whether this latter finding is a fracture or a normal parasagittal fissure.
Cranial injury
The imaging appearances of skull fractures may be classified into simple and complex categories. A simple, or linear, fracture consists of a single fracture line that appears to extend in straight, jagged, or slightly curved fashion, features that are dependent on a variety of technical factors. The fracture margins are separated by less than 3 mm. Such fractures are usually restricted to a single bone, but may cross a suture; typically biparietal fractures that cross the sagittal suture. In some instances, these fracture lines are not in direct continuity, but extend in close proximity to each other at the suture, suggesting that energy associated with one fracture is transmitted to the second via the interposed suture, or that flexure of both bones initiates the fractures. In such cases it can be impossible to determine whether the fractures have occurred with one or separate impacts solely on imaging grounds (Fig. 17.7). This type of injury is noted with increasing frequency with the liberal use of 3D CT cranial models, and the presence of this “wrap around” fracture does not preclude a single injury, even when there are apparently separate regions of soft tissue swelling over each fracture component (70–72).
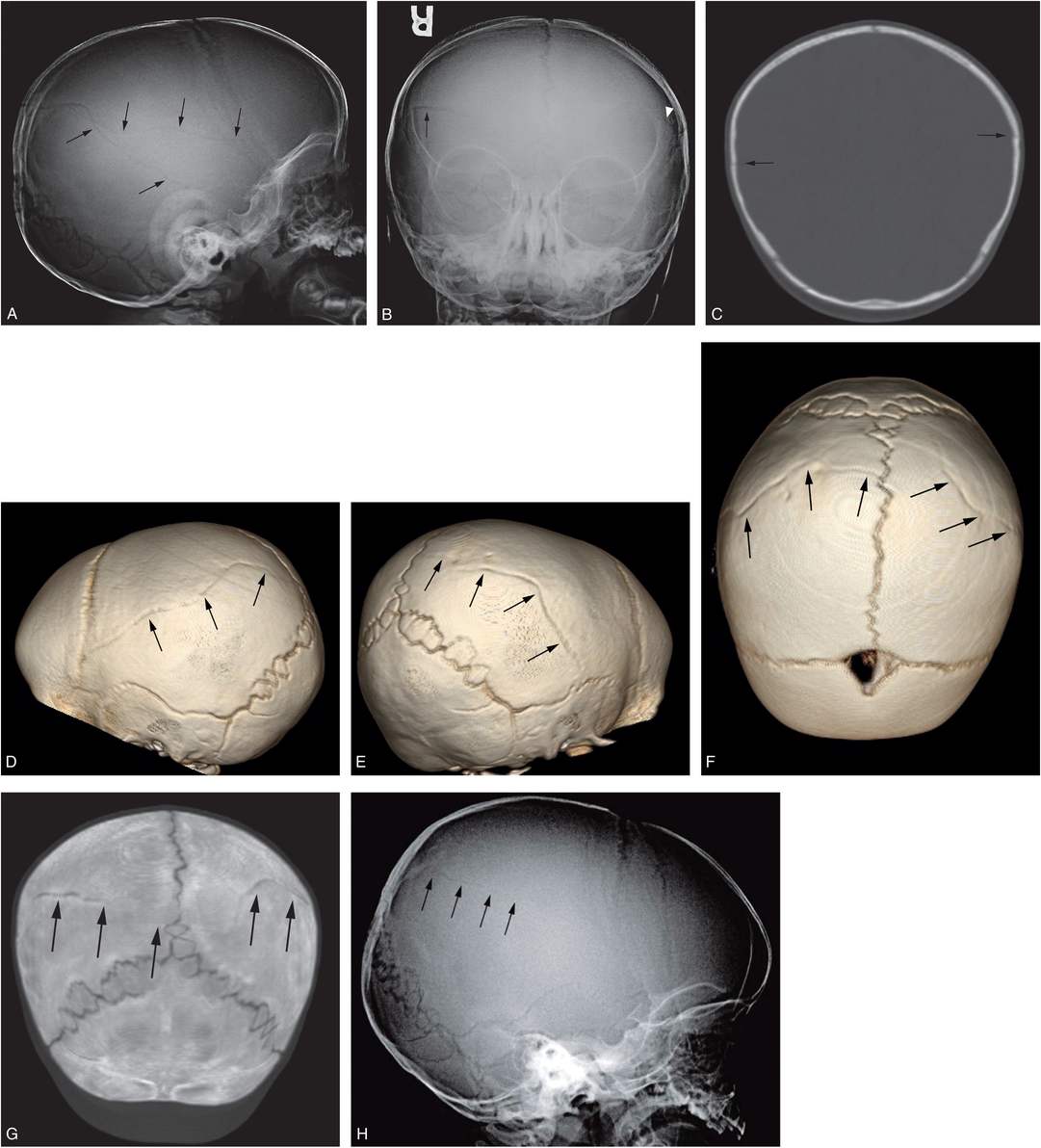
Figure 17.7 Biparietal noncommunicating skull fractures. A nine-month-old boy who initially presented at six months of age with unexplained scalp swelling. Skull radiographs were interpreted as normal and he was discharged home. He returned with unexplained knee swelling. SS showed metatarsal and metacarpal fractures. (See same patient in Figs. 3.99, 4.65.) A, Lateral view of the skull series demonstrates two parietal fractures (arrows), but the AP view (B) reveals only a right-sided fracture (arrow) and a questionable fracture on the left (arrowhead). C, Coronal reformat shows biparietal fractures (arrows). D–F, The 3D cranial CT models viewed from the left (D), right (E), and above (F) demonstrate bilateral skull fractures (arrows) that extend to the sagittal suture, but do not directly communicate. G, A thick axial coronal oblique reformat again shows no communication of the fractures (arrows). H, Review of the lateral view from the skull series at six months of age reveals a faint lucency (arrows) in the identical location of the subsequent parietal fracture, suggesting a reinjury at nine months.
