The high soft tissue contrast and tissue characterization properties of magnetic resonance imaging allow further characterization of indeterminate mediastinal lesions on chest radiography and computed tomography, increasing diagnostic specificity, preventing unnecessary intervention, and guiding intervention or surgery when needed. The combination of its higher soft tissue contrast and ability to image dynamically during free breathing, without ionizing radiation exposure, allows more thorough and readily appreciable assessment of a lesion’s invasiveness and assessment of phrenic nerve involvement, with significant implications for prognostic clinical staging and surgical management.
Key points
- •
Magnetic resonance (MR) imaging can add diagnostic specificity to the evaluation of indeterminate mediastinal lesions on radiography and computed tomography, serving as a means of virtual biopsy.
- •
Mediastinal MR imaging can assist in directing intervention and guiding surgical management, and can serve as a means of surveillance without exposure to ionizing radiation.
- •
Dynamic MR imaging during free breathing amplifies assessment of lesion invasiveness and phrenic nerve involvement.
- •
Using MR imaging to characterize mediastinal lesions noninvasively can have a significant impact on patient care.
Video content accompanies this article at http://www.radiologic.theclinics.com .
Introduction
Imaging of the mediastinum has long relied on chest radiography (CXR) and computed tomography (CT) to triage benign, malignant, and indeterminate lesions in terms of clinical management. A thorough understanding of the anatomy and classification schemas has allowed radiologists to localize and differentiate lesions, narrowing diagnostic possibilities. However, some lesions remain indeterminate, many of which are benign. Before the use of magnetic resonance (MR) imaging, many indeterminate mediastinal lesions required longitudinal CT follow-up, exposure to repeated doses of ionizing radiation, and often unnecessary surgical excision. MR imaging has the capacity to further characterize these indeterminate lesions and guide clinical management, on account of its superior soft tissue contrast and tissue characterization properties.
Discussion
Nature of the Problem: Limitations of Computed Tomography and the Added Value of Magnetic Resonance Imaging
One primary limitation of CT that must be considered is the ionizing radiation required to produce diagnostic images. Although progress has been made since the inception of CT to reduce radiation dose, diagnostic ionizing radiation exposure over a patient’s lifetime remains a concern because of higher cancer risk. Adhering to the guiding principle of radiation safety, as low as reasonably achievable, continued efforts must be made to limit radiation exposure throughout each patient’s lifetime. The lack of ionizing radiation use and exposure by MR imaging is therefore compelling.
