Fig. 13.1
Tumours appear cold in the diagnostic scan using 198Au-chloride (1 μm diameter) injected intravenously and trapped in the reticuloendothelial cells of the liver (Reprinted from Simon and Feitelberg [2] with permission of the Radiological Society of North America)
The first use of an Anger camera for bremsstrahlung imaging was reported by Kaplan et al. in 1985 [3] for 32P. Since the 1990s, bremsstrahlung imaging is widely performed in clinical routine for post-therapy check using beta emitter, such as 90Y synovectomy or 90Y liver radioembolisation. However, medical publications about bremsstrahlung imaging are sparse (Table 13.1).
Table 13.1
Sorted article counts obtained from www.pubmed.com when searching the combination (bremsstrahlung and (SPECT or SPET or PET or planar or imaging or whole body)) in title/abstract
Year | 89Sr | 32P | 90Y | 90Y | ||
---|---|---|---|---|---|---|
Planar | SPECT | Planar | SPECT | PET | ||
<2007 | 3 | 3 | 3 | 7 | ||
2007 | 2 | 1 | ||||
2008 | 2 | |||||
2009 | 1 | 3 | 1 | |||
2010 | 1 | 1 | 1 | 2 | 3 | |
2011 | 1 | 2 | 4 | 6 | ||
2012 | 3 | 2 | 3 | 4 | 7 |
More recently, Lhommel, Walrand et al. showed that the low positron emission of 90Y can be usefully imaged by PET after liver radioembolisation [4, 5] and after 90Y-DOTATOC therapy as well [6]. Although giving final images, a little bit noisier than bremsstrahlung SPECT, pure commercial TOF-PET systems directly provide good spatial resolution and quantification accuracy in 90Y imaging. On the contrary, to achieve similar quality, bremsstrahlung SPECT requires sophisticated reconstruction or acquisition software not commercially available [7–9]. Those features explained the increasing use of PET to image 90Y in place of bremsstrahlung SPECT (Table 13.1).
However, PET modality is not always easily accessible and as 32P and 89Sr do not own any usable isotope emitting γ rays which could be imaged, bremsstrahlung SPECT remains of paramount importance.
13.2 Bremsstrahlung SPECT Issues
Accurately imaging 90Y, 32P or 89Sr with a γ camera is one of the most challenging topics in nuclear medicine. The bremsstrahlung x-rays are spread along a continuous spectrum extending to energies up to the maximal beta energy emission, i.e. 2.3, 1.7 and 1.5 MeV for 90Y, 32P and 89Sr, respectively. The maximal energy usable by γ camera owning a mechanical collimator, such as Anger or CZT cameras, is limited to about 0.5 MeV. As a result, all acquisitions using such cameras are inevitably corrupted by high energy x-rays scattering down into the acquisition energy window.
This high energy x-rays down scattering contamination includes four different effects (Fig. 13.2): (1) the scattering inside the patient body, (2) the scattering through a collimator septa (usually called penetration), (3) the scattering from a collimator septa, (4) the lead fluorescence K α and K β emissions and (5) the back-scattering from the PMT, electronic boards and lead housing of the camera. Although less frequent, some x-ray paths can include several of the sub-cited effects. Lastly, the β range in the patient also slightly alters the final spatial resolution.