Complex fractures consist of more than one fracture line. They may have a branching pattern (Figs. 17.8, 17.9) or a stellate configuration so that numerous fractures radiate from a central point. If the fracture results in one or more separate fragments, it is considered comminuted. Complex fractures are most frequently encountered in the posterior parietal and occipital regions (Fig. 17.8). Stellate fractures may have vertical and transverse components radiating out from a central impact point. Fracture segments oriented in the craniocaudad direction are visualized on standard axial sections and the coronal reformat will optimize detection of fractures running in the anteroposterior (AP) plane. Reliance on two-dimensional (2D) imaging alone can fail to provide optimal fracture characterization and 3D models may detect subtle fractures not suspected on the 2D images (Fig. 17.9G). When complex fractures are identified and no soft tissue swelling is seen, a fracture may be old, a consideration which is further strengthened when there is a large head circumference (HC) and other findings are present to suggest chronic increased intracranial pressure.
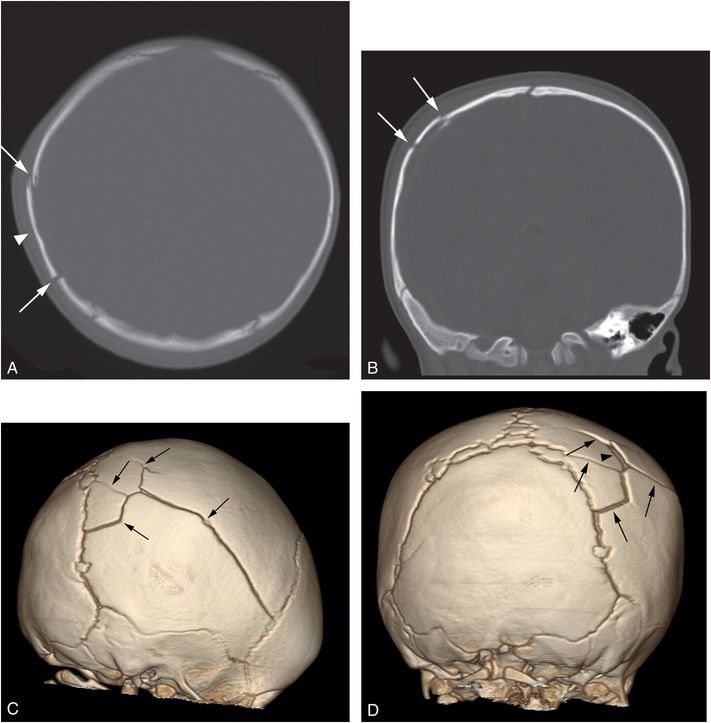
Figure 17.8 Complex fractures. An eight-month-old infant presenting with six days of right scalp swelling and vomiting for one day. Physical examination revealed boggy right scalp soft tissues and a step-off deformity of the skull. Axial head CT (A) with coronal reformat (B) and 3D models viewed from the right (C) and behind (D) shows a comminuted somewhat stellate right parietal fracture (arrows) with slight elevation of a comminuted fragment (arrowhead). There was underlying extra-axial blood and parenchymal injury (not shown). SS revealed multiple healing rib fractures (not shown).
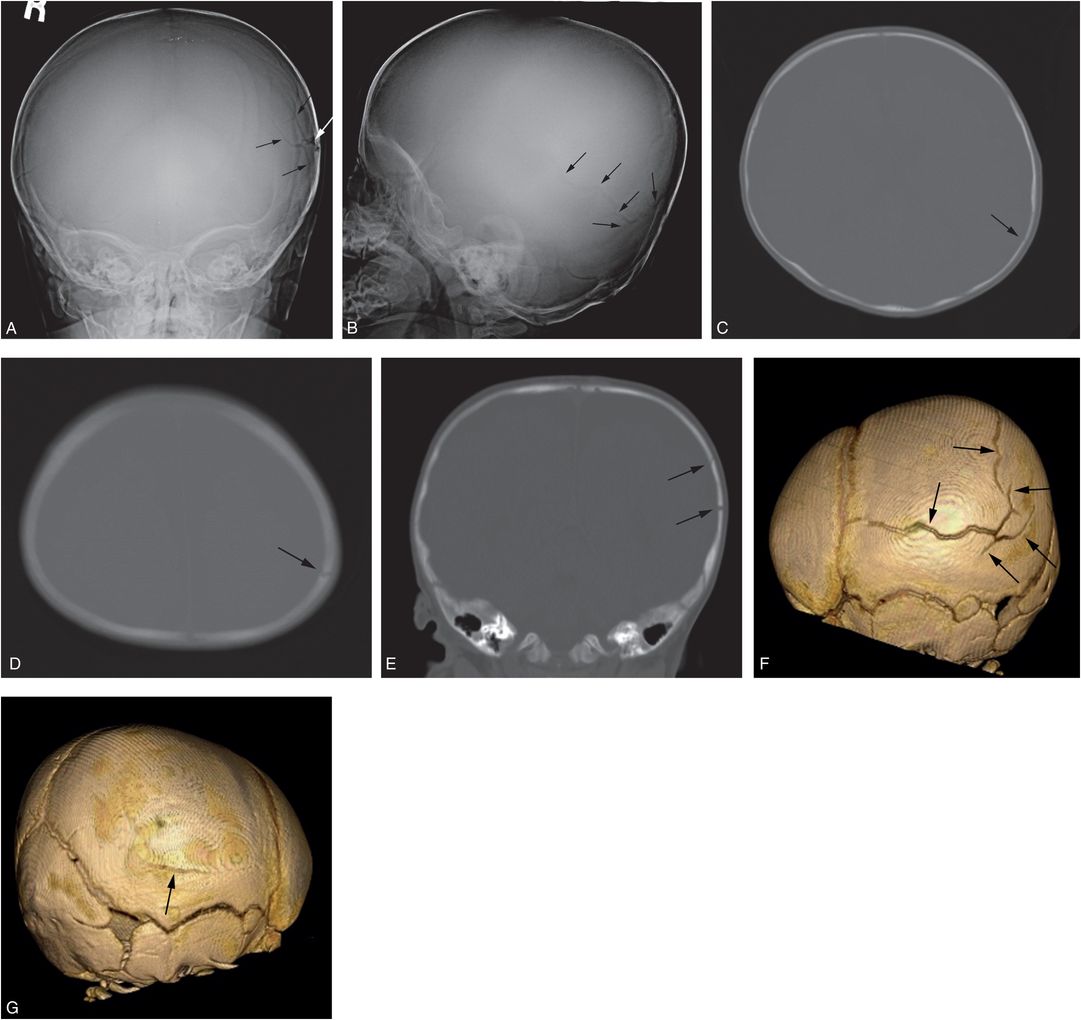
Figure 17.9 Complex skull fracture. A four-month-old infant with increasing HC. CT revealed bilateral SDHs and a left parietal skull fracture. SS demonstrated a complex skull fracture but was otherwise normal. There was no history of trauma, although bruising was noted above the right orbit. AP (A) and lateral (B) views of the skull demonstrate a complex left parietal fracture with a branching pattern (arrows). C, D, On the axial CT images the vertically oriented fracture is well defined (arrows), but only the coronal reformat (E) demonstrates the fracture components oriented in the AP plane (arrows). F, The true character and extent of the stellate fracture (arrows) is best demonstrated on the 3D cranial model viewed from the left. G, An unsuspected subtle linear right parietal fracture is also evident on the 3D model viewed from the right (arrow). Note is also made of postural brachycephaly with occipital flattening that is particularly evident in D.
