The advanced imaging techniques outlined in this article are only slowly establishing their place in surgical practice. Even a low risk of false information is unacceptable in neurosurgery, thus decision-making is necessarily conservative. As more validation studies and greater experience accrue, surgeons are becoming more comfortable weighing the quality of information from functional imaging studies. Advanced imaging information is highly complementary to established surgical “good practice” such as anatomic planning, awake craniotomy, and electrocortical stimulation; its greatest impact is perhaps on how neurosurgery is planned and discussed before the patient is ever brought to the operating room. Access to functional magnetic resonance (MR) imaging, diffusion tractography, and intraoperative MR imaging can influence neurosurgical decisions before, during, and after surgery. However, the widespread adoption of these techniques in neurosurgical practice remains limited by the lack of standardized methods, the need for validation across institutions, and the unclear cost-effectiveness particularly for intraoperative MR imaging. Before advanced imaging results can be used therapeutically, it is incumbent on the neurosurgeon and neuroradiologist to develop a working understanding of each technique’s strengths and weaknesses, positive and negative predictive values, and modes of failure. This content presents several imaging methods that are increasingly used in neurosurgical planning. As these techniques are progressively applied to surgery, radiologists, medical physicists, neuroscientists, and engineers will be necessary partners with the treating neurosurgeon to bridge the gap between the experimental and the therapeutic.
The development and validation of advanced anatomic and functional imaging is expanding the toolbox available for neurosurgical planning. Access to functional magnetic resonance (fMR) imaging, diffusion tractography, and intraoperative MR (iMR) imaging can influence neurosurgical decisions before, during, and after surgery. However, the widespread adoption of these techniques in neurosurgical practice remains limited by the lack of standardized methods, the need for validation across institutions, and the unclear cost-effectiveness, particularly for iMR imaging.
Before advanced imaging results can be used therapeutically, it is incumbent on the neurosurgeon and neuroradiologist to develop a working understanding of each technique’s strengths and weaknesses, positive and negative predictive values, and modes of failure. For example, language localization maps by fMR imaging can have a complex relationship to positive stimulation points using direct cortical stimulation in the operating room. The performance of fMR imaging is influenced regionally by the effects of tumor and other pathology on vasculature and the coupling of vascular responses to neural activity. Similarly, the fractional anisotropy measured by diffusion tensor imaging is regionally influenced by edema, whereas the 3-dimensional (3D) tractography map is dependent on the computational model used. This article reviews several imaging modalities that are increasingly used in neurosurgical planning. As these techniques are progressively applied to surgery, radiologists, medical physicists, neuroscientists, and engineers will be necessary partners with the treating neurosurgeon to bridge the gap between the experimental and the therapeutic.
Intraoperative mapping and monitoring
Surgical applications of advanced imaging are best considered in the context of standard practice and the neurosurgical “gold standards” for functional localization, which are based on intraoperative neurologic assessment and electrophysiology. These traditional forms of mapping are performed in patients awake under local anesthesia or, in certain circumstances, under light general anesthesia. During awake procedures patients may be monitored continuously as resection approaches functionally eloquent territory—that is, territory in which a focal injury leads to an conspicuous loss of function.
Function can be assessed intraoperatively by direct electrical stimulation of exposed cortical and white matter regions (electrocortical stimulation, ECS). ECS is generally performed with a dual electrode (bipolar) stimulator that limits current spread to less than 1 cm (see Matz and colleagues for a good review of the direct cortical stimulation protocol). ECS may elicit a positive motor sign that is observed directly (eg, hand clench or vocalization) or detected by electromyography (EMG). ECS stimulation may also elicit a negative functional sign or loss of function. Examples are speech arrest, word finding, paraphasias, or perseveration detected while the patient is engaged in an active task. Of course, stimulation at a particular site may elicit neither positive nor negative responses even after escalating to the maximal safe current. In that case, the surgeon must consider anatomy and the effects of the underlying pathology to decide whether this “negative result” signifies a safe region to operate through.
Electrocorticography (ECoG) is a related technique that can be used for sensorimotor mapping in sedated or noncooperating patients. Somatosensory evoked potentials (SSEP) are used to attempt localization of the central sulcus. In this technique, a stimulating electrode is placed on the wrist overlying the median nerve and an evoked potential is recorded from the strip electrode oriented perpendicular to the presumed rolandic gyri. The phase of the evoked waveform is “flipped” between electrodes in the frontal and parietal lobes, localizing central sulcus at the reversal point. SSEPs may be distorted or absent in the presence of underlying tumor, limiting the reliability of the technique.
