Figure 5.1 An early example of massive rib injuries in an abused infant.
The presence of rib fractures is now often central to the radiologic diagnosis of child abuse. A review of the older literature found that rib fractures constituted from 5 to 29% of all fractures detected in children surviving assaults upon them (5, 7–13). More recent data indicate that rib fractures are likely the most common skeletal injuries noted in abused children. In a database review of patients drawn from multiple centers, including postmortem material, Boal et al. found fractures in 213 of 303 (70%) child abuse cases (14). Of a total of 2025 fractures, 1608 (79%) involved the rib cage. In a postmortem study, Kleinman and colleagues found that 51% of all fractures in 31 abused infants who died involved the rib cage (15).
Mandelstam and others reviewed the records of 124 children with the discharge diagnosis of nonaccidental injury and found 32 who had undergone both skeletal survey (SS) and bone scintigraphy within 48 hours of each other (16). Twenty four (75%) were under 12 months old and 30 of 32 (94%) were less than 3 years of age. The total number of injuries identified was 124. Rib fractures were the commonest injury and were present in 16 of the 30 children with injuries. Six cases (37.5%) had solitary rib fractures, 50% of which were shown using both modalities and 50% of which were seen by bone scan alone. Their observation that scintigraphy was more sensitive for detecting rib fractures is in keeping with other studies (see below).
In 2008, Kemp and associates published a systematic review of the world literature on skeletal fractures and abuse and found 32 studies meeting their study criteria (17). They performed meta-analysis of seven cross-sectional studies of rib fractures. After exclusion of children who were involved in a motor vehicle crash, documented violent trauma cases, and post-surgical cases, the pooled probability of abuse given a rib fracture was 0.71 (0.42–0.91). They found that all but one study showed that children who had rib fractures from abuse had more fractures than those who had not been abused (17–24). An updated review and other evidence-based reviews have drawn similar conclusions regarding the high association of rib fractures and abuse in young children (25–27).
Karmazyn et al. reviewed the SSs in 930 children below 2 years of age (mean age 6 months) and found fractures in 317 children (34%); 97 (10%) had a total of 423 definite rib fractures (28). Duffy and colleagues reviewed the results of 703 SSs for suspected abuse and found that 76 (10.8%) had fractures, most of which involved the rib cage (29). In a multicenter study of 2049 children undergoing SSs for suspected abuse, Lindberg and others found a total of 250 (12%) cases with rib fracture (30). Barber and others reviewed the results of radiographic SSs in 567 infants with suspected abuse and found a total of 490 rib fractures in 77 (14%) cases (31). Rib fractures accounted for 490/1029 (48%) of the bony injuries noted on combined assessment of the initial and follow-up surveys (N = 121/567). Excluding the skull, the rib was the most common anatomic region to be fractured in their population.
In contrast to long bone shaft fractures, which are often suspected clinically, rib fractures are usually occult, either identified incidentally on a chest radiograph or on SS. In a series reported by Merten and colleagues, only 20% of rib fractures were suspected, in contrast to 57% of extremity fractures (32). Bruising only occasionally overlies the rib fracture. In a study of 192 children with inflicted fractures, Peters and others found bruising near the injury site in only 29/317 (9.1%) of rib fractures, a finding confirmed by others (33–35).
Rib fractures from abuse are infrequently associated with significant intrathoracic injuries, in contrast to documented accident victims where visceral injuries commonly accompany broken ribs (20, 36).
These ubiquitous injuries have even been reported as a result of prenatal abuse. Gee described multiple healing rib fractures in a newborn whose mother had presumably attempted to abort the fetus by banging her abdomen against tables and by falling downstairs (37). Rib fractures in infants and young children are unusual, and any such injury should be regarded with suspicion. When causes such as prematurity, metabolic disorders, skeletal dysplasias and syndromes, motor vehicle and pedestrian accidents, and rare instances of cardiopulmonary resuscitation (CPR)-related injuries are excluded, the great majority of rib fractures in infants and young children are the result of abuse (17–24, 38–39).
The strong association of rib fractures with abuse relates to unique anatomic factors and the type of forces applied to the thorax during violent assaults on infants and young children.
Anatomy
An appreciation of the anatomic relationships of the ribs to the spine, to the sternum, and to one another is helpful in understanding the variety of injuries affecting the rib cage. The rib head articulates with the costal facet of the vertebrae anteriorly; posteriorly the rib tubercle articulates with the transverse process facet (Figs. 5.2, 5.3).
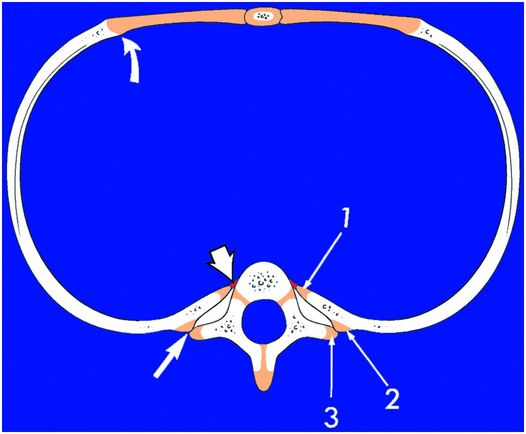
Figure 5.2 Normal rib anatomy. The rib head articulates with the costal facet of the vertebra anteriorly (short white arrow); posteriorly the rib tubercle articulates with the transverse process facet (solid straight arrow). Generous cartilaginous apophyses are noted at the rib head (1), tubercle (2), and transverse process (3). There is slight expansion of the CCJ (curved arrow).
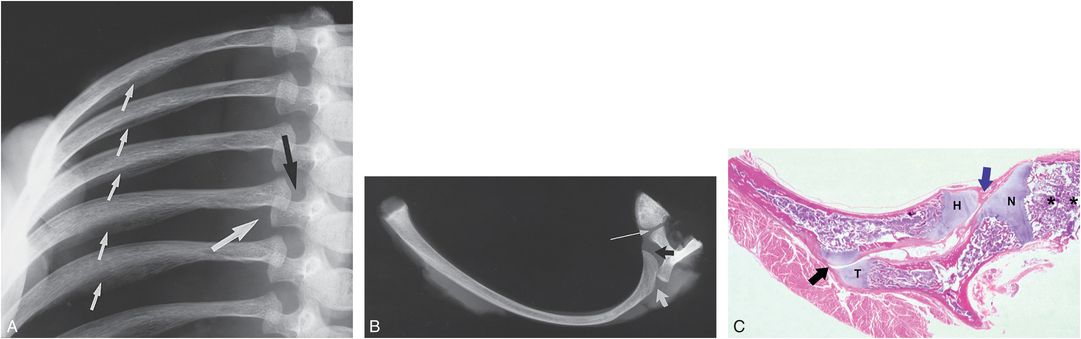
Figure 5.3 Normal rib anatomy: radiologic and histologic correlates. Right ribs with vertebral articulations in a normal two-month-old infant. A, Frontal projection. The rib heads are partially superimposed over the transverse processes. The rib head (black arrow) and transverse process (large white arrow) apophyses are radiolucent. Note the normal, inferiorly flanged appearance of the posterolateral ribs (small white arrows). B, Axial specimen radiograph. The rib head articulates with the vertebral body (black arrow) near the neurocentral synchondrosis (thin white arrow). The rib tubercle articulates with the transverse process posteriorly (short white arrow). Substantial radiolucency is evident at both articulations. C, A corresponding photomicrograph demonstrates the costovertebral (blue arrow) and costotransverse process (black arrow) articulations. Note the generous cartilaginous apophyses of the rib head (H) and the transverse process (T), accounting for the radiographic appearance in A and B. (*) = Vertebral body; N = neurocentral synchondrosis. (From Kleinman PK, Marks SC Jr, Spevak MR, Richmond JM. Fractures of the rib head in abused infants. Radiology. 1992;185:119–23.)
Each rib can be regarded as a lever, with the fulcrum lying at the level of the costotransverse process articulation. This lever mechanism provides the slight gliding movement that accompanies excursion of the rib cage during respiration. The posterolateral rib arc flares inferiorly. This flanged configuration may result in a double cortical contour that can be mistaken for subperiosteal new bone formation (SPNBF) (Fig. 5.3A) (see Chapter 12).
Anteriorly, the first ribs are directly united to the sternum by a synchondrosis. The second to the seventh ribs are attached to the sternum by costal cartilage of progressively increasing length. The ratios of costal cartilage to bone are at their greatest in infancy, diminishing with age. The eighth rib is attached to the seventh by facets and interchondral ligaments, and the ninth and tenth ribs are secured in a similar fashion. The eleventh and twelfth ribs are free floating (40).
Fundamental injury patterns, pathology, and mechanisms
Fractures can occur at any point along the rib arc, from the costovertebral articulations to the costochondral junction (CCJ). The location prevalence along the rib arcs varies depending on the research study design and technique. A particular problem prevalent in the literature is the lack of consistency in how authors divide the rib into anatomic regions. This author has separated rib fractures into those at the costovertebral articulation (head, neck), midposterior, lateral, anterior, and CCJ. Love and associates have recently suggested a somewhat different approach dividing each rib into posterior, posterolateral, anterolateral, and anterior portions (41).
The radiographic detection of rib fractures depends upon the type of radiographic system employed, a factor underlying the recommendation of high-detail imaging systems for radiographic SSs (14, 22, 24, 42–45). Between 33 and 65% of rib fractures in young abused children occur near the costovertebral articulations (14, 15, 45–47). Barsness and associates found that rib fractures involved the posterior arc in 43% of injuries versus 35% and 22% respectively for the mid and anterior arcs (23). Boal et al. found that 736/1463 (50%) rib fractures in their study of abused children had posteriorly situated injuries, and that 479 fractures (33%) occurred at the costovertebral junction (14). Barber and others found that nearly 50% of fractures occurred posteriorly, and these were equally divided between those at the head/neck and those involving the mid arc (Fig. 5.4) (31). Conventional chest radiographs tend to underestimate the number of anterior and posterior rib fractures subsequently found on chest computed tomography (CT). Wootton-Gorges and others found that the number of posterior fractures detected in their population increased from 12 to 39 (P <0.01) and anterior fractures increased from 11 to 42 (P <0.01) when CT supplemented chest radiography (47). Despite the higher sensitivity of CT compared to radiography, in the hands of some investigators, rib fractures detected at autopsy may be still missed with this technique (48).