If a fracture fragment is displaced inward, it is depressed. Depending on the plane of imaging, a fracture may appear linear and the depression will only be evident on a reformat that projects the downwardly displaced fragment in profile. The resultant deformity is well depicted on 3D models. Multiple depressed fractures suggest several impacts with a striking object, such as a hammer (Fig. 17.10) (73).
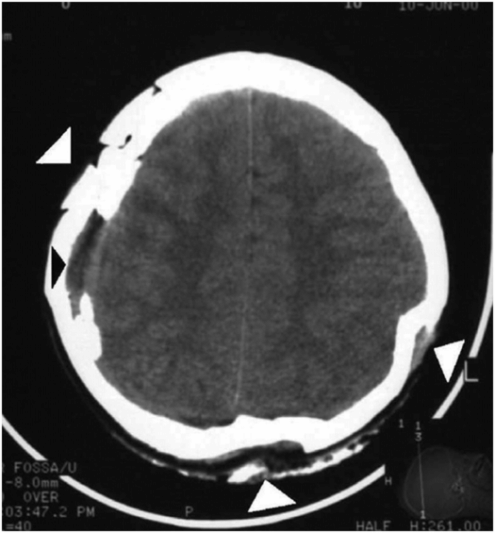
Figure 17.10 Multiple depressed skull fractures. CT of head in an 11-year-old child showing multiple and bilateral depressed fracture of the skull bones (white arrowheads) and aSDH at the right temporal region (black arrowhead). (Reprinted with permission from Lee AC, Ou Y, Fong D. Depressed skull fractures: a pattern of abusive head injury in three older children. Child Abuse Negl. 2003;27(11):1323–9.)
If a fracture fragment is displaced outward, it is elevated. Complex skull fractures with comminuted, elevated, or depressed fragments may be evident on careful palpation. When complex comminuted injuries are present and fracture fragments are displaced, it may be difficult to determine whether a fragment is displaced or whether the abutting fragment is elevated (Fig. 17.11), but full use of multiplanar reformats and 3D models usually resolve the issue (Fig. 17.12). Basilar skull fractures can pose a diagnostic challenge radiographically, since the fracture lucency may be obscured by the density at the skull base – occasionally the disruption of the calvarium may be evident on skull radiographs (Fig. 17.13). On cross-sectional imaging, the finding is more apparent, but may still be mistaken for a normal synchondrosis. Coronal and sagittal CT reformats and 3D models are invaluable in defining involvement of the basilar synchondroses. Although uncommon with abuse, they carry special significance; open vault fractures or those involving paranasal sinuses pose risk of infection; cribiform plate fractures may disrupt the olfactory nerves, causing anosmia, and petrous bone fractures can lead to hearing loss, VII nerve palsy, tinnitus, and vertigo. In addition to dedicated CT through the skull base, MRI is warranted in selected cases when basilar fractures are present. Additionally, complex central skull base fractures, and particularly those that involve the wall of the carotid canal warrant CT angiography (CTA) to evaluate for traumatic pseudoaneurysm or arterial dissection.
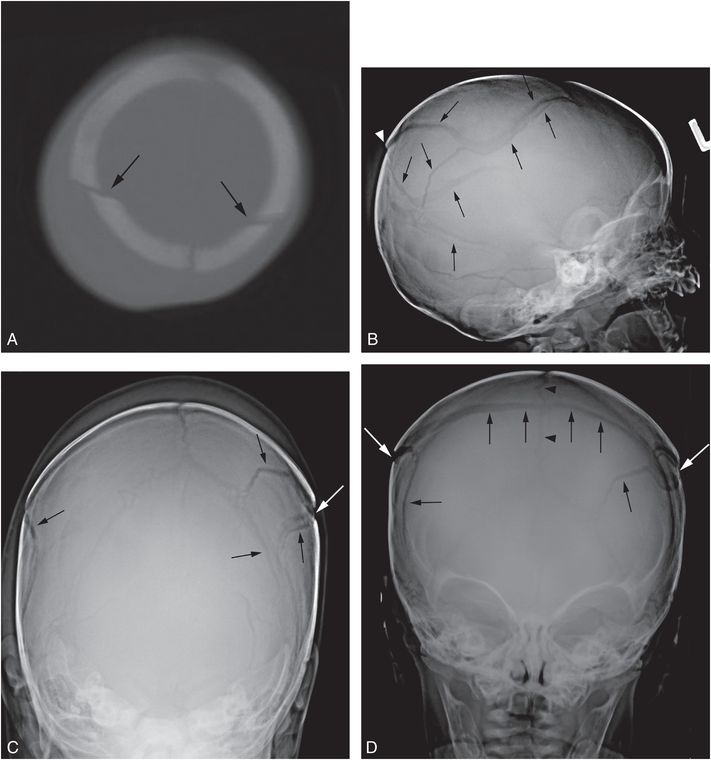
Figure 17.11 Complex fracture. A two-month-old infant with fussiness and right scalp swelling noted by parent on the evening of admission. A, High axial CT section demonstrates asymmetric soft tissue swelling and biparietal fractures that approach the midline vertex (arrows). SS demonstrated multiple healing rib fractures and a CML. Lateral (B), Towne’s (C), and AP (D) views of the skull demonstrate multiple complex diastatic skull fractures involving both parietal regions (arrows). On the AP view the fracture is seen to communicate across the midline via the sagittal suture (arrowheads in D). Displacement of a large parietal fragment is evident with a step-off deformity in the posterior parietal region (arrowhead in B), corresponding to the elevated fragment on the axial CT (A). Although there are bilateral fractures, it is not possible to determine whether they are the result of single or multiple impacts.