Fig. 1 shows an example of awake language and motor mapping guided by preoperative fMR imaging. Integration of functional and anatomic features in a neuronavigational system allows a reassuring registration of the expected picture with surface anatomy on craniotomy. During intraoperative mapping, sterile paper markers are placed on the cortex at positive stimulation points where behavior was evoked or interrupted by the bipolar stimulation. Note that after the strip electrode is used to establish somatosensory evoked potentials, it remains positioned on the cortex for seizure monitoring (electrocorticography) during bipolar stimulation and throughout the resection. In usual practice, a distance of 1 to 3 cm between the positive stimulation point and the surgical resection margin is respected to avoid deficit.
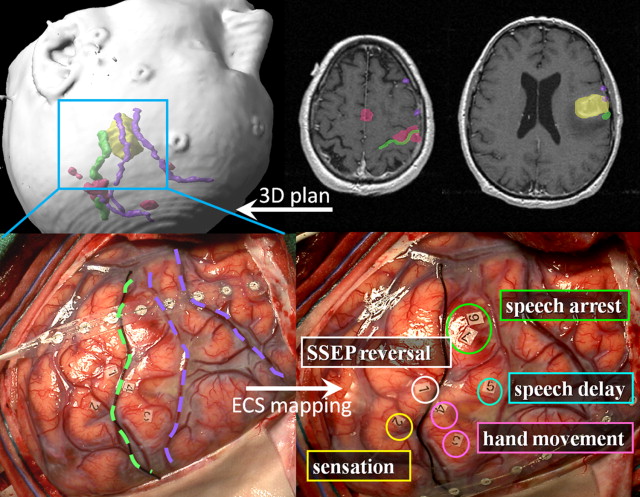
There are several technical limitations of functional mapping by bipolar stimulation. Cortical mapping is restricted to the gyral surface; sulcal function cannot be assessed. Some cortical regions are inconvenient for mapping. For example, lesions that impinge on the precentral motor area for the foot, located in the interhemispheric fissure, can be difficult to access with a cortical stimulator. Cortical sensitivity to stimulation (ie, the threshold current required to elicit responses) varies regionally and may be affected by the level of anesthesia and the effects of tumor and related edema. Stimulation may elicit pathologic afterdischarges or frank seizures that can limit or prevent further mapping. Even when cortical structures are successfully spared, white matter injuries can be devastating. In fact, it has been suggested that white matter injuries may result in more profound and permanent functional deficits than gray matter injuries. Although subcortical bipolar stimulation is becoming more common, it is technically more difficult and is not routinely performed.
Intraoperative mapping has practical limitations as well. Most awake mapping relies on the concerted participation of the patient; an exception is motor mapping by passive observation of movement and/or EMG. Patients can have difficulty awakening from anesthesia, and can be uncooperative or perform so variably that mapping is no longer reliable. In the authors’ experience, preoperative deficits often are exacerbated by anesthesia and patients with preoperative deficits can require a longer “wake-up” period to return to baseline performance before mapping. Furthermore, time for functional mapping in the operating room is limited and a balance must be achieved between a complete mapping, with a varied battery of tasks and the time necessary to perform them.
In light of these limitations of intraoperative mapping, preoperative functional imaging offers potentially more complete functional mapping, acquired under controlled conditions. It is important that these results are available before surgery to assess risk, inform discussion with the patient, and aid in surgical planning. In this article the authors consider the use of advanced imaging in brain tumor surgery. Specifically, the discussion focuses on fMR imaging, diffusion tensor imaging (DTI), and iMR imaging, and to what extent they affect treatment planning.
Functional MR imaging
The most common application of fMR imaging is to assess risk in patients with lesions in or near language and motor areas. Functional mapping by MR imaging can be done by several techniques. Blood oxygen dependent functional magnetic resonance imaging (BOLD fMR imaging) is most commonly used and is described more completely by Gupta and colleagues in the article on fMR imaging and DTI elsewhere in this issue. The BOLD signal itself measures differences in magnetic susceptibility between oxy- and deoxyhemoglobin, the ratio of which varies in relation to cortical blood flow and tissue oxygen use. During task performance, cortical regions that experience increased neuronal activity see increased oxyhemoglobin concentrations relative to the resting baseline. This phenomenon is due to (and dependent on) increased perfusion with oxygenated blood resulting from active arteriolar dilation. The observation of increased regional perfusion with neural activity was first made in humans by the neurosurgeon Wilder Penfield, who noted a red shift in the color of temporal cortical veins during an intraoperative seizure. The shift in oxygenation is detectable by a T2 pulse sequence from which quantitative comparisons for task related neural activity can be made.