Although CT is able to quickly and noninvasively provide cross-sectional evaluation of lesions, its soft tissue contrast and tissue characterization capability are inferior to those of MR imaging. As a result, CT does not always allow confident definition of a lesion’s relationship to surrounding structures ( Fig. 1 ), definitive placement of a lesion into a specific mediastinal compartment, and confident delineation of a lesion’s borders and invasiveness, all of which can have profound diagnostic and prognostic implications affecting clinical management.
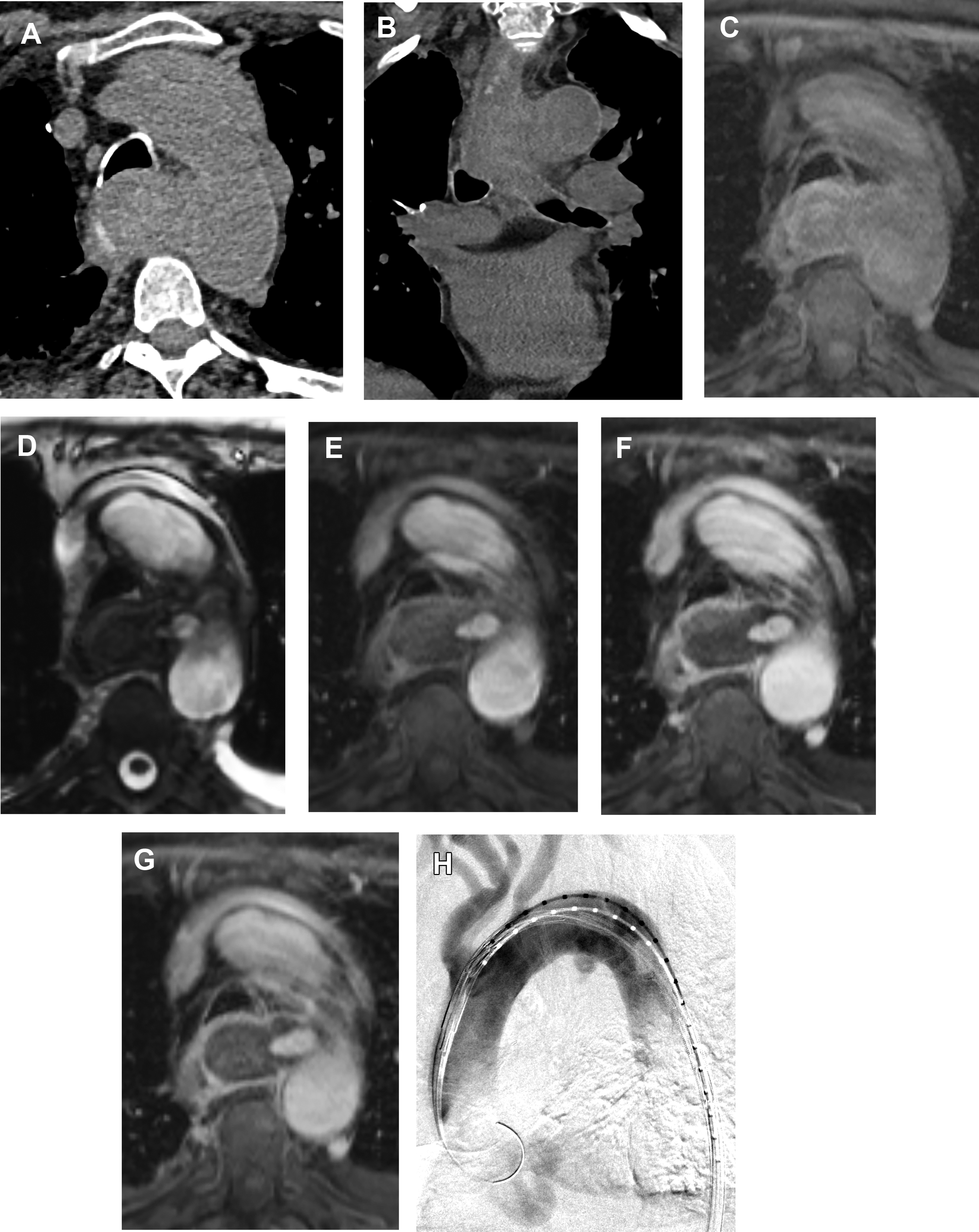
Determining a lesion’s internal characteristics is also imperative to diagnosis. Although CT does a good job delineating structures with starkly differing attenuation, such as air, macroscopic fat, water, and bone, it is less proficient at differentiating tissues of similar attenuation, of which there are many. A stark example is the frequent inability of CT, whether performed without or with intravenous (IV) iodinated contrast, to differentiate a hyperattenuating or isoattenuating cyst from a solid lesion, which typically occurs when the cyst contains sufficient proteinaceous or mineral material (eg, hemorrhage, calcium oxalate, manganese, magnesium) ( Fig. 2 ).
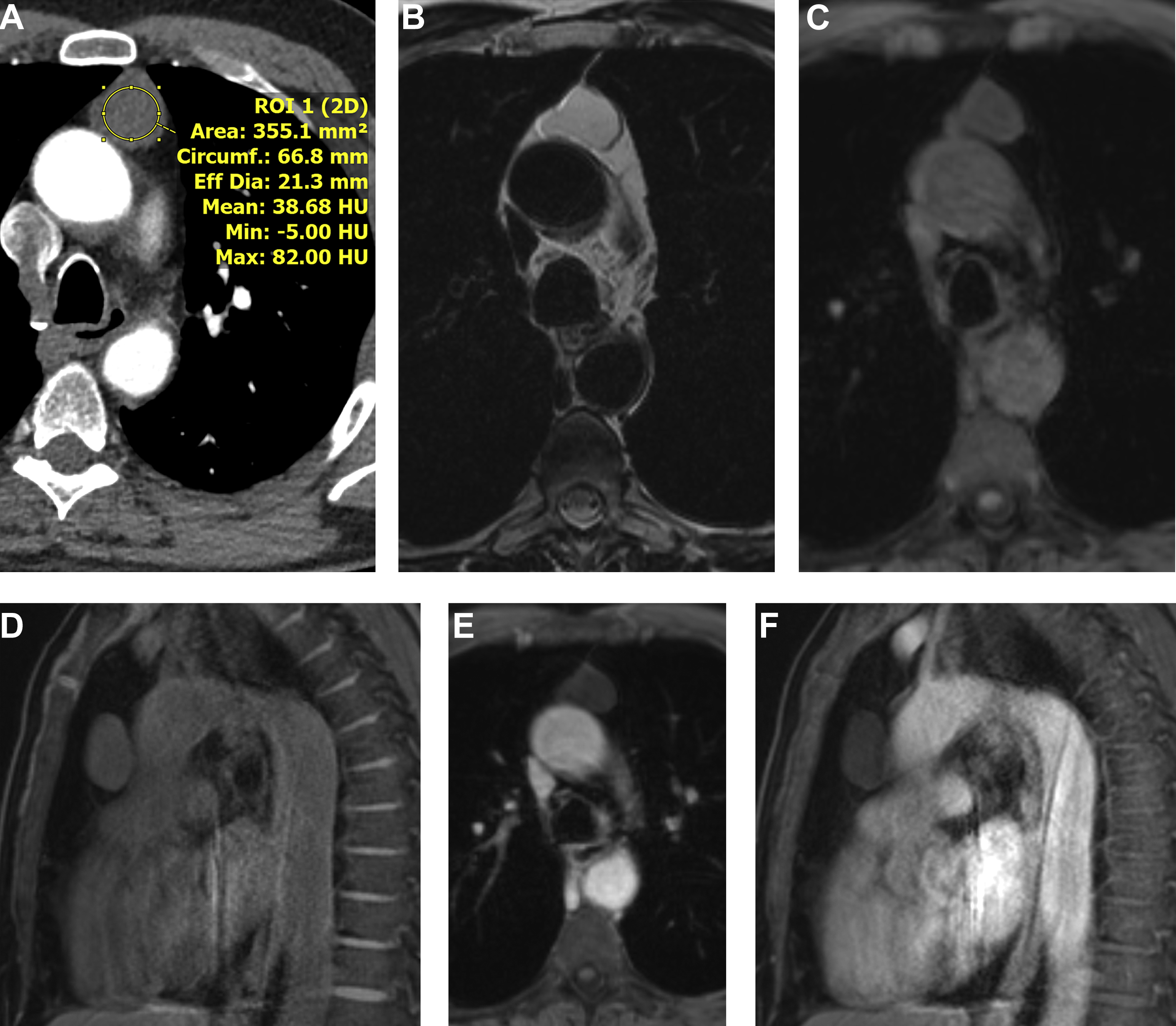
Use of IV iodinated contrast contributes to tissue characterization and can thereby narrow the differential diagnosis. Chest CT imaging without and with IV contrast and dynamic contrast enhancement (DCE) are seldom performed because of the consequential doubling and quadrupling of ionizing radiation exposure, respectively. The inferior soft tissue contrast of CT, compared with MR