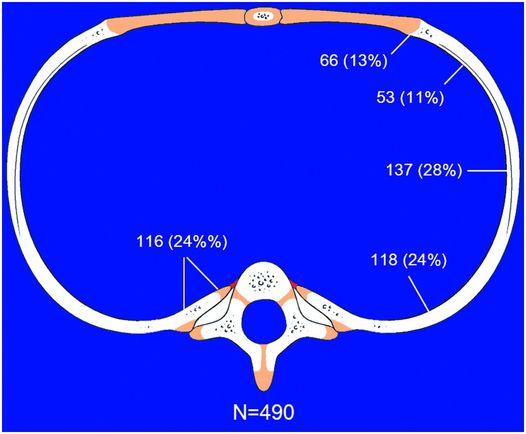
Figure 5.4 Distribution of 490 rib fractures in 77 of 567 infants with suspected abuse. (With permission from Barber I, Perez-Rossello JM, Wilson C, Kleinman PK. The yield of high-detail radiographic skeletal surveys in suspected infant abuse. Pediatr Radiol. 2015;44:69–80.
When rib resection, specimen radiography, and histopathology are employed in fatal cases, the distribution of fractures heavily favors the regions of the costovertebral articulations (Fig. 5.5) (49). Of a total of 84 rib fractures identified by Kleinman et al. in a radiologic–histopathologic study of 31 infant fatalities, 55 fractures (65%) occurred near the costovertebral articulations; 28 involved the rib head, and 27 the rib neck (15). Only 30 (36%) of the rib fractures were noted on SS.
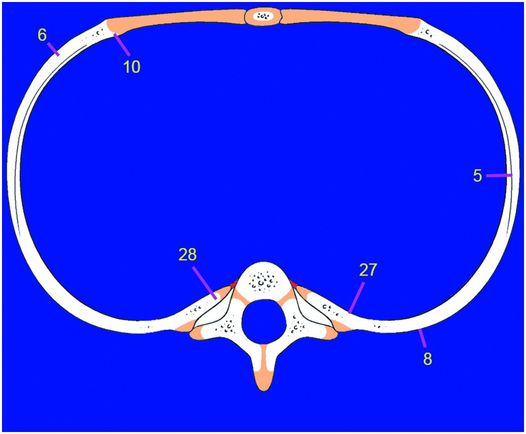
Figure 5.5 Distribution of 84 rib fractures at autopsy in 31 infants dying with evidence of abuse. (With permission from Kleinman PK, Marks SC Jr, Nimkin K, Rayder SM, Kessler SC. Rib fractures in 31 abused infants: postmortem radiologic–histopathologic study. Radiology. 1996;200:807–10.)
Posterior rib fractures
Fractures of the posterior rib arcs are frequently multiple and bilateral (15, 17, 19, 22, 24, 31, 32, 38, 43–45, 47, 49–54). At least half involve the rib head at the costovertebral articulation, or the rib neck near the costotransverse process articulation (Fig. 5.6). Cameron and Rae believed that these fractures occur with lateral compression during violent squeezing of the chest from side to side (50). Smith and colleagues felt that this type of fracture is usually caused when a child is struck from behind or trodden on (55). However, on the basis of clinical observations, as well as radiologic and histopathologic studies, there is little evidence to support these views.
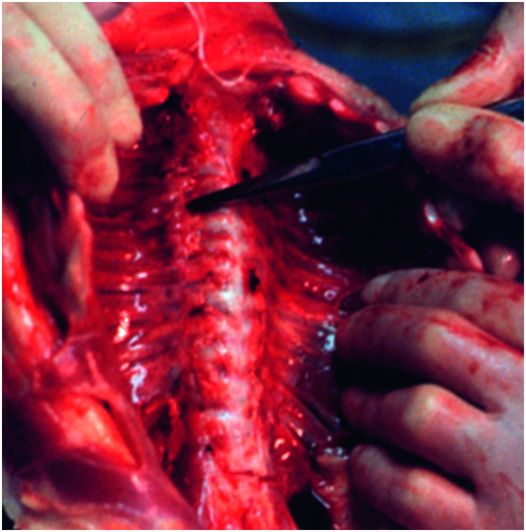
Figure 5.6 Healing fractures at autopsy. Scissor points to multiple healing rib fractures near the right costovertebral articulations in a fatally abused infant.
Analyses of the morphologic alterations associated with these fractures provide a mechanism of injury consistent with anteroposterior (AP) thoracic compression (43, 46, 51, 56–59). Early reports of posterior rib fractures described areas of callus formation along the posteromedial aspects of the ribs (19, 50). The appearance on the frontal radiograph, however, provides little information regarding the relationship between the rib and the vertebral elements. An appreciation of these relationships is fundamental to an understanding of the mechanism resulting in these important fractures.
Rib head fractures
In young infants the rib head is composed primarily of hyaline cartilage and is thus only partially visible radiographically (Fig. 5.3A,B). Histologically, a typical growth plate joins the rib head apophysis with the metaphyseal equivalent of the rib (Fig. 5.3C). The costovertebral articulation is a synovial joint maintained by a fibrous capsule and several strong ligamentous attachments (40). Fractures of the rib head disrupt the ventral bony cortex adjacent to the physis (Fig. 5.7).
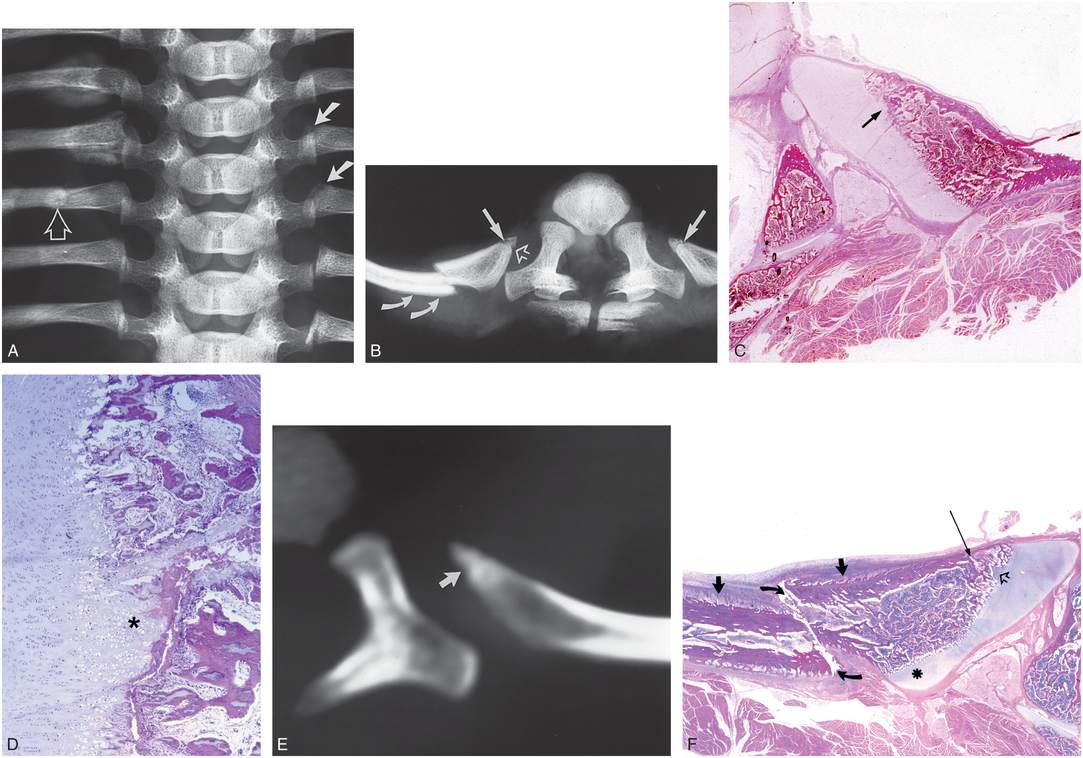
Figure 5.7 Posterior rib fractures in a 2½-month-old abused infant. (See same patient in Fig. 5.9.) A, Specimen radiograph of the sixth-to-tenth posterior ribs demonstrates vague radiolucencies involving the heads of the left seventh and eighth ribs (solid arrows). Also evident are healing fractures at the costotransverse process articulations of the right sixth and seventh ribs. There is a more recent fracture of the neck of the right eighth rib (open arrow). B, Axial specimen radiograph of the eighth ribs and their vertebral articulations. There are bilateral rib head fractures (solid arrows). Note the extension of radiolucency from the rib head apophysis into the fracture site (open arrow). The fracture of the lateral aspect of the rib neck shows posterior displacement with subtle SPNBF (curved arrows). C, A low power photomicrograph of the left eighth rib demonstrates the rib head fracture, with extension of growth plate cartilage into the primary spongiosa (arrow). D, Higher magnification of the area indicated by the arrow in C. Note the localized thickening (asterisk) of the zone of hypertrophic chondrocytes. E, A corresponding axial CT section demonstrates a rib head fracture with extension of low attenuation into the rib head (arrow), corresponding to the focal thickening of the zone of hypertrophied cartilage in C and D. F, Axial histologic section of the right eighth rib corresponding to B. The rib head fracture is noted (long arrow) with associated extension of growth plate cartilage into the primary spongiosa from the rib head apophysis (open arrow). Note also the posteriorly displaced rib neck fracture (curved arrows) just lateral to the cartilaginous rib tubercle (asterisk). Immature SPNBF is seen ventrally (short solid arrows), and dorsally, indicating early healing. (A–E from Kleinman PK, Marks SC Jr, Spevak MR, Richmond JM. Fractures of the rib head in abused infants. Radiology. 1992;185:119–23.)