Cortical activation leads to relatively small BOLD changes of 1% to 2% for most regions and tasks, though sensorimotor tasks can elicit stronger signals from rolandic cortex. As previously described, neurovascular uncoupling is a concern for brain regions affected by tumor burden, prior surgery, or radiation: the arterioles may be maximally dilated at baseline as a result of pathology. Weaker BOLD signals are susceptible to scanner artifacts and head motion, and this can lead to false-negative predictions. Arguably the single greatest concern when applying fMR imaging results in surgery is managing the risk of acting on a false-negative result. Therefore, significant attention has been directed at validating fMR imaging and understanding the modes of failure.
Functional MR Imaging Concordance with Direct Electrocortical Stimulation
One-way fMR imaging is evaluated for neurosurgical planning is by testing concordance with intraoperative direct cortical stimulation. To date, fMR imaging has shown varied degrees of agreement with direct cortical stimulation depending on the modality being tested. fMR imaging localization of the motor gyrus has achieved very good concordance in correlative studies. In fact, in the authors’ experience fMR imaging of the primary motor gyrus has been more reliable than intraoperative SSEP measurements in patients with peri-rolandic tumors. Krings and colleagues, in an investigation of 103 patients (194 functional scans), found that fMR imaging was able to correctly identify the motor gyrus in 85% of cases. fMR imaging failed to localize any functional motor information in 30 fMR imaging scans. In 11 patients no functional activation was seen in any motor scan performed. The investigators concluded that fMR imaging failure in these cases was predominantly a result of head motion. Roux and colleagues found that although fMR imaging overestimated the spatial extent of a cortical area required to elicit a positive motor response, it was highly concordant with ECS in brain tumor patients with various pathologies.
The experience of the authors in brain tumor patients supports that despite tumor-induced sensitivity loss of the BOLD fMR imaging signal, the motor gyrus can be identified correctly if the fMR imaging study is technically successful (ie, rolandic activation is seen). In practice, fMR imaging prediction of central sulcus is considered together with anatomic predictions. Interrater agreement on the location of central sulcus based on anatomic MR imaging alone in healthy volunteers has been reported to be low (76%) and would be expected to diminish in cases with rolandic tumor distorting anatomy. Fig. 2 shows a typical case of central sulcus localization by BOLD fMR imaging. The lesion (glioblastoma multiforme, GBM) is imposing significant mass effect and is effacing the central sulcus, making functional localization by anatomic markers alone difficult. fMR imaging localizes the motor gyrus in this case and places the majority of the lesion posterior to the primary motor gyrus. Surgical resection within or through the sensory cortex of the postcentral gyrus is far less likely to cause weakness than within the precentral gyrus. It is often useful to perform multiple motor paradigms to activate different portions of the motor homunculus using a tongue motor (inferolateral aspect of the motor gyrus), hand motor (lateral aspect), and foot motor (medial aspect) task. A typical finger-tapping paradigm can activate premotor, primary motor, and postcentral (sensory) cortices simultaneously, making motor localization sometimes difficult using a single fMR imaging task.
Concordance with Language Function
Compared with the sensorimotor system, language function is more broadly distributed and language tasks elicit smaller BOLD signals overall than do motor tasks. Intraoperative mapping by ECS is limited by the time and patient cooperation required to accurately detect subtle language errors across a panel of tests. Not surprisingly, fMR image mapping appears overall to be less concordant with ECS for language function. Some forms of discordance are systematic, and for these it is useful to investigate why the functional study failed to predict intraoperative findings.
Sometimes the failure of concordance between fMR imaging and direct cortical stimulation is traceable to a mismatch between MR imaging and intraoperative testing paradigms. One of the main behavioral signs used during intraoperative ECS to localize essential expressive language territory is speech arrest. fMR imaging activation during covert (nonverbal) expressive language tasks might extend over broad territory, but would be expected to include these sites of intraoperative speech arrest. In a study of patients undergoing awake language mapping, it was found that silent speech fMR imaging correctly predicted sites of language disturbance with ECS in the inferior and middle frontal gyri but failed to predict observed sites of speech arrest in the precentral motor gyrus. In normal controls, vocalized speech not only enlisted the classical Broca’s area (in the inferior frontal gyrus) but also the inferior aspect of the motor gyrus. Because interruption of vocalized speech (speech arrest) is a principal finding during ECS mapping, it follows that having the patient perform at least one fMR imaging speech task aloud should support better concordance between the testing protocols. However, vocalized speech paradigms are challenging to employ for fMR imaging, as they require the subject to keep his or her head still while speaking. This rigidity requires a level of concentration that may be unsustainable for sick patients. Head motion can degrade the study and can lead to false positives if motion is correlated with the stimulus.