imaging, additionally limits the ability of CT to detect subtle enhancement ( Fig. 3 ). Dual energy techniques have shown promise in further characterizing lesions; however, inherent limitations remain. Dynamic contrast evaluation with automatic postprocessed subtraction enables MR imaging to provide potentially valuable information regarding a lesion’s temporal enhancement pattern and cellularity, without the risk conferred by added ionizing radiation exposure.
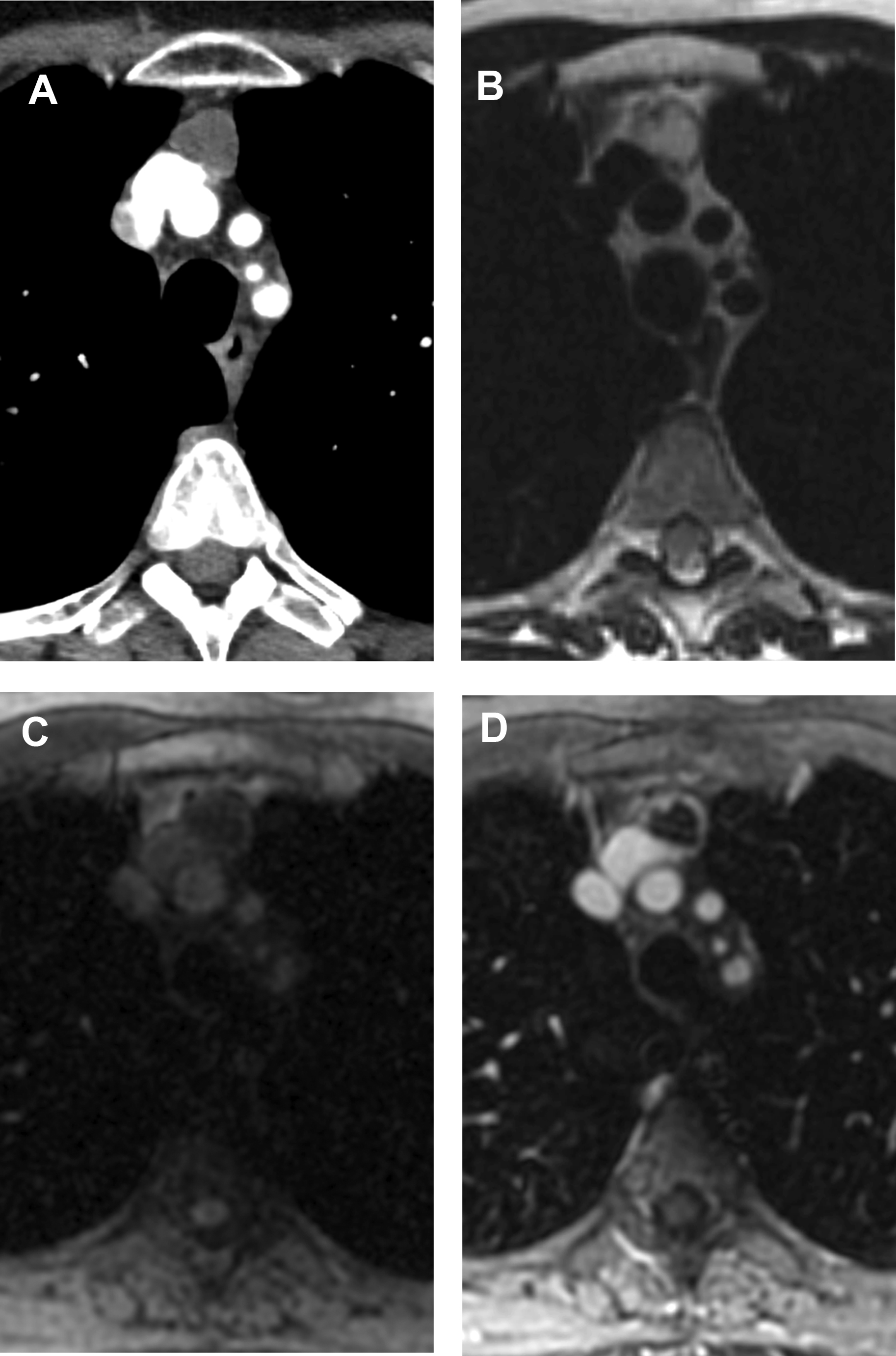
In addition to serving as a means of noninvasive tissue sampling or virtual biopsy, preventing unnecessary diagnostic intervention, MR can facilitate diagnostic tissue sampling. Because MR imaging is proficient at identifying viable, cellular tissue, it can direct the interventionalist or surgeon to biopsy the area of highest diagnostic yield and accessibility ( Fig. 4 ).
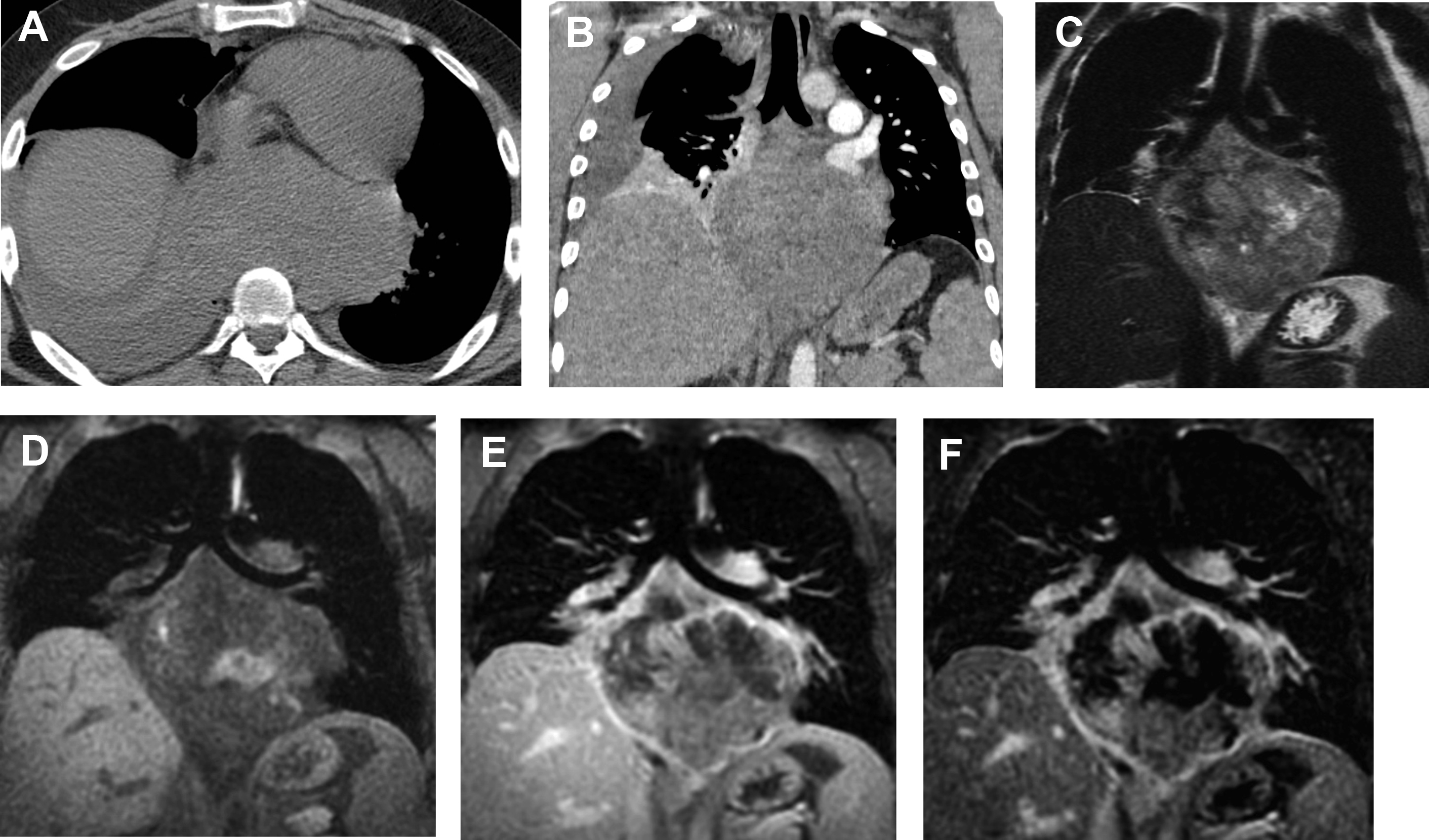
Although MR imaging surpasses the ability of CT regarding lesion characterization, it has its own limitations. The greatest limiting factor is acquisition time. MR imaging requires a patient to lie still for 15 to 45 minutes, which for most patients is possible; however, it limits the number of examinations that can be performed per day at a given site. Nevertheless, MR imaging pulse sequence acquisition times continue to improve with advances in software and hardware with every passing year. In addition, imaging protocols tailored to indication and accommodation of patients’ needs can surmount these limitations and expedite