The fracture line extends posteriorly into the region of the rib head cartilage, undercutting an osseous fragment of variable size. As seen histologically, the fracture runs from the ventral cortex of the rib dorsally into the chondro-osseous junction (COJ). Thus, the fracture conforms to the growth plate injuries described by Salter and Harris (60). Histologic evidence of healing including fibrosis and increased osteoblastic activity. SPNBF and callus formation are occasionally seen. An interesting histologic finding is a localized increase in the thickness of the zone of hypertrophic chondrocytes at the fracture site in most cases (Figs. 5.7C,D). This is the most common histopathologic indicator of healing (51).
Rib neck fractures
The morphologic features of rib neck fractures provide an intuitive understanding of the physical factors responsible for these characteristic inflicted injuries. When rib fractures near the costotransverse process articulation are viewed in axial projections, consistent alterations are evident (Figs. 5.7–5.10). The ventral surface of the rib is invariably fractured and the more extensive injuries show continuation of the fracture line through the medullary cavity to reach the dorsal cortex. Typically, there is distraction of the ventral cortical margins. With healing, SPNBF occurs mainly ventrally and is only occasionally noted dorsally. The fracture lies deep to the transverse process and is inconsistent with a direct blow (contact injury) to the back. Excessive leverage of the rib over the transverse process is a mechanism of injury consistent most with the morphologic features (Fig. 5.10).
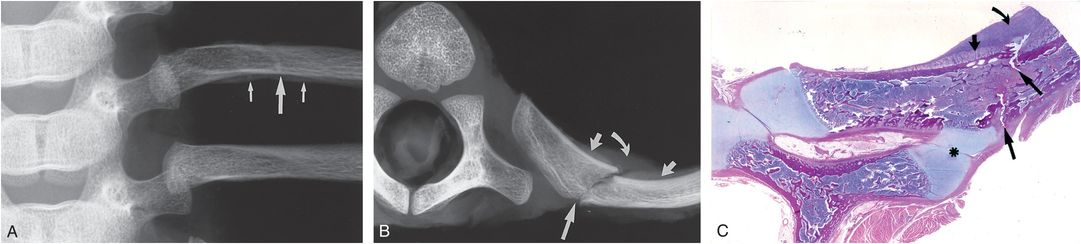
Figure 5.8 Rib neck fracture with early healing in a two-month-old abused infant. (See same patient in 5.62.) A, Specimen radiograph of the left sixth and seventh ribs with vertebral articulations. A faint area of radiodensity crosses the left sixth rib (large white arrow). The fracture site appears to lie well lateral to the level of the transverse process. Note also the fine recent SPNBF (small white arrows). B, An axial specimen radiograph of the left sixth rib shows an oblique fracture through the rib neck (long arrow). Early SPNBF, approximately 7–10 days old, is noted only ventrally (short arrows). Note that the fracture appears to lie well lateral to the costotransverse process articulation. Radiolucent callus (curved arrow) is visible along the ventral (inner) aspect of the rib. C, A corresponding low power axial photomicrograph demonstrates the fracture of the rib neck (large arrows) just lateral to the costotransverse process articulation (asterisk). The apparent lateral location of the fracture with respect to the costotransverse process articulation on the radiograph in A is due to the large radiolucent cartilaginous apophysis of the transverse process. Note the early SPNBF ventrally (short arrow) and the cartilaginous callus (curved arrow).
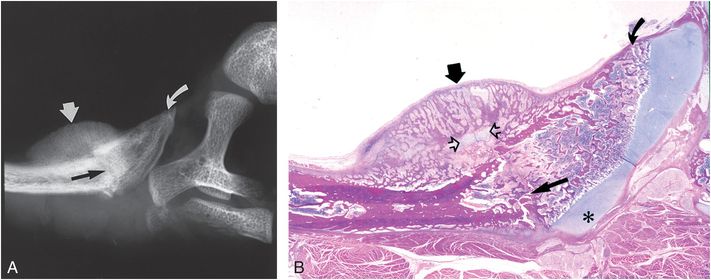
Figure 5.9 Mature healing fracture of the rib neck in the infant shown in Fig. 5.7. A, Axial specimen radiograph of the right sixth rib demonstrates a faint fracture of the rib neck (black arrow) and conspicuous mature callus formation along the ventral aspect of the rib neck (short white arrow). A sclerotic line suggests a rib head fracture (curved arrow). B, Corresponding histologic section demonstrates the neck fracture (long arrow) extending from the ventral cortex to just lateral to the rib tubercle (asterisk). Extensive osseous callus (short arrow) and cartilaginous callus (open arrows) are noted only ventrally. The rib head fracture is confirmed.
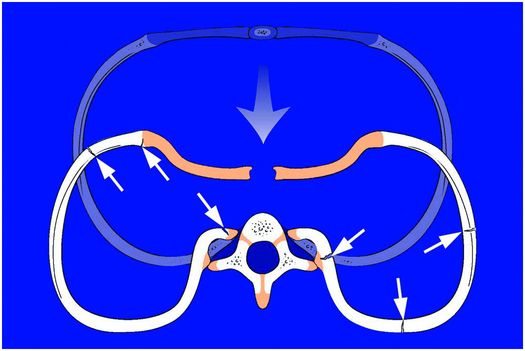
Figure 5.10 Mechanism of rib injury, diagram. With AP compression of the chest, there is excessive leverage of the posterior ribs over the fulcrum of the transverse processes. This places tension along the inner aspects of the rib head and neck regions, resulting in fractures at the costovertebral articulations. This mechanism is also consistent with the morphologic patterns of injury occurring at other sites along the rib arcs and at the CCJ.
A lever is a rigid bar that is free to pivot on a fixed point or fulcrum. The lever arm can be straight, as in a crowbar, or curved, as in a claw hammer. The lever mechanism of the rib is a classic type 1 lever: the fulcrum is situated between the effort (applied force) and the load. Viewing the rib as a lever, the effort is applied to the ventral portion of the rib, which results in leverage of the posterior rib on the transverse process, loading the costovertebral articulation. Assuming that the costovertebral ligaments are stronger than the rib, mechanical failure will occur near the fulcrum (Fig. 5.10).
A 1997 laboratory study in rabbits lends further support to the concept of excessive leverage of the ribs over the transverse processes during thoracic compression (Fig. 5.11) (46). When rabbit thoraxes are gripped with the palms at the side, the fingers at the back, and the thumbs near the midline and the chest is squeezed in the AP plane, excessive leverage of the ribs over the transverse processes occurs (Fig. 5.11D–F). This places maximal mechanical stress at the level of the fulcrum, resulting in rib fracture near the costotransverse articulation. The fracture in this experimental setting is identical to those noted in abused infants. When the chest is compressed on a flat surface, without leverage, no fractures occur (Fig. 5.11A,C). Finite element (FE) modeling of the young infant chest may provide quantitative estimates of the forces required to produce injuries at the costovertebral articulations, as well as fractures elsewhere along the rib arc (61).
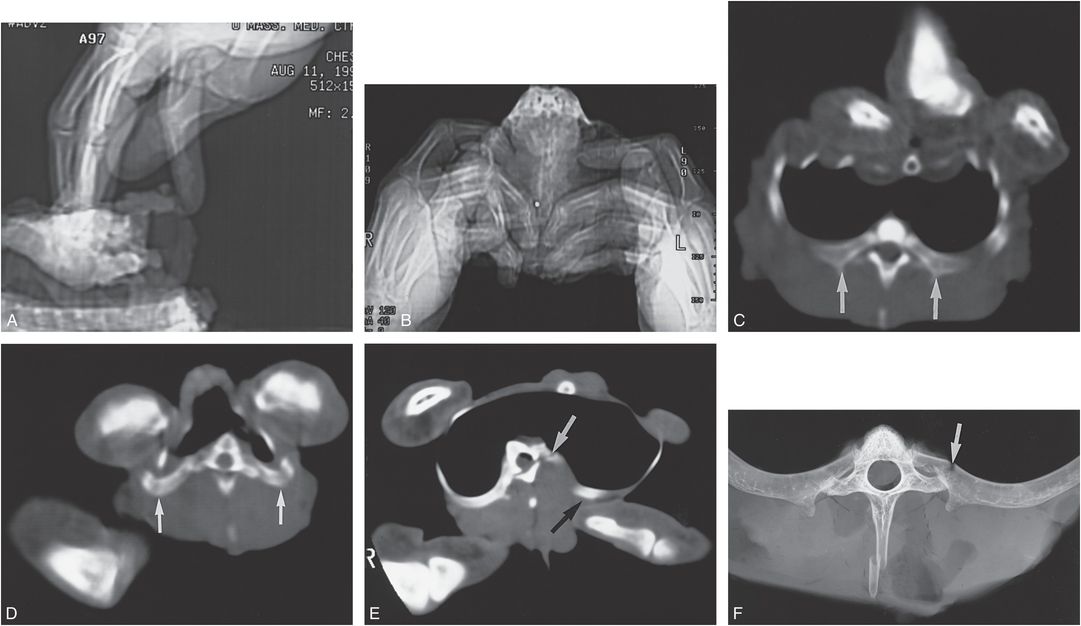
Figure 5.11 Experimental rib fracture study in rabbits. A, Lateral CT topogram with digital compression (as in CPR). B, Frontal CT topogram with manual thoracic compression; the thorax is held with the thumbs in front and the fingers in back. C, Axial CT section with sternal depression on a flat surface (as in CPR). Although the chest is compressed in the AP plane, there is no excessive leverage of the ribs (arrows) over the transverse processes. D, An axial CT section through the upper thorax during bimanual thoracic compression demonstrates posterior leverage of the ribs over the transverse processes (arrows). E, A section through the lower thorax with asymmetric thoracic compression demonstrates excessive leverage of the left posterior ribs over the transverse processes (black arrow) with associated fracture of the left rib neck (white arrow). Relative fixation of the right chest provides a mechanical advantage to the left, resulting in an asymmetric fracture pattern. F, A specimen radiograph shows the fracture (arrow) to lie at the level of the costotransverse process with a pattern identical to that noted in abused infants. (From Kleinman PK, Schlesinger AE. Mechanical factors associated with posterior rib fractures: laboratory and case studies. Pediatr Radiol. 1997;27:87–91.)