Functional MR Imaging Determination of Hemispheric Dominance for Language
The left hemisphere is the dominant seat of language in nearly all right-handed patients as well as the majority of left-handed patients. It is important in neurosurgical practice to be able to identify the exceptions: patients with language dominance in the right hemisphere or shared between hemispheres (“codominant”). The intracarotid amobarbital test (or Wada test) has historically been the gold standard test to determine which hemisphere predominantly controls language (language laterality). This test employs transient anesthesia of each hemisphere separately to determine language laterality. Language lateralization using fMR imaging has achieved good but imperfect concordance with the Wada procedure. Reasons for the discordance are multifaceted depending on whether Wada or fMR imaging predicted language dominance correctly. For example, in epilepsy patients concordance between fMR imaging and the Wada test has been shown to reach 91%. Because fMR imaging laterality measures are based on quantitative comparisons, they may be particularly sensitive to subtle effects of neurovascular uncoupling on the pathology-bearing side. Ulmer and colleagues found that of 85 functional areas tested (in 50 patients with brain lesions), 23 (27%) areas showed perilesional (within 5 mm) decreases in fMR imaging signal. This finding led to incorrect measurements of dominance. Patients with previous surgery are another cohort at risk of false-negative fMR imaging signals as a result of signal dropout imposed by blood products and surgical staples. This risk may be reduced by using a hemispheric region of interest to calculate language laterality in order to minimize regional susceptibility artifacts. Increasing refinement of laterality measure by fMR imaging will hopefully lead to standards by which studies can be made comparable and quality measures can reduce the risk of false prediction.
In clinical practice, all patients with left-sided lesions in potential language areas are considered for awake mapping because the majority will be left-dominant. fMR imaging measures of hemispheric dominance are most valuable when they indicate unexpected or atypical results that are clinically actionable. For example, when fMR imaging indicates right hemispheric dominance or split dominance, either a Wada test can be ordered or the potentially translocated/reorganized area in question can be mapped intraoperatively. These cases are uncommon. More typically, in the authors’ experience brain tumor patients exhibit either the expected left hemisphere laterality on fMR imaging or varying degrees of bilateral activation, depending in part on how the study is processed.
The functional significance of bilateral fMR imaging activation is not yet clear and as a result, it is not prudent to infer functional codominance without confirmatory testing. A study by Knecht and colleagues investigated transcranial magnetic stimulation (TMS) to validate the fMR imaging measurement of hemispheric dominance. TMS uses extracranial magnetic pulses during speech production tasks to map function much the same way direct electrocortical stimulation is employed in the operating room. This study tested control subjects with varying degrees of putative hemispheric dominance predicted by fMR imaging laterality (completely left dominant, codominant, and completely right dominant). In individuals with bilateral fMR imaging activation, TMS identified speech interruption sites in both hemispheres more often than for well-lateralized counterparts. However, whether this pattern of speech distribution provides any protection against surgical injury is unknown.
Unlike invasive mapping techniques, fMR imaging is well suited to fine-mapping language codominance or split dominance and to follow changes in the dominance pattern over time. In rare cases, split dominance appears to be consistent with cortical compensation and/or translocation of function in the context of progressive injury from tumor growth and treatment.
Issues with Functional MR Imaging Interpretation for Surgical Use
In addition to the problem of neurovascular uncoupling, the interpretation of BOLD fMR imaging in brain tumor patients suffers from 2 other central issues: thresholding and essential versus nonessential function. These issues, in turn, affect the way the fMR imaging map is trusted in the operating room.
Thresholding refers to the choice of statistical cutoff applied during the analysis, which affects the apparent pattern of a functional activation. If the statistical significance is lenient, the functional activation is more extensive and includes a higher chance of false positives; stringent thresholds risk false-negative calls. There is no single optimum statistical threshold that can be applied patient to patient. BOLD signal, task performance, artifacts, and noise vary individually, regionally, and from study to study.
There have been many attempts to address the thresholding problem. Voyvodic and colleagues proposed a method to localize the motor strip using the most statistically active voxels, achieved by normalizing statistical t -test maps to peak amplitudes of voxels within functional regions. Another study has similarly suggested using the first appearance of a fixed number of active voxels as a more stable measure of language laterality than the traditional statistical thresholding. All things considered, thresholding is a weakness in laterality measurements and localization of weak BOLD signals, and the clinician should be mindful when interpreting fMR imaging data.