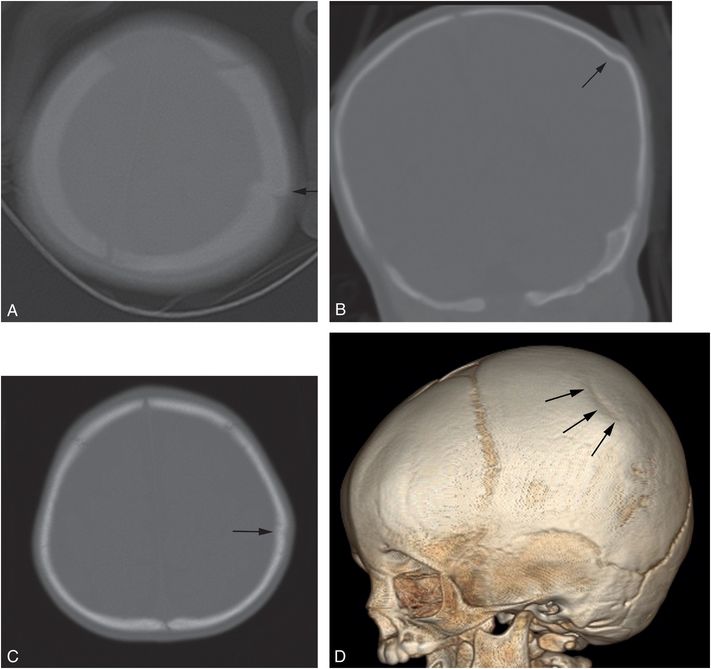
Figure 17.12 Depressed parietal fracture. A two-month-old infant who was strapped in a bouncy seat on the floor. (See same patient in Fig. 4.50.) In an unwitnessed event, a young sibling tipped the seat over causing the infant to strike her head. Axial CT (A) done elsewhere suggests an elevated fracture fragment (arrow), but the coronal reformat (B) reveals a mildly depressed left parietal fracture (arrow). There was soft tissue swelling, but no evidence of ICI. The infant returned at seven months of age with fussiness and diminished PO and blood noted in the posterior pharynx three days earlier. Chest radiograph showed healing rib fractures and SS showed a healing fracture of the right radius. Axial head CT (C) and a 3D model viewed from the left (D) now show flattening of the skull at the site of the previous depressed fracture (arrow), but no new abnormality.
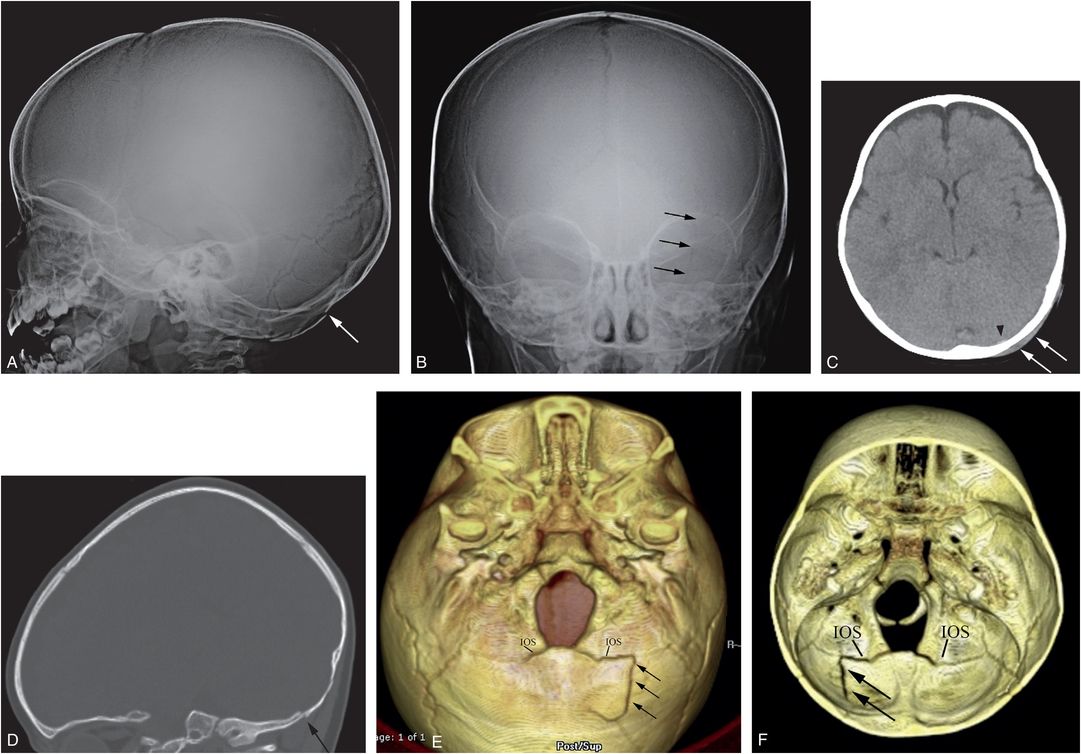
Figure 17.13 Basilar skull fracture. One-year-old not moving his left arm and unwilling to stand the morning after he was allegedly thrown from the crib by a three-year-old. (See same patient in Figs. 3.15, 3.69, 4.19, 5.40. SS showed multiple extremity, rib, clavicle, and skull fractures.) Lateral (A) and AP (B) views of the skull demonstrate a subtle linear fracture in the left occipital region (arrows). C, Axial CT shows a subtle scalp hematoma (arrows) and a tiny extra-axial high attenuation blood collection (arrowhead). D, The left parasagittal CT reformat shows a step-off of the occipital bone (arrow). E, The 3D model viewed from below and a cut-away view from above (F) show the linear skull fracture (arrows) extending through the left side of the supraoccipital bone into the diastatic IOS abutting the left exoccipital bone. The child remained neurologically intact.