Clinical support for this mechanism of injury is provided by the occasional description supplied by assailants in cases of abuse. Figure 5.12 reveals over 30 rib fractures that occurred during a 6-week period in a 6-month-old abused infant. A precise history provided by the abuser described multiple episodes of shaking with AP thoracic compression. The infant was held by the chest with the abuser’s palms situated laterally, the thumbs extended anteriorly to the midline, and the other fingers positioned posteriorly. Compression was from front to back as the infant was shaken in a to-and-fro fashion (see Introduction, Fig. I.1). CT obtained to assess the associated intrathoracic injuries demonstrated multiple fractures of the posterior rib arcs. Figure 5.12C shows several fractures involving the posterior ribs with at least one fracture at the level of the costotransverse process articulation. SPNBF is noted only along the ventral aspects of the ribs.
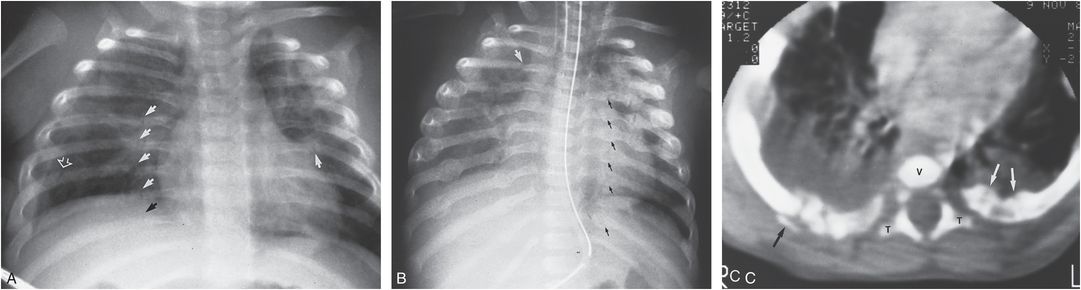
Figure 5.12 Six-month-old abused infant with multiple rib fractures occurring over a six-week period. A, The initial chest film demonstrates multiple recent fractures of the posteromedial aspects of the ribs (solid arrows). There is a suggestion of early healing. A fracture of the posterolateral aspect of the right seventh rib is also evident (open arrow). A small amount of pleural fluid is seen on the right. B, Approximately three weeks later, the rib fractures show advanced healing. Multiple additional fractures are seen involving the posterior and lateral aspects of the ribs, not demonstrable on the original film. Note in particular the fractures in the region of the costovertebral articulations (black arrows). An acute fracture is present involving the right posterior third rib (white arrow). The child was not in protective custody between the initial and subsequent film. C, Axial CT scan through the mid-thoracic region. Two fractures are noted at and just lateral to the left costotransverse process articulation. Note that the SPNBF occurs only ventrally (white arrows). On the right there is a comminuted fracture with SPNBF ventrally. A small bone fragment is displaced posteriorly, but there is no adjacent SPNBF (black arrow). Note the pleural fluid on the right and parenchymal disease on the left. V, vertebral body; T, transverse processes.
Thus, the basic morphologic features, biomechanical analysis, and clinical studies provide compelling support for the concept of AP thoracic compression of the chest as the causative mechanism of posteromedial rib fractures. In most instances the fractures appear to result from manual thoracic compression as the child is gripped and possibly shaken. However, another mechanism could generate similar forces if the infant were slammed face down, or hurled face forward into a solid surface. With this scenario, the posterior rib arc is free to migrate posteriorly, resulting in excessive leverage over the transverse processes. This mechanism has been invoked to explain posteromedial rib fractures occasionally noted when young children are involved as passengers or pedestrians in high-force motor vehicle accidents (MVAs) (46, 62).
Midposterior rib fractures
More laterally situated posterior rib fractures are typically noted with similarly aged fractures of the rib neck in adjacent or contralateral ribs, and are thus explainable by a similar mechanism of injury. Even the twelfth rib, which has a free-floating anterior margin, can be fractured by manual leverage over the transverse process (Fig. 5.13). However, midposterior rib fractures are also seen with other injury mechanisms, such as birth trauma (see Chapter 11), and, therefore, fractures in these regions do not have a single causative mechanism.
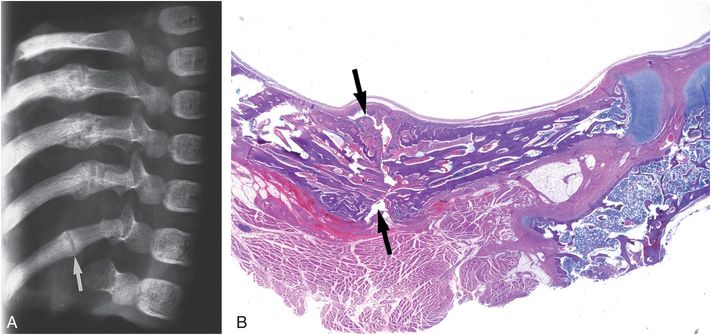
Figure 5.13 Midposterior arc fracture of the twelfth rib in a four-month-old abused infant. A, An oblique specimen radiograph of the right eighth-to-twelfth ribs demonstrates fractures through the posterior arcs of the ninth-to-twelfth ribs (arrow) with advanced healing. B, A photomicrograph of the twelfth rib demonstrates a fracture through the midposterior arc (arrows) with surrounding callus formation. Given the similar ages of all these fractures, it is likely that they occurred with the same mechanism of injury (i.e., excessive posterior leverage of the ribs over the transverse processes).
Lateral and anterior rib fractures
Beyond the level of the transverse processes, the rib arcs are exposed and fractures may occur from direct blows by a hand, foot, or other blunt object. However, the tendency for these fractures to occur at multiple levels at similar points along the arcs of adjacent ribs supports thoracic compression as the usual mechanism of injury. An examination of the morphologic features adds further support to indirect compressive forces rather than direct blows as causative factors in most cases (Figs. 5.14–5.16) (49). Specimen radiography in the axial projection, in conjunction with comparable histologic sections, typically shows buckling or impaction of the inner cortical margin of the rib (Figs. 5.14B, 5.15B,C, 5.16C,D). When the fracture extends to the outer cortex, the fracture margins may be distracted. The findings are analogous to buckle and greenstick fractures of the extremities in which a bending force results in impaction along the concave margin and distraction along the convex margin of the bone. In the rib cage, an external compressive force produces maximal tension along the inner cortex of the rib, leading to fracture. As with posteromedial fractures, thoracic compression with manual squeezing of the rib cage or an impact as the infant is slammed or thrown against a solid surface is consistent with the morphologic findings.
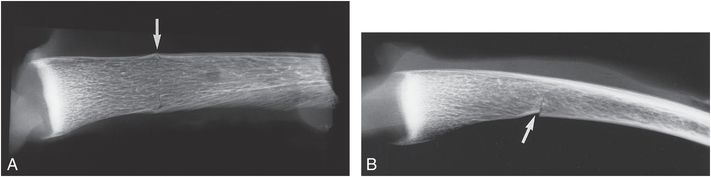
Figure 5.14 Acute anterolateral rib fracture in a four-month-old abused infant. A, A frontal specimen radiograph demonstrates a faint vertical radiolucency with slight buckling of the superior rib margin (arrow). This fracture was not evident on the SS. B, An axial specimen radiograph shows a fracture through the inner margin of the rib (arrow) with impaction at the fracture site. The fracture pattern is consistent with an inward bending of the anterior rib during thoracic compression (see Fig. 5.10). Note the slightly expanded appearance of the CCJs as the cortex extends to the growth plate.
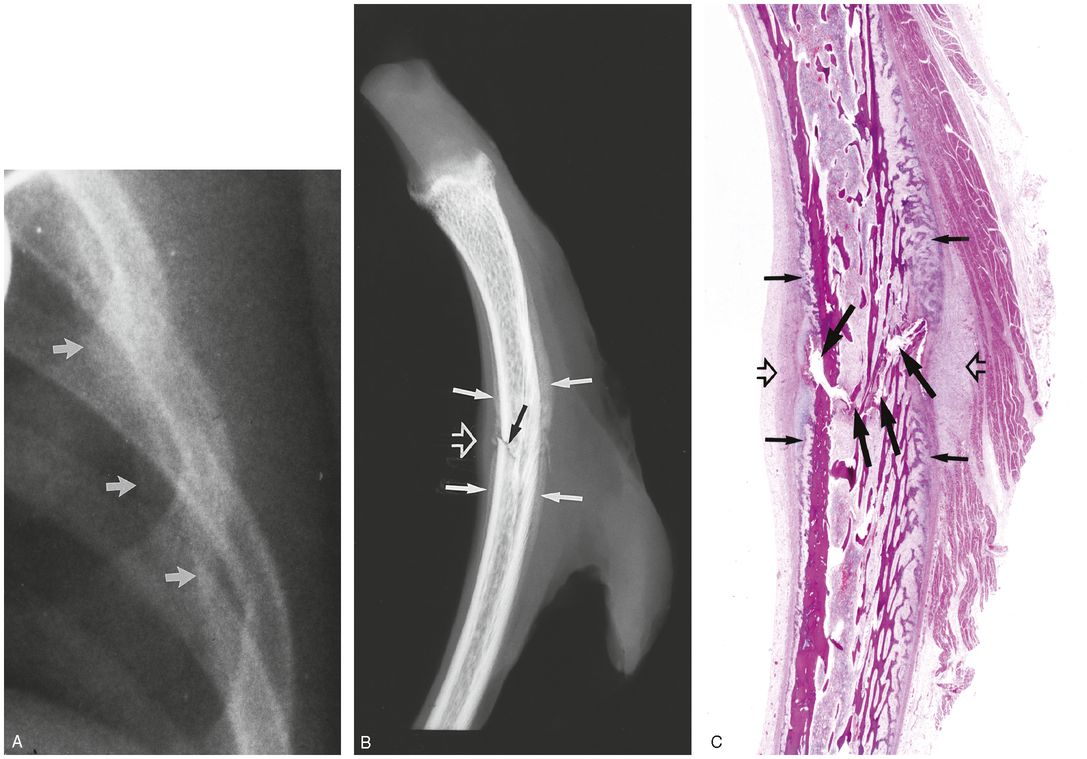
Figure 5.15 Healing lateral rib fracture in a two-month-old abused infant. A, View of the left lateral ribs from a chest radiograph obtained in the ICU demonstrates an extrapleural density along the left fourth, fifth, and sixth ribs (arrows). B, An axial specimen radiograph of the left fifth rib shows an impacted fracture along the inner aspect of the rib (black arrow). SPNBF is seen adjacent to the fracture site (solid white arrows). Soft tissue swelling is noted at the fracture site (open arrow), corresponding to the extrapleural density on the chest film in A. C, A corresponding histologic section demonstrates the fracture site (large arrows) and SPNBF (small arrows). Areas of organizing hematoma without substantial cartilaginous or bony callus are present (open arrows). (From Kleinman PK, Marks SC Jr, Nimkin K, Rayder SM, Kessler SC. Rib fractures in 31 abused infants: postmortem radiologic–histopathologic study. Radiology. 1996;200:807–10.)