It is surprising that although the BOLD phenomenon is well established, the relationship between BOLD signal and the underlying cortical circuit—that is, what kind of activity BOLD signal is measuring—is not at all clear (see review by Logothetis for sobering detail). Among the complications to consider are that inhibitory neurons outnumber excitatory neurons in the central nervous system and their function adds to metabolic demand; and that synaptic potentials (representing, in part, cortical input) lead to far larger ion fluxes and metabolic demand than action potentials (representing output). Even when a stimulus is known to generate cortical activation and excitatory output from a particular region, there is no general rule regarding the neurologic effects of injury to that region. For instance, fMR imaging activation of the motor strip in a finger-thumb tapping task is associated with cortical output and predicts weakness if the area is injured. However, activation of Heschl’s gyrus in an auditory-driven task is also associated with cortical output and yet injuries to this site may have no discernible effects. Therefore, even with “true positive” fMR imaging activation, it is impossible to know which areas are essential versus supportive for the function tested. This issue is particularly relevant in neurosurgical planning because only a subset of the fMR imaging localizations, when stimulated with a bipolar stimulator, will actually show interruption of function. Accordingly, fMR imaging (particularly for language studies) is most commonly used as a moderate preoperative estimator of risk and a guide to intraoperative cortical stimulation. In practical terms this use of FMR imaging has been shown to reduce mapping time in the operating room.
Functional MR Imaging and Clinical Outcomes
The historical view of neurosurgical outcomes suggests that new technologies lead to incremental improvements rather than dramatic advances. In light of the cost of preoperative fMR imaging, there is great interest in measuring the effect on clinical outcomes. At present there are only data on intermediate or technical outcomes, such as influence on surgical technique or correlations with neurologic morbidity. Petrella and colleagues, in a case series of 39 patients, found that treatment plans for 19 patients were changed in light of the preoperative functional imaging; they also found that fMR imaging resulted in reduced surgical mapping in 22 patients, a more aggressive resection in 6 patients, and a smaller exposure in 2 patients. A study of patients with seizure disorders concluded that fMR imaging helped avoid further clinical testing (including the Wada test) in 63% of patients. In the same study fMR imaging altered intraoperative mapping procedures in 52% and altered surgical plans in 42%.
The predictive value of fMR imaging for recovery from stroke and other neurologic injuries is also being investigated. A recent study of stroke recovery found that fMR imaging was a significant predictor, along with age and neuropsychological performance, of whether a patient would significantly regain language performance 6 months after the event, together achieving 86% accuracy. Another study found that temporal lobe epilepsy patients with ipsilateral temporal lobe fMR imaging activation versus contralateral activation had greater memory decline following mesial temporal lobe resection. Thus, the value of clinical fMR imaging has the potential to be significant as its use is refined.
Future Directions for Functional Imaging for Presurgical Planning
Arterial spin labeling (ASL) is an alternative approach to fMR imaging, which measures activity-dependent blood flow changes directly by tracking the passage of arterial blood that has been spin-labeled in the carotid artery. The advantages of ASL include higher specificity than BOLD, less vulnerability to head motion, less variability in spatial localizations in test/retest studies, and fewer artifacts from magnetic susceptibility. The major drawback is limited sensitivity (signal magnitudes are between half and a quarter that of BOLD fMR imaging).
Resting state fMR imaging, where very slow fluctuations of the BOLD signal are measured during prolonged resting state, has recently reemerged as an alternative approach to task-driven imaging in clinical applications. The practical advantage is that the behavioral constraints in these studies are lifted because intrinsic network connectivity/integrity is measured while patients are at rest in the scanner. This technique is still in its early stages and will need significant validation before it can be used for neurosurgical planning.
Multimodality mapping is also gaining traction in neurosurgical planning. Complementary techniques like magnetoencephalography add temporal resolution to the BOLD signal. TMS has the potential to validate fMR imaging maps, characterize clinically ambiguous fMR imaging studies, and clarify the functional significance of bilateral language activation by allowing relatively unrestricted stimulation compared with what is available intraoperatively. TMS can achieve spatial precision when used with a stereotactic navigational system, lending information about the essential versus supportive nature of fMR imaging activations preoperatively.
Functional MR imaging
The most common application of fMR imaging is to assess risk in patients with lesions in or near language and motor areas. Functional mapping by MR imaging can be done by several techniques. Blood oxygen dependent functional magnetic resonance imaging (BOLD fMR imaging) is most commonly used and is described more completely by Gupta and colleagues in the article on fMR imaging and DTI elsewhere in this issue. The BOLD signal itself measures differences in magnetic susceptibility between oxy- and deoxyhemoglobin, the ratio of which varies in relation to cortical blood flow and tissue oxygen use. During task performance, cortical regions that experience increased neuronal activity see increased oxyhemoglobin concentrations relative to the resting baseline. This phenomenon is due to (and dependent on) increased perfusion with oxygenated blood resulting from active arteriolar dilation. The observation of increased regional perfusion with neural activity was first made in humans by the neurosurgeon Wilder Penfield, who noted a red shift in the color of temporal cortical veins during an intraoperative seizure. The shift in oxygenation is detectable by a T2 pulse sequence from which quantitative comparisons for task related neural activity can be made.