Cranial sutures and synchondroses may also be diastased, either from extension of a calvarial fracture (Fig. 17.13), or as an isolated fracture of the suture (traumatic suture diastasis) (Fig. 17.14). Diastatic sutural injury should be distinguished from sutural splitting resulting from increased intracranial pressure. The latter tends to be more widespread than traumatic sutural diastasis, which is usually localized to a single suture and is often associated with a fracture. When sutural widening due to increased intracranial pressure is present, the widening between the margins of intersutural (Wormian) bones may result in a striking appearance, suggesting complex diastatic fractures (Fig. 17.15). The lack of expected soft tissue swelling points more to a developmental variant (74). Abused infants with focal traumatic sutural diastasis may subsequently develop split sutures due to progressive increased intracranial pressure (Fig. 17.14). Generalized sutural widening has also been described as a result of “catch-up” brain growth in children recovering from neglect or deprivation, and should not be misinterpreted as traumatic suture diastasis (see Chapter 23).
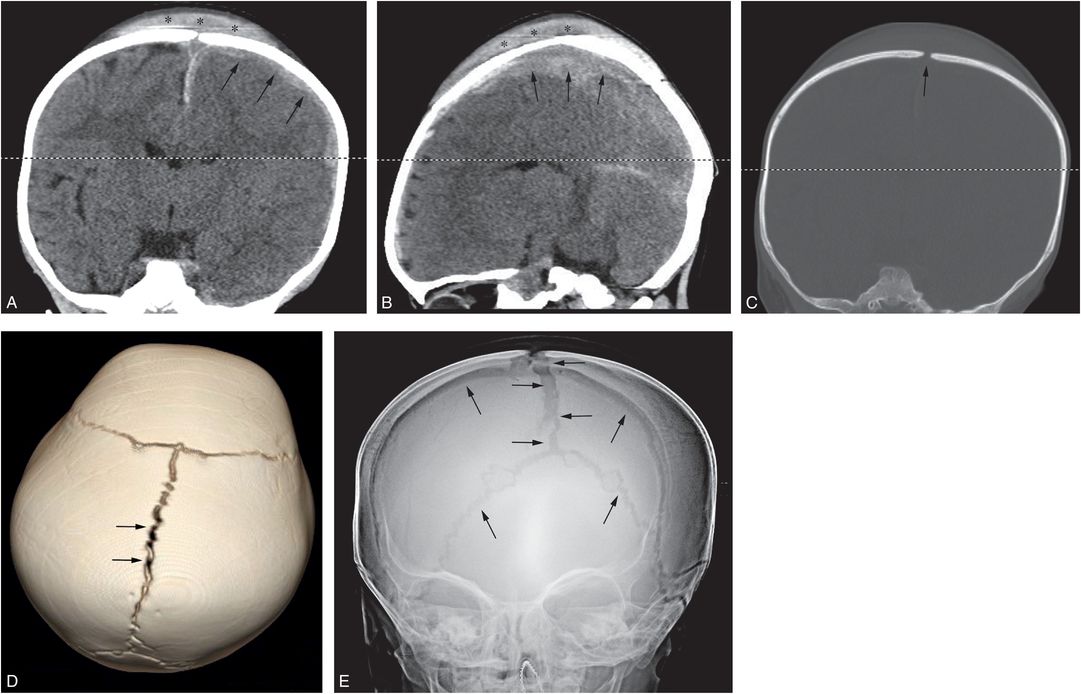
Figure 17.14 Traumatic suture diastasis. A 17-month-old infant with history of a fall from the babysitter’s couch. (See same patient in Figs. 19.17, 19.25, 19.26, 20.4, 21.26.) In the ER the infant was noted to be unresponsive with a tongue laceration. Coronal (A) and left parasagittal (B) CT reformats demonstrate a large vertex subgaleal hematoma spanning the midline (*), diffuse brain edema, and left SDH (arrows). On the coronal bone window reformat (C) and the 3D model viewed from above (D), there is diastasis of the sagittal suture (arrows). SS revealed a proximal left humeral metaphyseal fracture (not shown). The patient was declared dead on day 3. AP radiograph of the skull (E) at that time shows progressive diffuse sutural diastasis (arrows) due to increased intracranial pressure.