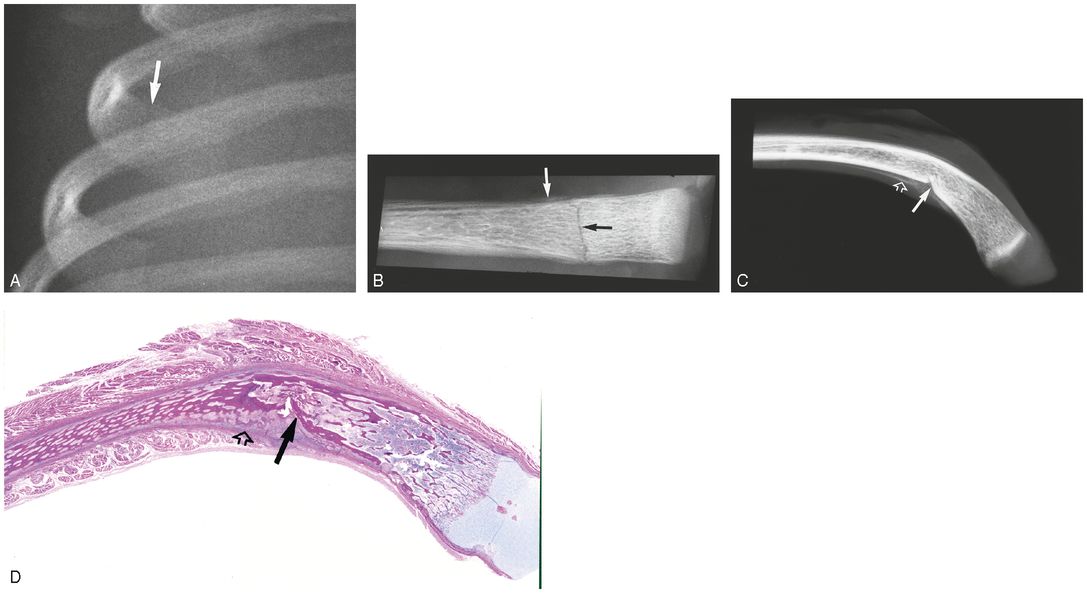
Figure 5.16 Recent anterior rib fracture in a one-month-old abused infant. (See same patient in Figs. 2.19, 5.17, 5.54, 5.57.) A, A high-detail radiograph of the right ribs at the time of autopsy demonstrates a faint radiolucency extending through the anterior aspect of the right fourth rib (arrow). B, A frontal specimen radiograph shows the fracture line (black arrow) and a minimal amount of SPNBF (white arrow). C, An axial specimen radiograph demonstrates impaction of the inner aspect of the fracture site (solid arrow) and minimal SPNBF (open arrow). Note the inward angulation of the distal rib margin. D, A corresponding histologic section demonstrates impaction of the fracture site (solid arrow) and minimal SPNBF (open arrow). Again note the inward angulation of the anterior portion of the rib. The pattern is consistent with thoracic compression and inward bending of the anterior rib margins.
CCJ fractures
Fractures near the CCJ received scant attention in the earlier child abuse literature (50, 63, 64). With radiologic–pathologic correlations, and the increased use of high-detail radiography with oblique radiography and chest CT, these have become well-recognized injuries (14, 31, 44, 45, 47, 49, 65).
The features of CCJ fractures are closely linked to the regional anatomy. In infants and children, a growth plate is present between the osseous anterior rib end and the costal cartilage (see Fig. 5.3). The histologic features are similar to those of growth plates elsewhere and, thus, the bony rib end can be viewed as a metaphyseal equivalent. A 1996 radiologic–histologic study demonstrated similarities between CCJ fractures and injuries involving the metaphyses of the long bones (Fig. 5.17) (49).
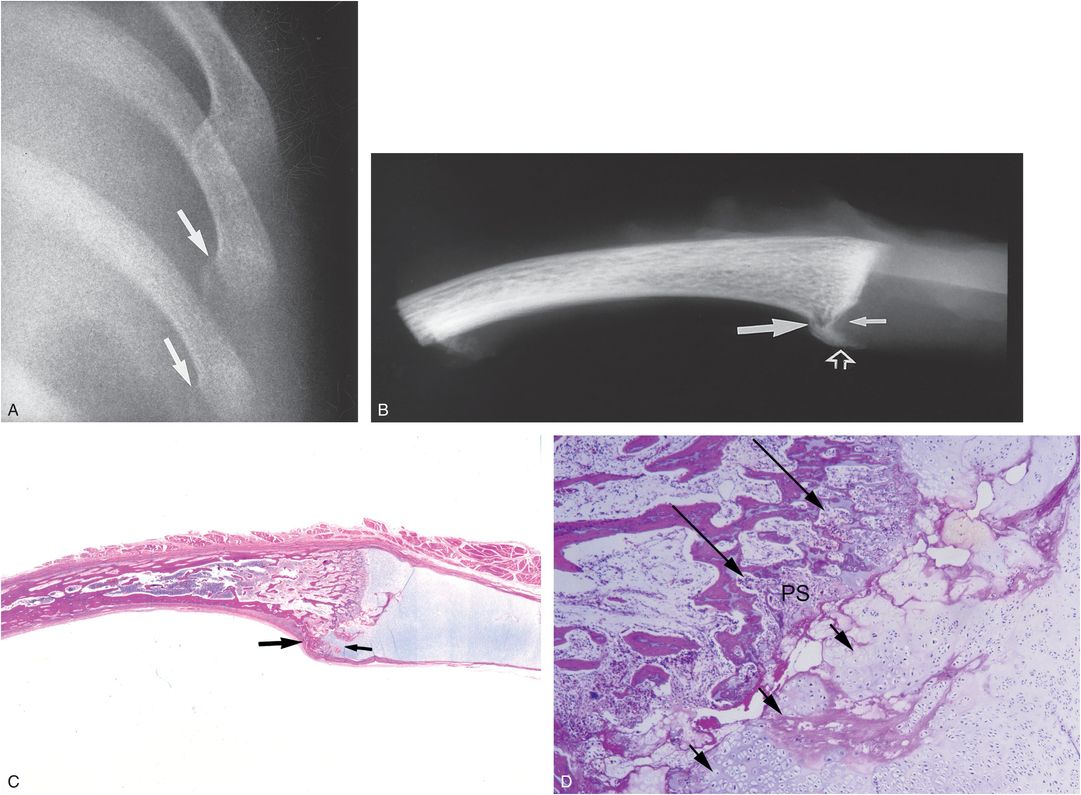
Figure 5.17 CCJ fracture in a one-month-old abused girl. (See same patient in Figs. 2.19, 5.16, 5.54, 5.57.) A, AP radiograph of the left ribs at the time of autopsy demonstrates fracture lines extending through the distal portions of the left eighth and ninth ribs (arrows). B, An axial specimen radiograph of the left eighth rib shows a fracture along the posterior margin of the CCJ (large arrow). Extension of radiolucency of the costal cartilage into the fracture site is noted (small arrow). Note the conspicuous fracture fragment (open arrow). C, A corresponding low power photomicrograph demonstrates the posterior fracture fragment (large arrow) with extension of cartilage into the fracture site (small arrow). D, A high power photomicrograph of the area indicated by the small arrow in C demonstrates disruption of the osseous and cartilaginous architecture at the COJ (long arrows). Note the extension of hypertrophic chondrocytes into the primary spongiosa (PS) (short arrows). The features are consistent with a force that depresses the sternum and/or costal cartilage and impacts the CCJ posteriorly. (From Kleinman PK, Marks SC Jr, Nimkin K, Rayder SM, Kessler SC. Rib fractures in 31 abused infants: postmortem radiologic–histopathologic study. Radiology. 1996;200:807–10.)
Axial specimen radiography of these fractures shows asymmetric involvement, with the greatest bony disruption occurring along the posterior margin of the rib. The resultant fragment may be displaced inward (Fig. 5.17B). Additionally, the periosteum along the inner aspect of the rib may be stripped, and SPNBF may be visible posteriorly. Histologically, osseous disruption is noted mainly posteriorly, and with healing an extension of hypertrophic chondrocytes into the fracture margin correlates with an extension of radiolucency from the costal cartilage into the primary spongiosa (Fig. 5.17C,D).
These pathologic features are consistent with a force that depresses the sternum and costal cartilage, impacting the CCJ posteriorly. A direct focal blow would be expected to produce superficial bony disruption, rather than the deep injuries noted pathologically. Thus, depression of the sternum and/or costal cartilages during manual thoracic compression appears to explain CCJ fractures (see Fig. 5.10).
First rib fractures
Fractures of the first rib are unusual, and inferences regarding mechanism have been drawn from accidental injuries reported in a variety of settings (65). Both direct impact and compressive mechanisms have been described with accidents in older children and adults. Fractures of the posteromedial first ribs can be seen with posteromedial fractures involving subjacent ribs, supporting an AP compressive mechanism. Strouse and Owings have suggested that an additional mechanism is at work when more laterally situated first rib fractures are encountered (21). They believe that an upward force exerted by the scalene muscles on the first rib during shaking or axial loading explains the fractures. The occurrence of laterally situated first rib fractures, without subjacent rib fractures supports this view (see below). The factors resulting in first rib fractures are complex, but what is clear is that these uncommon injuries require high energy forces occurring with severe assaults. Curiously, a fracture of the first rib has been described in an 11-year-old child with pertussis (67). Care should be taken to avoid confusion of lateral first rib fractures with anatomic variants in ossification (see Chapter 12) (68).