an imaging diagnosis. In terms of tissue characterization, MR imaging’s sole limitation, compared with CT, is its inability to specifically identify calcium. Because of its ability to show many other, often more important, features of a lesion, this limitation rarely has clinical implications ( Fig. 5 ). Also, because MR imaging is often used to problem solve indeterminate lesions on CT, the presence or absence of calcium in a lesion is usually known.
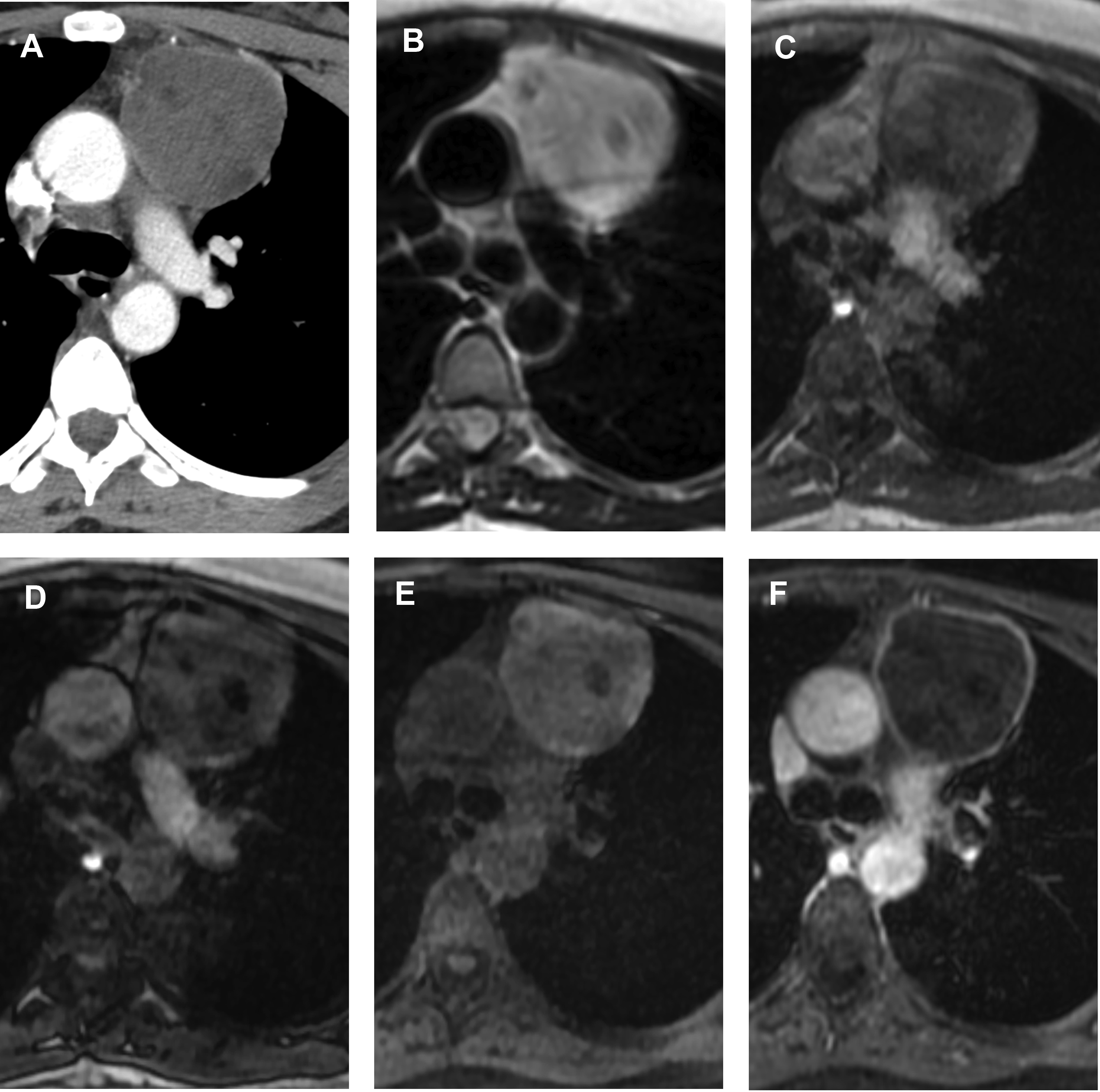
Imaging Protocol
Limiting the effects of cardiac and respiratory motion is essential for the acquisition of high-quality images and reduction of image-degrading artifacts ( Fig. 6 ). Limiting respiratory motion artifact can be achieved via breath-hold imaging or respiratory triggering. When possible, breath-hold imaging is preferable, because it more reliably freezes respiratory motion and is a much faster image acquisition method. Considerations must be made regarding coverage in order to limit breath-hold duration, because prolonged breath holds (>20 seconds) and an increased overall number of breath-hold sequences can result in patient fatigue and suboptimal image quality. Breath-hold imaging can be reliably achieved in most patients with preemptive coaching by the technologists and the use of supplemental, MR-compatible nasal cannula oxygen, the latter when difficulty is anticipated. ,
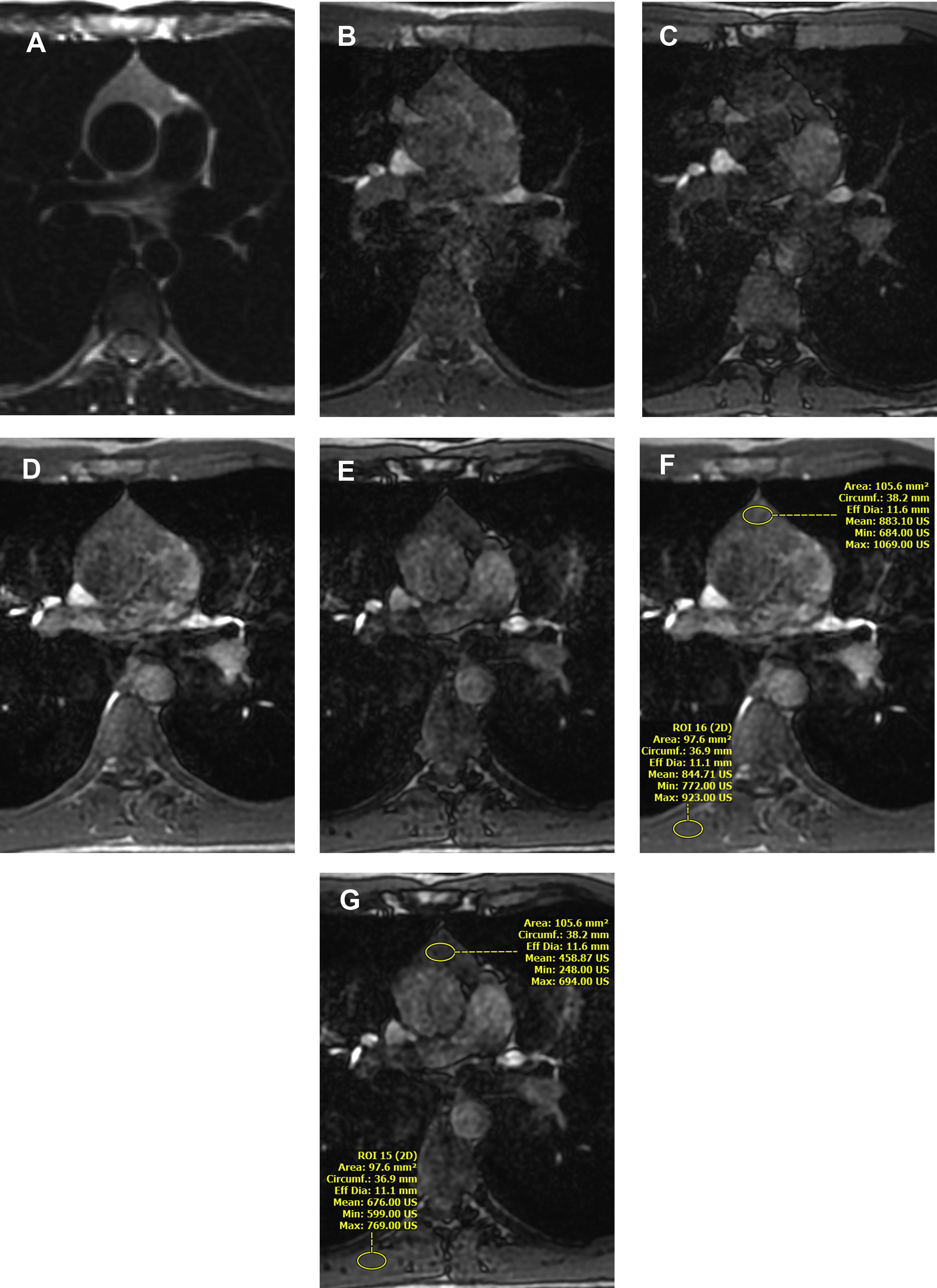