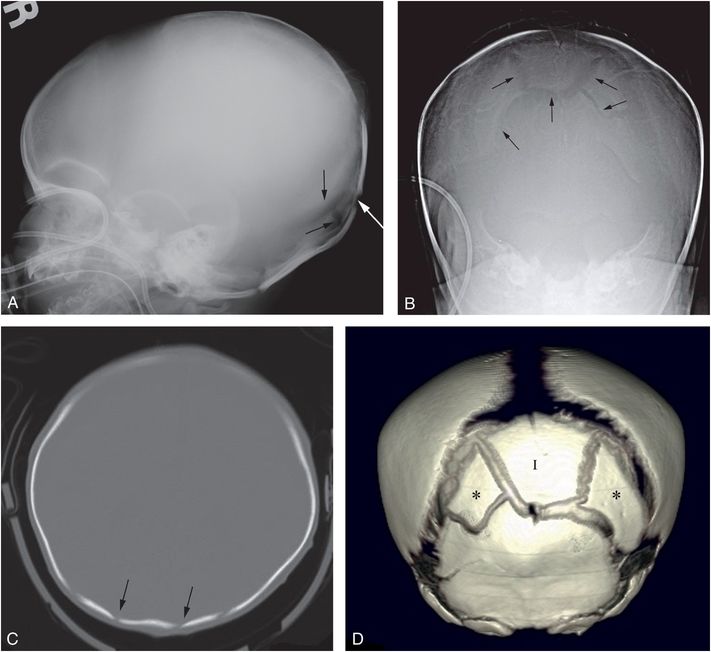
Figure 17.15 Split sutures with increased intracranial pressure. A six-week-old infant with lethargy and bilateral SDH. The SS revealed CMLs, clavicle, rib, and extremity fractures. Lateral (A) and Towne’s (B) views of the skull demonstrate complex lucencies in the occipital bone (arrows). C, CT demonstrates multiple lucencies in the occipital region (arrows) suggesting fractures without soft tissue swelling. D, The 3D model viewed from behind demonstrates a large intrasutural (Inca) bone (I) bordering the posterior fontanel. Additional posterolateral islands of ossification (*) lie adjacent to the Inca bone. Despite extensive ICI, the lack of soft tissue swelling and the presence of a large Inca bone are in keeping with developmental variants. There is widening of the sutures and conspicuity of intrasutural bones in this patient with increased intracranial pressure.
With increasing complexity and diastasis of a cranial fracture, there is a greater likelihood that an underlying dural defect will be present. A rent in the dura will allow extrusion of the underlying intracranial contents, which may result in “growth” of the fracture in as little as two months. If the process continues, actual herniation of arachnoid and CSF through this defect may result in a leptomeningeal cyst (75–79). Often there are overlying chronic subgaleal and underlying chronic subdural collections with adjacent encephalomalacia. Leptomeningeal cysts are rare beyond three years of age.
Fracture patterns and abuse
There may be considerable subjectivity in the assignment of a fracture pattern to complex cranial injuries, and to avoid confusion the features of the fracture(s) should be described in detail. Wiersema et al. recently proposed a standardized method to describe skull fractures (80). Numerous studies assess the various radiographic appearances of skull fractures in abused children, and some have sought to draw distinctions between the patterns of skull injury associated with abuse and those occurring with accidental injury. Hahn and associates studied 37 skull fractures in 77 abused children with ICI (20). They described 30 linear fractures, 5 depressed fractures, and 2 diastatic fractures. An additional six patients had traumatic suture diastases. The parietal bone was the most frequent site of fracture, showing involvement in 86% of cases. Merten and others found 93 cases of craniocerebral injury in 712 physically abused children; 67 skull fractures (72%) were seen in this group (15). Fifty-nine (88%) of these were simple linear fractures that were parietal or occipital in location. Diastatic, comminuted, and depressed fractures were uncommon. ICI was associated with fractures in 56% of cases.
Hiss and Kahana performed a postmortem forensic analysis of four young children with bilateral cranial fractures (81). They concluded that symmetric fractures most often result from bilateral compression of the head between two surfaces rather than from a localized impact to one side of the skull. However, as previously noted, bilateral fractures that are continuous across the midline can result from a single unilateral impact, and symmetric fractures may also occur with an impact to the cranial vertex (71, 82). Billmire and Myers studied the characteristics of 55 skull fractures in a group of 84 infants with head trauma (18). Thirty infants were subsequently found to be abused. Eighty-seven percent of the fractures were linear, occurring commonly in both accident and abuse victims. Two depressed fractures were noted, one in an abused child, the other in a child hit by a falling television. Four complex fractures were associated with intracranial hemorrhage, and all occurred in abused children.
In a study of 60 accidental fractures, Hobbs found that 55 were linear, 3 complex, 3 depressed, 4 diastatic (maximal width at presentation 3 mm), and 2 of the growing type (21). In contrast, of the 29 fractures associated with abuse, 6 were linear, 23 multiple or complex, 12 depressed, 10 diastatic, and 6 growing. In the abused infants with depressed fractures, the fragments were noted to lie within the central area of the complex bony injury. In three abused children, multiple depressed areas suggested repeated blows. There were six parietal and six occipital depressed fractures. A fatal outcome occurred in 50% of the children with parietal fractures and in all the children with occipital fractures. In the accidental cases, no fracture was wider than 5 mm. In the abused children, 6 fractures measured over 5 mm in width. In 40 accidental trauma cases, the maximal width of the fracture was less than 1 mm. In the abused infants, none of the fractures were less than 1 mm in width. In contrast to previous reports, Hobbs found that the presence of a growing fracture had a strong association with abuse. He concluded that a growing fracture implies a severe impact force, and that if a minor injury is alleged in such cases, abuse is likely. Hobbs considered depressed fractures to be strongly suspicious of an inflicted injury from a high impact blunt force, unless a definite history of a direct contact injury with a sharp or pointed object is provided, and that a depressed occipital bone fracture is virtually pathognomonic of abuse.
In contrast, Meservy and colleagues found no significant differences in the patterns of skull fracture between abused and accidentally injured children regarding depressed fractures, diastatic fractures of 3 mm or more, nonparietal fractures, or fractures having a complex configuration (31). These results, however, concurred with those of Hobbs in that multiple fractures, bilateral fractures, and fractures that crossed suture lines were significantly associated with abuse (21). Reece and Sege found no significant differences in the prevalence of complex skull fractures in abusive versus accidental head injuries (37). In a systematic literature review from 1982 to 2000, Kemp and others found that most skull fractures in abused children were linear parietal injuries, and emphasized the lack of agreement of studies with respect to the predictive value of diastatic and complex fractures for abuse (18, 31–33, 35, 36, 38, 62, 83).