Conclusions
Although there are a variety of mechanisms resulting in rib fractures in abused children, the morphologic features of these injuries allow some general conclusions to be drawn. If the rib cage is viewed as a functional unit composed of a series of parallel struts attached anteriorly to the sternum and posteriorly to the spine, complex patterns of rib injury can be explained. Because diffuse forces are delivered to similar locations of multiple adjacent rib arcs, a row of fractures may occur at similar sites in several adjacent ribs. When there is compression of a rib arc with excessive posterior leverage over the transverse process and/or severe stress applied along the inner margin of the lateral and anterior arcs of the ribs, multiple fractures within individual ribs may result. If symmetric forces are applied to both hemithoraces as the infant is held and squeezed by the chest or slammed, bilateral mirror-image fractures of similar ages may be noted. Ribs may undergo repeated injury; exuberant callus can be present around a site of repeated injury and a more recent fracture may be visible elsewhere in the same rib. An appreciation of basic anatomy and the biomechanical principles outlined above provides a systematic basis to approach the radiologic patterns of rib injury.
Imaging features
Radiography
Acute posterior rib fractures are often invisible radiographically, even with high-detail imaging systems (Fig. 5.18) (15, 16, 47, 51, 69). In the absence of callus formation, detectability of the fracture depends on the plane of the fracture line and the position and alignment of the fracture fragments (43). When no displacement is present and the fracture courses at an obliquity to the plane of the x-ray beam, the fracture will be invisible on the frontal radiograph (Fig. 5.18A). Axial radiography and histologic study reveal the fracture (Fig. 5.18B,C). When multiple acute injuries are present, some of which are displaced, the initial study may provide a hint of injury at some sites. However, a follow-up examination in several weeks will show striking callus formation due to multiple fractures (Fig. 5.19) (70).
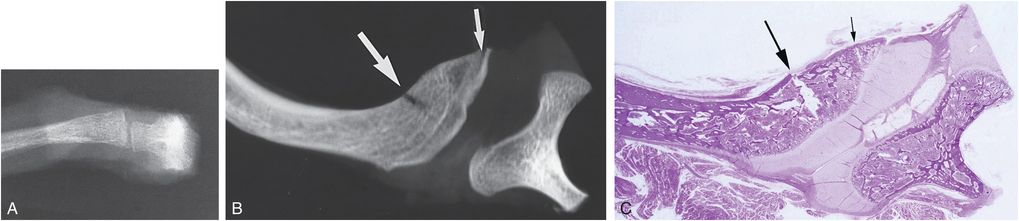
Figure 5.18 Posterior rib fracture: influence of the fracture plane on visibility. A, Frontal specimen radiograph of posterior rib and vertebral articulation in a two-month-old abused infant. A fracture was noted at gross autopsy. No fracture is visible radiographically. B, An axial specimen radiograph demonstrates an acute fracture at the level of the costotransverse process articulation (long arrow) and a fracture of the rib head (short arrow). C, An axial histologic section demonstrates fractures of the rib head (short arrow) and neck (long arrow). (From Kleinman PK, Marks SC Jr, Adams VI, Blackbourne BD. Factors affecting visualization of posterior rib fractures in abused infants. AJR. 1988;150:635–8.)
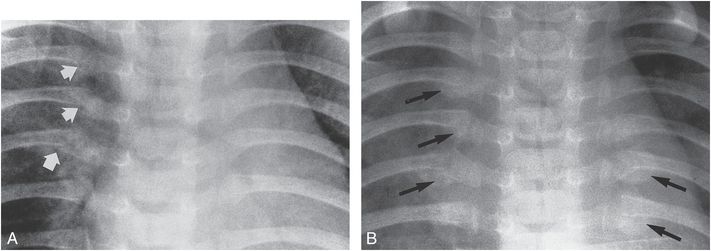
Figure 5.19 Acute posterior rib fractures in a six-week-old abused infant: value of follow-up study. A, Initial study demonstrates possible fractures of the right fourth, fifth, and sixth rib necks (arrows). B, Follow-up study approximately 10 days later confirms the fractures on the right and reveals callus formation around the fractures of the left sixth and seventh rib necks. Note that callus appears predominantly below the rib margins (arrows).
The subtlety of acute posterior rib fractures provides an argument for the use of skeletal scintigraphy and CT in the initial evaluation of suspected abuse (16, 47, 55, 69, 71–73). When healing is present, posterior rib fractures usually become visible. The notable exceptions involve the rib head. Because rib head fractures are commonly modest injuries that disrupt the ventral aspect of the rib head adjacent to the COJ and frequently have no associated SPNBF, they may be undetectable on frontal radiography. When visible, they appear as subtle vertically oriented radiolucencies along the extreme medial aspect of the rib. Occasionally, faint sclerosis is noted along the fracture margins radiographically (Fig. 5.20) or on CT (see Fig. 5.7).
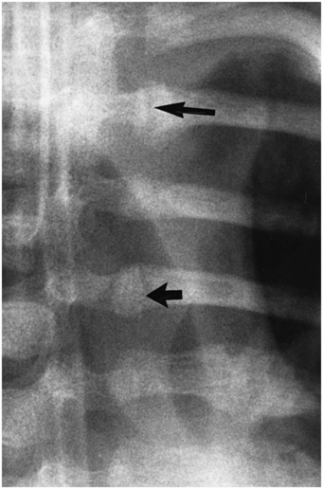
Figure 5.20 Rib head fractures in a two-month-old abused infant. Radiograph of the upper left ribs reveals subtle sclerosis along the fracture of the second rib head (long arrow). There is no evidence of healing along the fourth rib head fracture (short arrow).
With more extensive injury to the rib head, new bone formation and sclerosis become visible with healing; the callus formation and SPNBF may extend well lateral to the transverse process, providing radiographic visualization (Fig. 5.21). A subtle feature of healing posterior fractures is slight apparent widening of the rib neck. This may occur with early healing due to fine SPNBF. In these instances the margins of the rib are indistinct (Fig. 5.22).
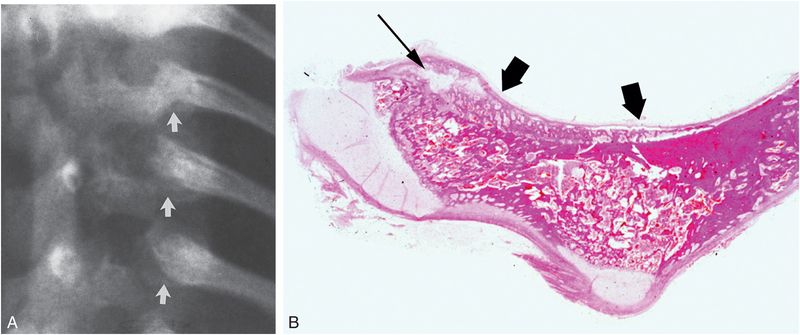
Figure 5.21 Healing rib head fractures in a three-month-old abused infant. (See same patient in Fig. 3.71.) A, View of the left posterior ribs demonstrates irregularity, sclerosis, and callus formation involving the heads of the fifth-to-seventh ribs. Visibility is enhanced by extension of SPNBF along the rib necks (arrows). B, Axial histologic section of the fifth rib shows the rib head fracture (thin arrow) and extension of SPNBF to the rib neck (thick arrows). (From Kleinman PK, Marks SC Jr, Adams VI, Blackbourne BD. Factors affecting visualization of posterior rib fractures in abused infants, AJR. 1988;150:635–8.)
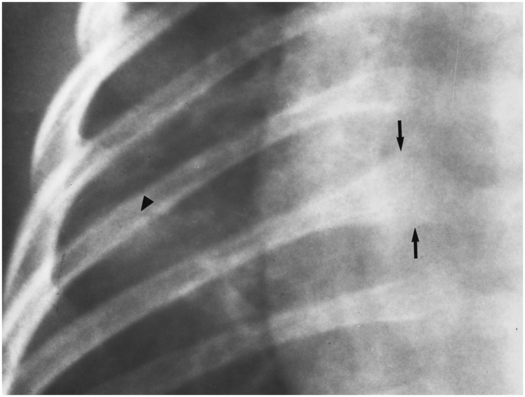
Figure 5.22 Healing rib neck fracture in an abused infant. An oblique radiograph shows apparent widening of the rib neck (arrows). The margins are indistinct, suggesting that this is a relatively recent injury. A faint radiolucent line is noted in the posterolateral aspect of the superjacent rib. As there is faint callus formation at this site, a healing fracture is confirmed (arrowhead).
With advanced healing and remodeling, a widened rib neck may also be evident, and in these cases the rib margins will be sharply defined with distinct underlying trabecular architecture (Fig. 5.23). The rib head may have a fragmented appearance with mixed sclerosis and lucency. This probably results from repeated injury (Fig. 5.24). Fractures of the rib neck typically become evident on high-detail radiographs approximately 7–10 days after injury, although early documentation of healing depends on the quality of the radiograph. The amount of callus and SPNBF varies with the amount of osseous disruption, periosteal elevation, hemorrhage, motion, and repetitive injury. Modest injuries manifest fine subperiosteal new bone and may be easily overlooked (Fig. 5.25; also see Fig. 5.8). However, the osseous injury may be associated with substantial hemorrhage, and an extrapleural soft tissue density may be visible on the lateral radiograph (Fig. 5.25B). This may provide the first clue to underlying rib injury, which can be confirmed by high-detail skeletal imaging (Fig. 5.25C).