Cortical activation leads to relatively small BOLD changes of 1% to 2% for most regions and tasks, though sensorimotor tasks can elicit stronger signals from rolandic cortex. As previously described, neurovascular uncoupling is a concern for brain regions affected by tumor burden, prior surgery, or radiation: the arterioles may be maximally dilated at baseline as a result of pathology. Weaker BOLD signals are susceptible to scanner artifacts and head motion, and this can lead to false-negative predictions. Arguably the single greatest concern when applying fMR imaging results in surgery is managing the risk of acting on a false-negative result. Therefore, significant attention has been directed at validating fMR imaging and understanding the modes of failure.
Functional MR Imaging Concordance with Direct Electrocortical Stimulation
One-way fMR imaging is evaluated for neurosurgical planning is by testing concordance with intraoperative direct cortical stimulation. To date, fMR imaging has shown varied degrees of agreement with direct cortical stimulation depending on the modality being tested. fMR imaging localization of the motor gyrus has achieved very good concordance in correlative studies. In fact, in the authors’ experience fMR imaging of the primary motor gyrus has been more reliable than intraoperative SSEP measurements in patients with peri-rolandic tumors. Krings and colleagues, in an investigation of 103 patients (194 functional scans), found that fMR imaging was able to correctly identify the motor gyrus in 85% of cases. fMR imaging failed to localize any functional motor information in 30 fMR imaging scans. In 11 patients no functional activation was seen in any motor scan performed. The investigators concluded that fMR imaging failure in these cases was predominantly a result of head motion. Roux and colleagues found that although fMR imaging overestimated the spatial extent of a cortical area required to elicit a positive motor response, it was highly concordant with ECS in brain tumor patients with various pathologies.
The experience of the authors in brain tumor patients supports that despite tumor-induced sensitivity loss of the BOLD fMR imaging signal, the motor gyrus can be identified correctly if the fMR imaging study is technically successful (ie, rolandic activation is seen). In practice, fMR imaging prediction of central sulcus is considered together with anatomic predictions. Interrater agreement on the location of central sulcus based on anatomic MR imaging alone in healthy volunteers has been reported to be low (76%) and would be expected to diminish in cases with rolandic tumor distorting anatomy. Fig. 2 shows a typical case of central sulcus localization by BOLD fMR imaging. The lesion (glioblastoma multiforme, GBM) is imposing significant mass effect and is effacing the central sulcus, making functional localization by anatomic markers alone difficult. fMR imaging localizes the motor gyrus in this case and places the majority of the lesion posterior to the primary motor gyrus. Surgical resection within or through the sensory cortex of the postcentral gyrus is far less likely to cause weakness than within the precentral gyrus. It is often useful to perform multiple motor paradigms to activate different portions of the motor homunculus using a tongue motor (inferolateral aspect of the motor gyrus), hand motor (lateral aspect), and foot motor (medial aspect) task. A typical finger-tapping paradigm can activate premotor, primary motor, and postcentral (sensory) cortices simultaneously, making motor localization sometimes difficult using a single fMR imaging task.
Concordance with Language Function
Compared with the sensorimotor system, language function is more broadly distributed and language tasks elicit smaller BOLD signals overall than do motor tasks. Intraoperative mapping by ECS is limited by the time and patient cooperation required to accurately detect subtle language errors across a panel of tests. Not surprisingly, fMR image mapping appears overall to be less concordant with ECS for language function. Some forms of discordance are systematic, and for these it is useful to investigate why the functional study failed to predict intraoperative findings.
Sometimes the failure of concordance between fMR imaging and direct cortical stimulation is traceable to a mismatch between MR imaging and intraoperative testing paradigms. One of the main behavioral signs used during intraoperative ECS to localize essential expressive language territory is speech arrest. fMR imaging activation during covert (nonverbal) expressive language tasks might extend over broad territory, but would be expected to include these sites of intraoperative speech arrest. In a study of patients undergoing awake language mapping, it was found that silent speech fMR imaging correctly predicted sites of language disturbance with ECS in the inferior and middle frontal gyri but failed to predict observed sites of speech arrest in the precentral motor gyrus. In normal controls, vocalized speech not only enlisted the classical Broca’s area (in the inferior frontal gyrus) but also the inferior aspect of the motor gyrus. Because interruption of vocalized speech (speech arrest) is a principal finding during ECS mapping, it follows that having the patient perform at least one fMR imaging speech task aloud should support better concordance between the testing protocols. However, vocalized speech paradigms are challenging to employ for fMR imaging, as they require the subject to keep his or her head still while speaking. This rigidity requires a level of concentration that may be unsustainable for sick patients. Head motion can degrade the study and can lead to false positives if motion is correlated with the stimulus.