In Barber and colleagues’ recent study of 138 calvarial injuries, 55% (76/138) had simple skull fractures and 45% (62/138) had complex injuries (42). Overall, 19% (26/138) had a positive SS (unsuspected fracture) and the likelihood of a positive SS was significantly higher in infants with complex skull fractures (27% [17/62]) versus those with simple fractures (12% [9/76]). Infants with a complex skull fracture were at higher risk for other fractures (OR: 2.8; 95% CI: 1.2–6.9). Although there was no overall correlation between skull fracture and ICI, 53% (31/59) of infants with complex skull fracture had ICI, compared with 29% (22/75) of infants with simple skull fracture and ICI, a statistically significant finding. Infants with a complex skull fracture had a higher risk of associated ICI (OR: 2.7; 95% CI: 1.3–5.4).
John et al. recently reviewed the records of 519 children under 3 years of age with head injuries and abnormal neuroimaging seen over a 10-year period (84). Skull fractures were documented in 355 patients (68%). They dichotomized the data in children less than six months of age and those aged six months to three years. In both age groups, the proportion with fractures increased with fall height and when there was a history of trauma as opposed to none. The proportion with complex fractures also rose with fall height in the six-month-to-three-year cohort, but not in those younger than six months. Their observations support the view that when complex skull fractures are found in young infants with a history of a short fall, child abuse should be strongly considered.
Isolated basilar skull fractures are infrequently identified in cases of abuse. In a study of 34 temporal bone fractures in children, Nicol and Johnstone found that only 1 fracture was due to abuse (85). In contrast to isolated basilar fractures, extension of calvarial injuries to the skull base, particularly the foramen magnum, is not unusual. It should be reemphasized that the presence of extensive and complex cranial fractures crossing the sutures does not necessarily indicate multiple injuries. A single major impact, particularly when applied at the midline, may transfer sufficient energy to the cranial vault to produce a complex and even an eggshell fracture pattern.
Because linear fractures commonly occur with abuse and accidental trauma, and no pattern of skull injury is diagnostic of abuse, the specific cause of a skull fracture in a particular patient can be determined only when viewed in conjunction with the history provided. Abuse should be suspected when a child with reportedly mild head trauma presents with multiple, complex, or diastatic skull fractures, particularly when the fracture involves the occipital bone. However, considerable restraint should be exercised in considering AHT when simple linear fractures are encountered, including those parietal fractures that communicate across the midline via the sagittal suture. Depressed fractures are not particularly characteristic of abuse, and most are accidental. When an accidental fall is alleged to explain any skull fracture, knowledge of the height of the fall and the nature of the surface impacted is critical. Furthermore, care should be taken to differentiate a depressed fracture with a discrete inwardly displaced fracture fragment from an inward buckling of the skull, or “ping-pong” fracture, typically seen in young infants with falls to a flat surface (see below).
Patterns of repeat cranial injury
Infants with missed AHT often return with repetitive neurotrauma (86, 87). There is little literature regarding calvarial re-injury patterns, but the presence of a prior skull fracture or perhaps a normal variant may alter the features of secondary osseous injury. Figure 17.16 illustrates an infant with an initial right linear parietal fracture and either a short linear fracture or a normal fissure in the contralateral parietal region. Imaging following a subsequent abusive event showed extension of this original left-sided lucency to a lengthy linear left parietal fracture. Figure 17.17 illustrates another infant with a prior linear parietal fracture that developed a branching configuration after a subsequent inflicted injury that also resulted in a contralateral diastatic parietal fracture. These illustrated cases highlight the potential for the imaging manifestations of an acute traumatic event to be influenced by a prior injury or normal variant. Many infants with abusive head injury have a history of earlier trauma, and a thorough correlation of all available neuroimaging is essential to elucidate the clinical problem (86). Further study with 3D modeling may shed light on the interesting and poorly understood issue of repetitive cranial injury.
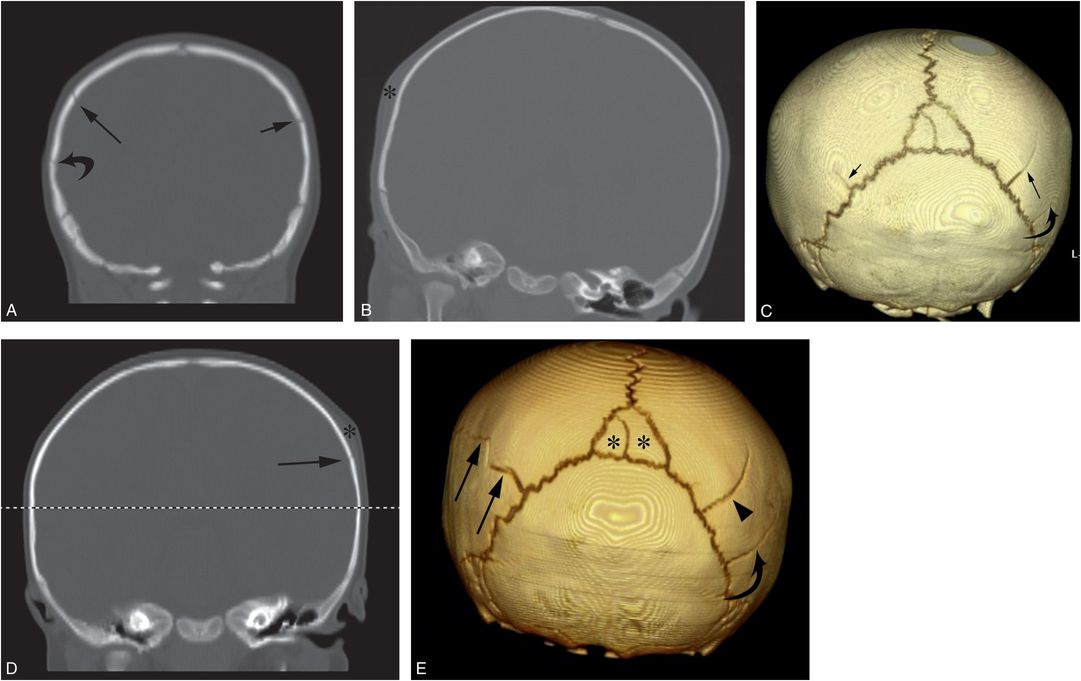
Figure 17.16 Repeat cranial injury. A six-month-old infant with right scalp swelling and no history of trauma. Coronal CT reformats (A, B) and the 3D model viewed from behind (C) demonstrate a linear right parietal skull fracture (long arrows) with adjacent soft tissue swelling (*). A short linear lucency (short arrows) is noted extending anteriorly from the left lambdoid suture, consistent with a second fracture or a normal fissure. Following a CPT consult, normal ophthalmologic assessment, and an otherwise unremarkable SS, the child remained in the custody of the mother. The infant returned three weeks later with unexplained left scalp swelling. Coronal reformat (D) and 3D model viewed from behind (E) of the repeat CT show new left-sided soft tissue swelling (* in D) with an underlying posterior parietal fracture (long arrows). Note that the fracture extends from the posterior parietal lucency visible on the initial CT (small arrow in C). The original right posterior parietal fracture is again seen (arrowhead in E). A second lucency, likely a normal fissure, is evident (curved arrow) just below the original fracture that was also visible on the initial images (curved arrows in A and C). There is also an incidental bipartite midline intrasutural (Wormian) bone (* in E).