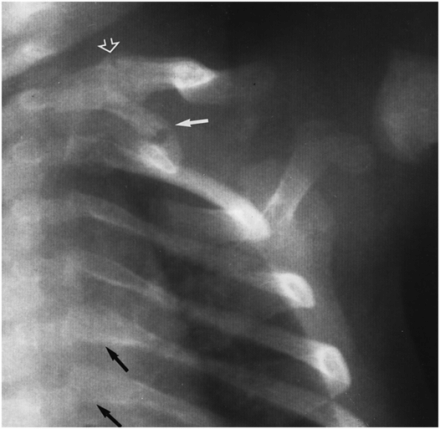
Figure 5.23 Rib neck, first rib, and clavicular fractures in an abused infant. Widening of the necks of the left fifth and sixth ribs is seen. The margins are quite sharply defined, suggesting that these are mature (black arrows). There is a recent fracture of the lateral aspect of the left first rib (white arrow) and a healing fracture of the proximal clavicle (open arrow).
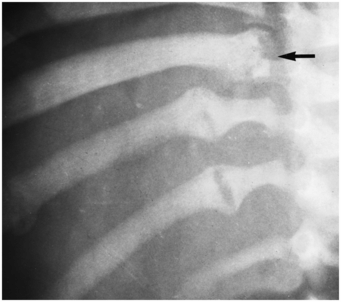
Figure 5.24 Healing rib head fracture in an abused infant. A fracture of the right ninth rib head is manifested by irregularity, sclerosis, and fragmentation (arrow). This probably reflects repetitive injury. Fractures of the tenth and eleventh rib necks are noted, with evidence of advanced healing.
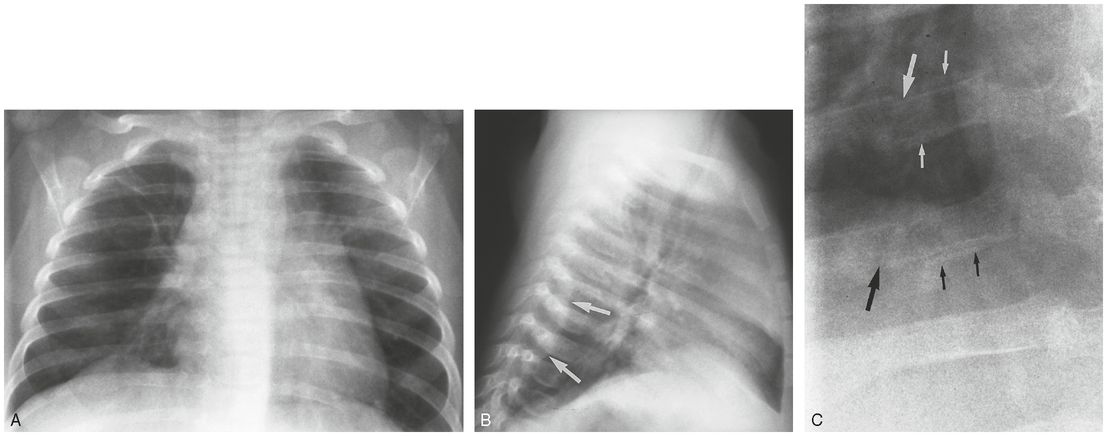
Figure 5.25 Extrapleural soft tissue density with rib neck fracture in a four-month-old abused infant. PA (A) and lateral (B) chest films obtained for suspected respiratory disease reveal a soft tissue mass on the lateral projection (arrows). No definite abnormality is seen on the frontal view. C, A high-detail view of the right lower ribs demonstrates fractures of the necks of the right ninth and tenth ribs (large arrows) with fine SPNBF (small arrows).
Most posterior rib fractures manifest a substantial area of callus formation, often projecting mainly below the inferior margin of the rib. In the phase of soft callus (see Chapter 6) the margins are indistinct without a clear trabecular architecture (Fig. 5.19B). With further healing a sharply marginated zone of callus, often with a nodular appearance, becomes evident (see Figs. 5.7A, 5.9, 5.12). In some cases the fracture line is difficult to identify, but in other instances a distinct vertical lucency conforming to the fracture line is visible (Fig. 5.24; see also 5.13).
Lateral and anterior arc fractures pose similar problems in detection. They are difficult to identify acutely unless displacement or separation of the fracture fragments is present (see Figs. 5.12, 5.14). Oblique views are useful, as they may bring the fracture line into tangent or accentuate any modest displacement (Figs. 5.26, 5.27A,B) (44, 45). Because rib fractures tend to be multiple, and the total number of fractures is often underestimated on early radiographs, as noted, extrapleural density related to hemorrhage or early callus formation may be a clue to additional fractures invisible radiographically (Fig. 5.28). With more advanced healing, callus, and SPNBF enhance the detectability of lateral arc fractures (Figs. 5.27C,D, 5.29). Early callus may be difficult to differentiate from a parenchymal density on a chest radiograph, but a follow-up study should resolve this issue (Fig. 5.29).
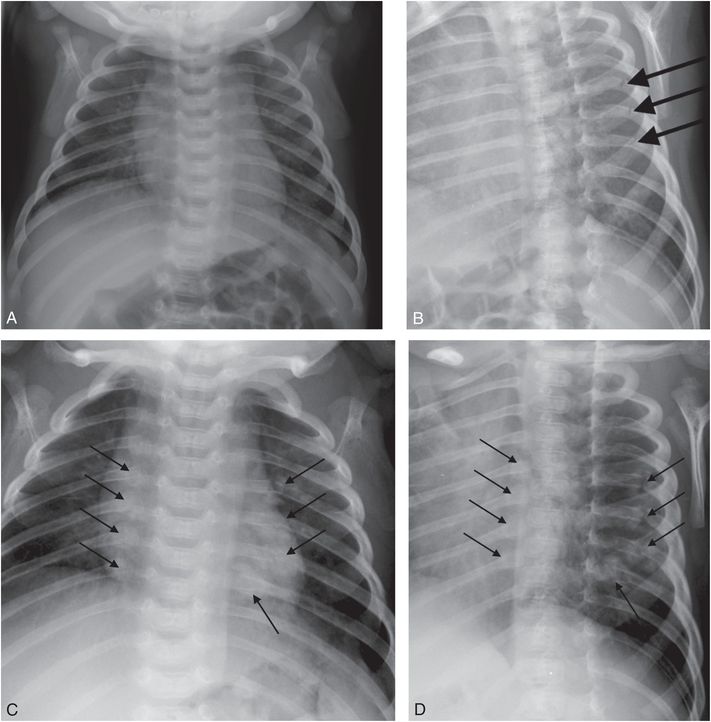
Figure 5.26 Rib fractures not visible on PA radiograph and others seen only on follow-up SS. A five-week-old infant with multiple bruises, abrasions, and bite marks. A, AP view of the thorax from initial SS shows no fractures. B, Right posterior oblique (RPO) view demonstrates acute fractures of the posteromedial aspects of the left fifth-to-seventh ribs (arrows). There were multiple CMLs. Mother and father were reportedly both smoking crack cocaine and the father described the mother beating the infant. AP (C) and RPO (D) views of the two-week follow-up survey demonstrate healing about the previously noted fractures, as well as healing fractures at the costovertebral articulations of the left eighth and the right fifth-to-eighth ribs (arrows).
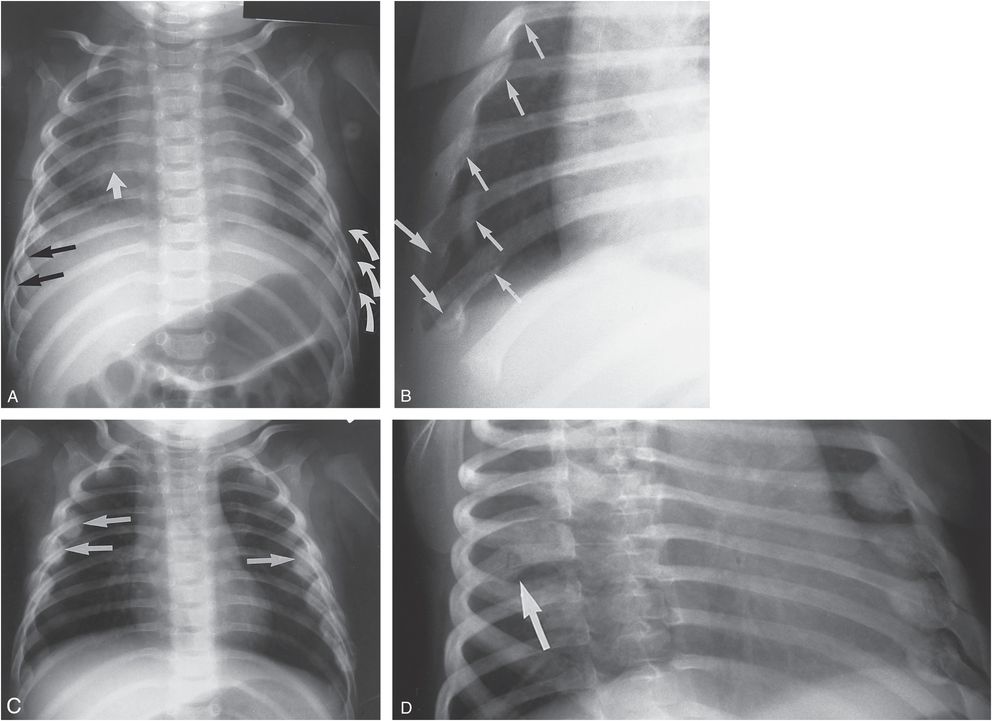
Figure 5.27 Multiple rib fractures with follow-up study in a two-month-old abused infant. A, A frontal radiograph demonstrates recent fractures of the left fifth-to-seventh ribs with displacement (curved arrows). SPNBF is noted along the anteromedial aspects of the right sixth and seventh ribs (black arrows). There is a suggestion of a fracture of the posterior aspect of the right sixth rib (straight white arrow). B, An oblique view of the right ribs shows that there are also fractures of the anterolateral aspects of the right fifth-to-eighth and possibly ninth ribs (small arrows). Additionally, there are CCJ fractures of the eighth and ninth ribs (large arrows). AP (C) and left posterior oblique (LPO) (D) views two weeks later show extensive callus formation about the recent rib fractures noted on the initial study, as well as healing of the left fourth and right third and fourth lateral arcs (arrows in C) not visible on the earlier frontal examination. A healing fracture of the posterior arc of the right sixth rib is now visible, particularly well seen on the LPO projection (arrow in D).