Functional MR Imaging Determination of Hemispheric Dominance for Language
The left hemisphere is the dominant seat of language in nearly all right-handed patients as well as the majority of left-handed patients. It is important in neurosurgical practice to be able to identify the exceptions: patients with language dominance in the right hemisphere or shared between hemispheres (“codominant”). The intracarotid amobarbital test (or Wada test) has historically been the gold standard test to determine which hemisphere predominantly controls language (language laterality). This test employs transient anesthesia of each hemisphere separately to determine language laterality. Language lateralization using fMR imaging has achieved good but imperfect concordance with the Wada procedure. Reasons for the discordance are multifaceted depending on whether Wada or fMR imaging predicted language dominance correctly. For example, in epilepsy patients concordance between fMR imaging and the Wada test has been shown to reach 91%. Because fMR imaging laterality measures are based on quantitative comparisons, they may be particularly sensitive to subtle effects of neurovascular uncoupling on the pathology-bearing side. Ulmer and colleagues found that of 85 functional areas tested (in 50 patients with brain lesions), 23 (27%) areas showed perilesional (within 5 mm) decreases in fMR imaging signal. This finding led to incorrect measurements of dominance. Patients with previous surgery are another cohort at risk of false-negative fMR imaging signals as a result of signal dropout imposed by blood products and surgical staples. This risk may be reduced by using a hemispheric region of interest to calculate language laterality in order to minimize regional susceptibility artifacts. Increasing refinement of laterality measure by fMR imaging will hopefully lead to standards by which studies can be made comparable and quality measures can reduce the risk of false prediction.
In clinical practice, all patients with left-sided lesions in potential language areas are considered for awake mapping because the majority will be left-dominant. fMR imaging measures of hemispheric dominance are most valuable when they indicate unexpected or atypical results that are clinically actionable. For example, when fMR imaging indicates right hemispheric dominance or split dominance, either a Wada test can be ordered or the potentially translocated/reorganized area in question can be mapped intraoperatively. These cases are uncommon. More typically, in the authors’ experience brain tumor patients exhibit either the expected left hemisphere laterality on fMR imaging or varying degrees of bilateral activation, depending in part on how the study is processed.
The functional significance of bilateral fMR imaging activation is not yet clear and as a result, it is not prudent to infer functional codominance without confirmatory testing. A study by Knecht and colleagues investigated transcranial magnetic stimulation (TMS) to validate the fMR imaging measurement of hemispheric dominance. TMS uses extracranial magnetic pulses during speech production tasks to map function much the same way direct electrocortical stimulation is employed in the operating room. This study tested control subjects with varying degrees of putative hemispheric dominance predicted by fMR imaging laterality (completely left dominant, codominant, and completely right dominant). In individuals with bilateral fMR imaging activation, TMS identified speech interruption sites in both hemispheres more often than for well-lateralized counterparts. However, whether this pattern of speech distribution provides any protection against surgical injury is unknown.
Unlike invasive mapping techniques, fMR imaging is well suited to fine-mapping language codominance or split dominance and to follow changes in the dominance pattern over time. In rare cases, split dominance appears to be consistent with cortical compensation and/or translocation of function in the context of progressive injury from tumor growth and treatment.
Issues with Functional MR Imaging Interpretation for Surgical Use
In addition to the problem of neurovascular uncoupling, the interpretation of BOLD fMR imaging in brain tumor patients suffers from 2 other central issues: thresholding and essential versus nonessential function. These issues, in turn, affect the way the fMR imaging map is trusted in the operating room.
Thresholding refers to the choice of statistical cutoff applied during the analysis, which affects the apparent pattern of a functional activation. If the statistical significance is lenient, the functional activation is more extensive and includes a higher chance of false positives; stringent thresholds risk false-negative calls. There is no single optimum statistical threshold that can be applied patient to patient. BOLD signal, task performance, artifacts, and noise vary individually, regionally, and from study to study.
There have been many attempts to address the thresholding problem. Voyvodic and colleagues proposed a method to localize the motor strip using the most statistically active voxels, achieved by normalizing statistical t -test maps to peak amplitudes of voxels within functional regions. Another study has similarly suggested using the first appearance of a fixed number of active voxels as a more stable measure of language laterality than the traditional statistical thresholding. All things considered, thresholding is a weakness in laterality measurements and localization of weak BOLD signals, and the clinician should be mindful when interpreting fMR imaging data.