Evaluation of mediastinal lesions by MR imaging requires complementary high-quality sequences to aid in tissue characterization. In general, these include a breath-hold two-dimensional (2D) or three-dimensional (3D) T1-weighted sequence; a breath-hold or respiratory-triggered T2-weighted sequence, with or without fat saturation; and a breath-hold pregadolinium and postgadolinium 3D ultrafast gradient echo (GRE), dynamic contrast-enhanced (DCE), fat-saturated T1-weighted sequence, with automatic postprocessed subtraction. The use of dual echo ultrafast GRE in-phase and out-of-phase chemical shift MR imaging for the T1-weighted sequence allows full coverage of most lesions and simultaneous acquisition of both phases in a single 20-second breath-hold. The addition of diffusion-weighted imaging (DWI) with apparent diffusion coefficient (ADC) mapping can further narrow the differential diagnosis in specific instances and provide reassuring information with regard to likely benignity when IV contrast cannot be administered. Short tau inversion recovery imaging is rarely needed for mediastinal mass evaluation, although it can be useful if detection of subtle bone marrow edema is needed.
Respiratory-triggered imaging (usually for T2-weighted sequences, although increasingly available for T1-weighted imaging) can be used when breath-hold capacity is limited or when the need for extended coverage would require multiple axial stacks and an undue number of serial breath holds. Respiratory-triggered imaging acquires data during a single phase of the respiratory cycle (usually end-expiratory), resulting in longer scan time and some compromise of lung evaluation on account of atelectasis. Further advancements in scanner software have led to increasing availability of high-quality T1-weighted GRE imaging during free breathing: so-called stack-of-stars.
Effective electrocardiogram (ECG)-facilitated cardiac gating freezes cardiac motion and eliminates pulsation artifact via image acquisition during a specific phase of the cardiac cycle (usually the R wave). Peripheral pulse gating is less desirable because the time delay between the heartbeat and the peripheral pulse leads to less precise cardiac gating and residual pulsation artifact. Cardiac gating may be preferable for T2-weighted imaging when the field of coverage requires fewer than 10 to 14 slices, because it yields higher-quality images than respiratory-triggered, radially acquired, fast spin-echo T2-weighted images and breath-hold single-shot fast spin-echo images. This benefit comes at the cost of 1 breath-hold per slice, as opposed to no breath holding or a single breath-hold for the entire acquisition.
Use of 3D ultrafast GRE, fat-saturated T1-weighted imaging allows the evaluation of dynamic contrast enhancement (DCE). These scans are acquired at selected intervals to allow evaluation of temporal enhancement characteristics. Suggested intervals include 20 seconds (angiographic phase), 1 minute, 3 minutes, and 5 minutes after injection, with automatic, postprocessed subtraction. Planes of image acquisition should be tailored to the patient-specific imaging question and usually include at least 1 orthogonal plane.