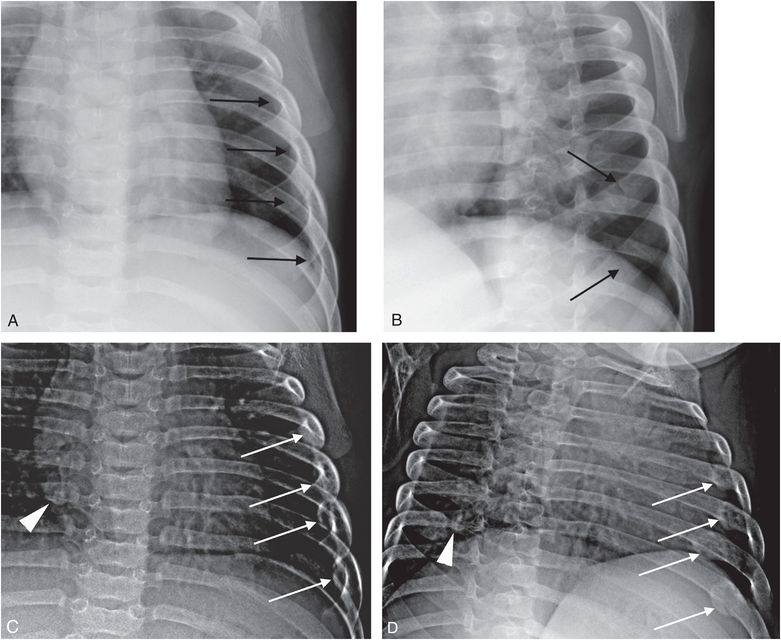
Figure 5.28 The value of oblique radiographs and extrapleural density in identifying fractures. A five-month-old infant whose mother noted raised erythematous/edematous lesions covering the lower and upper extremities. The father later indicated that he had recently placed the child in a freezer in an effort to reduce a high fever. A, AP view of the thorax from the SS shows subtle extrapleural density along the inner concavity of the left fourth-to-seventh ribs (arrows). B, The RPO projection shows subtle acute fractures of the lateral aspects of the left sixth and seventh ribs (arrows). AP (C) and LPO (D) views from the follow-up study 12 days later show healing about the fractures of the sixth and seventh ribs and also healing injuries of the left fourth and fifth ribs (arrows). The extrapleural density provides an early clue to the presence of adjacent fracture. Additionally, there is a healing fracture of the neck of the right seventh rib (arrowhead).
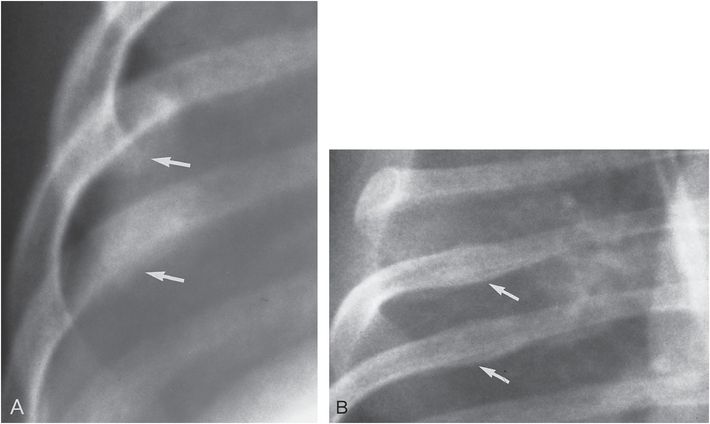
Figure 5.29 Lateral arc fracture with early healing in a two-month-old abused infant. A, Faint callus formation is seen along the inferior margin of the ribs (arrows) without a distinct fracture line. It is difficult to differentiate this density from underlying parenchymal disease. B, A follow-up study several weeks later shows mature SPNBF at the fracture sites, but again no fracture line is visible (arrows).
An interesting phenomenon is the tendency for healing fractures to show an area of rounded radiolucency at the fracture site, the so-called “hole-in-the-rib” pattern (Fig. 5.30) (56, 74). This appearance relates to the presence of bone resorption with surrounding sclerosis at the fracture site (Fig. 5.31) (49). Injuries near the CCJ are exceedingly difficult to identify acutely. It is therefore likely that these rib fractures are more common than suggested by the early literature (14, 15, 32, 38, 44, 45, 47, 65, 75). Even with oblique views, and with healing, there may be little bone production to point to injury (see Fig. 5.17). However, on occasion substantial fracture callus may produce a rounded opacity at the CCJ, first noticed on an abdominal radiograph and confirmed on views of the thorax (Fig. 5.32). A slightly different pattern is an arcuate bony density at the rib end, which can produce a “ring shadow” on appropriate projections and a faint layer of SPNBF may be visible (Figs. 5.27B, 5.32D). Ng and Hall have drawn the analogy between this appearance and the bucket handle pattern of the CML in the extremities (Fig. 5.33) (65).
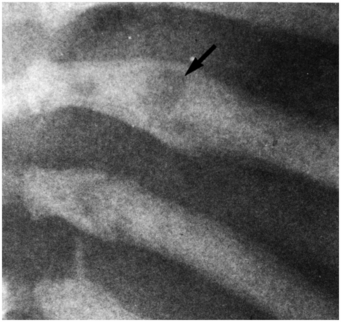
Figure 5.30 “Hole-in-the-rib” appearance. Posterior arc fracture in an abused infant manifested by mature subperiosteal new bone and a central radiolucency, suggesting a lytic lesion (arrow).
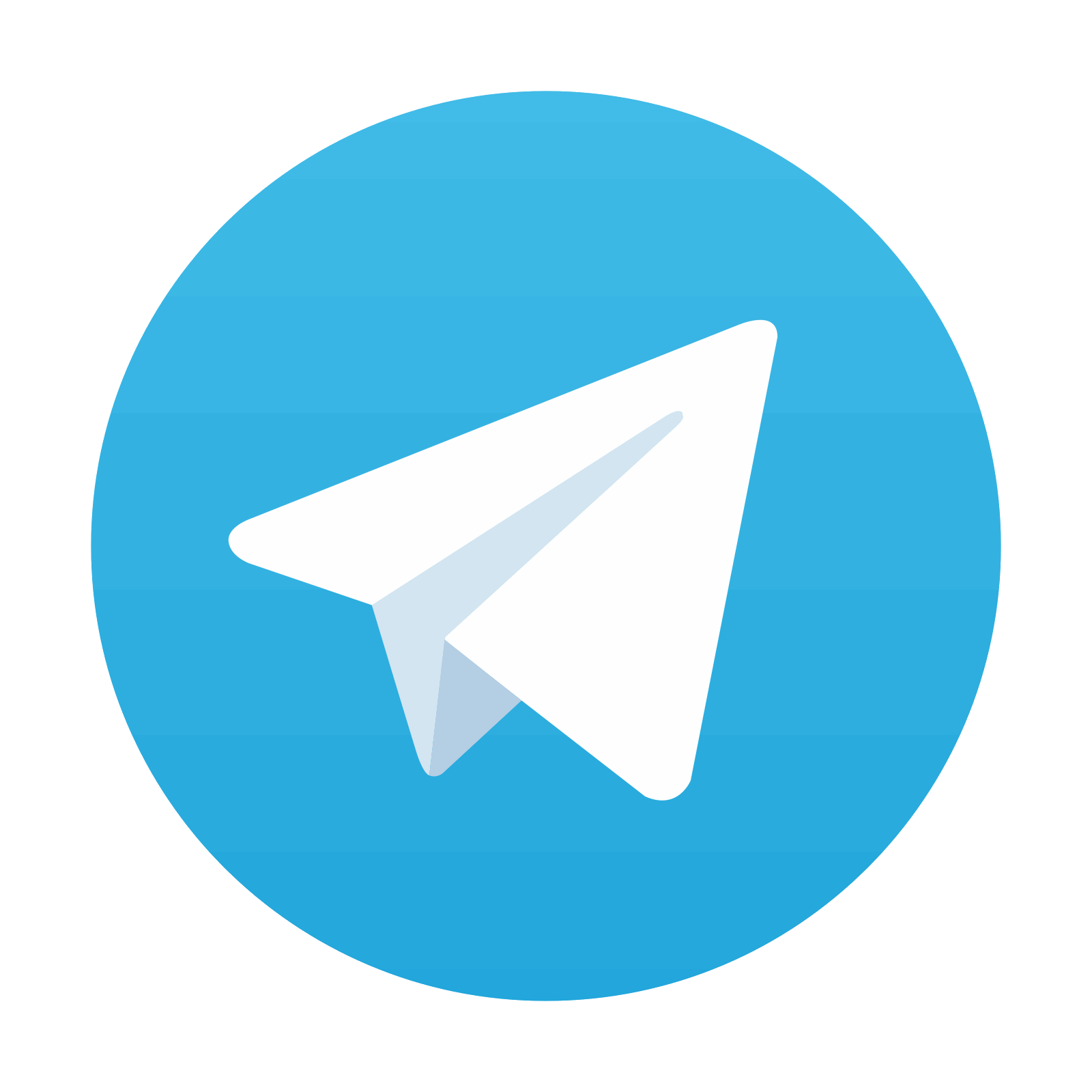
Stay updated, free articles. Join our Telegram channel

Full access? Get Clinical Tree
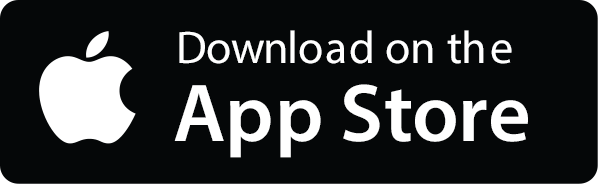
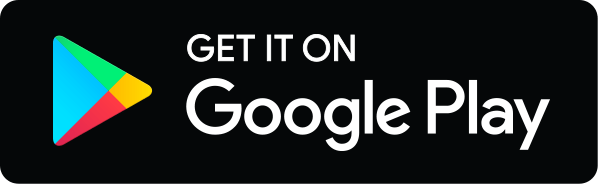