It is surprising that although the BOLD phenomenon is well established, the relationship between BOLD signal and the underlying cortical circuit—that is, what kind of activity BOLD signal is measuring—is not at all clear (see review by Logothetis for sobering detail). Among the complications to consider are that inhibitory neurons outnumber excitatory neurons in the central nervous system and their function adds to metabolic demand; and that synaptic potentials (representing, in part, cortical input) lead to far larger ion fluxes and metabolic demand than action potentials (representing output). Even when a stimulus is known to generate cortical activation and excitatory output from a particular region, there is no general rule regarding the neurologic effects of injury to that region. For instance, fMR imaging activation of the motor strip in a finger-thumb tapping task is associated with cortical output and predicts weakness if the area is injured. However, activation of Heschl’s gyrus in an auditory-driven task is also associated with cortical output and yet injuries to this site may have no discernible effects. Therefore, even with “true positive” fMR imaging activation, it is impossible to know which areas are essential versus supportive for the function tested. This issue is particularly relevant in neurosurgical planning because only a subset of the fMR imaging localizations, when stimulated with a bipolar stimulator, will actually show interruption of function. Accordingly, fMR imaging (particularly for language studies) is most commonly used as a moderate preoperative estimator of risk and a guide to intraoperative cortical stimulation. In practical terms this use of FMR imaging has been shown to reduce mapping time in the operating room.
Functional MR Imaging and Clinical Outcomes
The historical view of neurosurgical outcomes suggests that new technologies lead to incremental improvements rather than dramatic advances. In light of the cost of preoperative fMR imaging, there is great interest in measuring the effect on clinical outcomes. At present there are only data on intermediate or technical outcomes, such as influence on surgical technique or correlations with neurologic morbidity. Petrella and colleagues, in a case series of 39 patients, found that treatment plans for 19 patients were changed in light of the preoperative functional imaging; they also found that fMR imaging resulted in reduced surgical mapping in 22 patients, a more aggressive resection in 6 patients, and a smaller exposure in 2 patients. A study of patients with seizure disorders concluded that fMR imaging helped avoid further clinical testing (including the Wada test) in 63% of patients. In the same study fMR imaging altered intraoperative mapping procedures in 52% and altered surgical plans in 42%.
The predictive value of fMR imaging for recovery from stroke and other neurologic injuries is also being investigated. A recent study of stroke recovery found that fMR imaging was a significant predictor, along with age and neuropsychological performance, of whether a patient would significantly regain language performance 6 months after the event, together achieving 86% accuracy. Another study found that temporal lobe epilepsy patients with ipsilateral temporal lobe fMR imaging activation versus contralateral activation had greater memory decline following mesial temporal lobe resection. Thus, the value of clinical fMR imaging has the potential to be significant as its use is refined.
Future Directions for Functional Imaging for Presurgical Planning
Arterial spin labeling (ASL) is an alternative approach to fMR imaging, which measures activity-dependent blood flow changes directly by tracking the passage of arterial blood that has been spin-labeled in the carotid artery. The advantages of ASL include higher specificity than BOLD, less vulnerability to head motion, less variability in spatial localizations in test/retest studies, and fewer artifacts from magnetic susceptibility. The major drawback is limited sensitivity (signal magnitudes are between half and a quarter that of BOLD fMR imaging).
Resting state fMR imaging, where very slow fluctuations of the BOLD signal are measured during prolonged resting state, has recently reemerged as an alternative approach to task-driven imaging in clinical applications. The practical advantage is that the behavioral constraints in these studies are lifted because intrinsic network connectivity/integrity is measured while patients are at rest in the scanner. This technique is still in its early stages and will need significant validation before it can be used for neurosurgical planning.
Multimodality mapping is also gaining traction in neurosurgical planning. Complementary techniques like magnetoencephalography add temporal resolution to the BOLD signal. TMS has the potential to validate fMR imaging maps, characterize clinically ambiguous fMR imaging studies, and clarify the functional significance of bilateral language activation by allowing relatively unrestricted stimulation compared with what is available intraoperatively. TMS can achieve spatial precision when used with a stereotactic navigational system, lending information about the essential versus supportive nature of fMR imaging activations preoperatively.
Diffusion tensor imaging
fMR imaging and DTI play complementary roles in operative planning. As discussed in this issue by Gupta and colleagues in the article on fMR imaging and DTI elsewhere in this issue, DTI infers the pathway of major white matter tracts by their impact on the directionality and magnitude of water diffusion. Water diffusion in the brain tends to track along bundles of white matter fibers, and the portion of overall diffusion that is directional (as opposed to random) is referred to as fractional anisotropy (FA). The most commonly used representation of DTI is the directionally encoded color FA map. The FA map encodes the magnitude of anisotropy in signal brightness and fiber orientation in 3 standard colors: transverse in red, anteroposterior in green, and craniocaudal in blue. Fig. 3 shows a typical FA map for neurosurgical planning. The FA map can be coregistered with conventional anatomic imaging to provide a preoperative map of functional anatomy and identify major fiber tracts at risk during surgery. In the case shown, preoperative DTI was useful to direct white matter stimulation to the anteromedial margin.