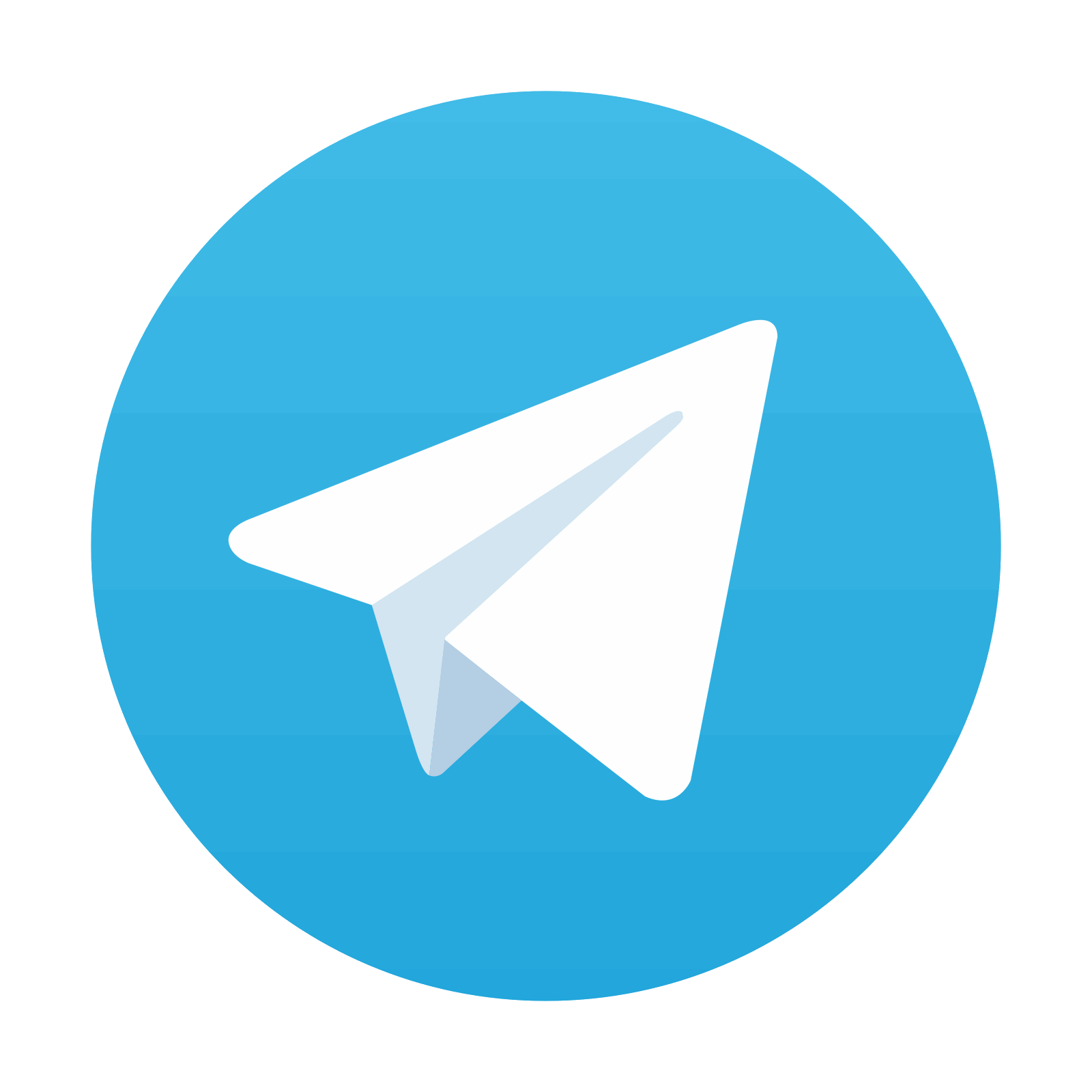
Stay updated, free articles. Join our Telegram channel

Full access? Get Clinical Tree
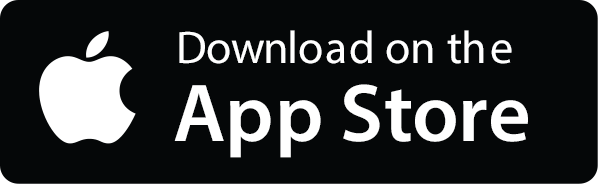
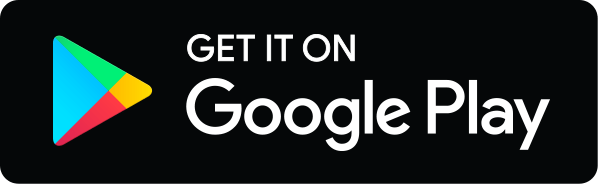