DWI can complement the aforementioned imaging sequences to further define a lesion’s characteristics. Measuring signal decay related to motion of water molecules relies on gradient pulses of different intervals and intensity denoted as b values. The more b values used, the more accurate the ADC map; however, this comes at the cost of time to acquire each individual b value. A minimum of 3 b values is a reasonable compromise. Low b values (<50) result in nearly pure perfusion-weighted imaging because of microvascular blood flow and resultant T2 shine-through. The higher the b value, the more accurate the reflection of true diffusion. However, this comes at the cost of increased image noise with values greater than or equal to 1000. Thus, a range of b values greater than 0, evenly spaced less than 1000, can facilitate an accurate ADC map in the chest. Suggested b values are 50, 400, 800.
A standard mediastinal MR protocol and a thymus MR protocol, along with optional, supplementary sequences, are provided in Tables 1 and 2 .
Protocol | Technique | Sequences |
---|---|---|
Mediastinum |
| BH 3 plane localizer |
BH sagittal and axial balanced gradient echo or steady state free precession (SSFP) (FIESTA/true FISP/b-FFE) a | ||
BH coronal SSFSE/HASTE/SSTSE T2W | ||
BH axial ECG-gated DIR T2W(∼20 s or 1 BH/slice) b | ||
BH axial and sagittal c pre-3D ultrafast gradient echo (LAVAFLEX/DIXON/mDIXON) fat-saturated T1W DCE d , e | ||
BH post-3D ultrafast gradient echo (LAVAFLEX/DIXON/mDIXON) fat-saturated T1W: axial 20 s, axial 1 min, sagittal c 3 min, axial 5 min) f |
a Order of MR acronyms presentation: GE/Siemens/Philips.
b Substitute radially acquired T2W imaging (PROPELLER/BLADE/Multivane) when full chest coverage is needed and for large masses, when extent of coverage makes ECG-gated DIR T2W imaging onerous on account of the number of serial 20-second breath-hold that would be needed to cover the lesion. Can also use this T2W sequence when a patient is having difficulty breath-holding in general.
c Orthogonal plane subject to change, depending on location of lesion relative to other important structures.
d All 4 series sent to Picture Archive and Communication System (PACS), including in-phase/out-of-phase T1W, water-only, and fat-only series.
e Optional BH before/after LAVAFLEX/DIXON/mDIXON T1W coronal can be added or substituted.
Protocol | Technique | Sequences |
---|---|---|
Thymus |
| BH 3-plane localizer |
BH axial balanced gradient echo (FIESTA/true FISP/b-FFE) | ||
BH coronal SSFSE/HASTE/SS TSE T2WI | ||
BH axial ECG-gated double IR T2WI (∼20 s or 1 BH/slice) a | ||
BH axial and sagittal b pre-3D ultrafast gradient echo (LAVAFLEX/DIXON/mDIXON) fat-saturated T1W c , d | ||
BH post-3D ultrafast gradient echo (LAVAFLEX/DIXON/mDIXON) fat-saturated T1W DCE: axial 20s, axial 1 min, sagittal b 3 min, axial 5 min) e |
a Substitute radially acquired T2W imaging (T2WI) (PROPELLER/BLADE/MULTIVANE) for large masses, when extent of coverage makes ECG-gated DIR T2W imaging onerous on account of the number of serial 20-second breath holds that would be needed to cover the lesion. Can also use this T2W sequence when a patient is having difficulty breath-holding in general.
b Orthogonal plane subject to change, depending on location of lesion relative to other important structures.
c All 4 series sent to PACS, including in-phase/out-of-phase T1W, water-only, and fat-only series.
d Optional BH before/after-LAVAFLEX/DIXON/mDIXON fat-saturated T1W coronal can be added or substituted.
Evaluation: Tissue Characterization by Magnetic Resonance Imaging
Fat content
Mediastinal lesions are often surrounded by fat and, in some cases, contain macroscopic or microscopic fat. The use of appropriate fat suppression techniques can reduce the signal of both macroscopic and gross fat, including that present in the prevascular space and subcutaneous fat of the chest wall, and microscopic or intravoxel fat (when fat-containing and water-containing molecules are present in the same voxel). The former is achieved by standard fat saturation and the latter is achieved by opposed-phase chemical shift MR imaging. Fat saturation highlights a lesion’s signal characteristics by eliminating the competing high T1/T2 signal of the adjacent macroscopic fat. Identification of macroscopic or microscopic fat within a lesion often adds diagnostic specificity (see Fig. 5 ; Fig. 7 ).