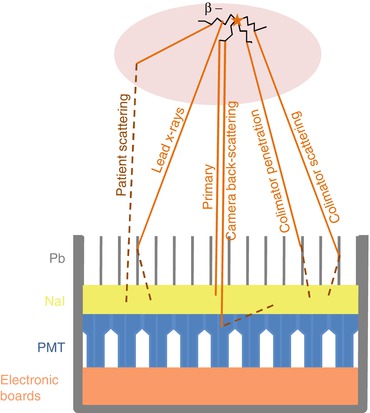
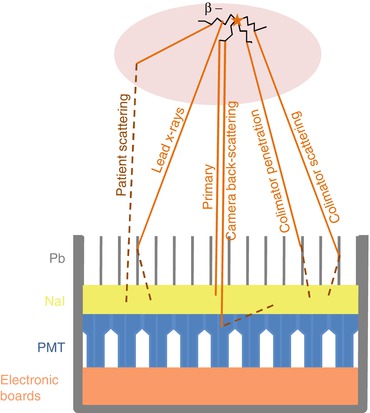
Fig. 13.2
The different x-ray paths producing a photoelectric effect in the camera crystal
Monte Carlo simulations allow assessing the individual contributions to the total x-rays producing a photoelectric effect in the camera crystal [10] (Fig. 13.3). Note that on the contrary of conventional γ emitters, the primary photons represent only a small part of the total detected counts. The most favourable ratio is obtained around 100 keV explaining why the 50 → 150 keV energy window is often chosen [10, 11]. A medium energy general purpose (MEGP) parallel hole collimator is a good choice to reduce the collimator penetration. However, even with this energy window and collimator choice, spatial resolution and quantification accuracy of bremsstrahlung SPECT remain low. Different approaches are proposed to improve this situation: physical effects modelling and adapted collimator design.
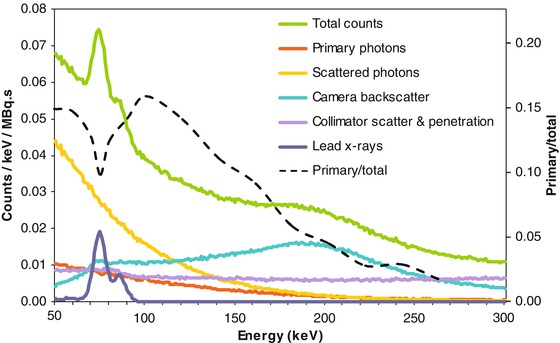
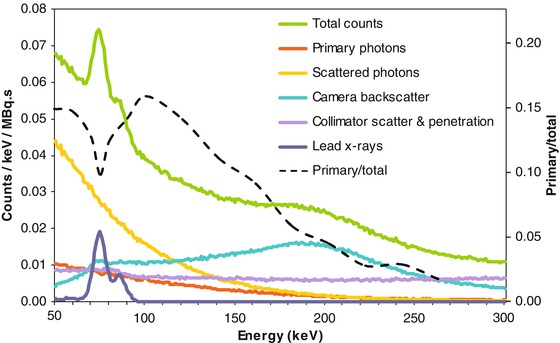
Fig. 13.3
90Y x-ray energy spectra according to photon origin for a point source 5 cm deep in water cube, MEGP collimation, 3/8 in. thick NaI, computed by Monte Carlo simulation (Courtesy of Dr. S Heard). The primary to total counts curve (dashed black curve) was added by the author of the present chapter
13.3 Intra-patient Scatter and Collimator-Detector Response Modelling
With the increasing speed of the computer stations, most tomographic reconstructions in nuclear medicine are nowadays performed using the iterative algorithm OSEM, an accelerated version of the EM-ML. Compared to analytical reconstruction, such as FBP, these iterative algorithms have the benefit to correctly account for the Poisson nature of the statistical noise present in radioactive counts measurement and to avoid apparition of negative voxel artefacts. In addition, iterative algorithms can reconstruct any tomographic acquisition setups, i.e. all physical effects introduced in the projection step of the iterative loop will be corrected during the reconstruction process. The state of the art in bremsstrahlung SPECT is thus to model the different x-ray paths during this projection step. However, for the time being an exact modelling of these effects leads to reconstruction time incompatible with the daily SPECT routine.
Minarik et al. [12] built a pre-calculated collimator-detector response (CDR) table by Monte Carlo simulation and modelled the scatter into the object using the effective source scatter estimation (ESSE) method [13]. The modelling was performed for a 105–195 keV acquisition window in order to avoid the lead fluorescence x-rays contamination. This model was incorporated into the OSEM reconstruction algorithm. Evaluation in an abdominal phantom showed a quantification accuracy of 8.5 % for the liver activity. Accuracy in lesions activity measurement was not assessed.
This group applied their correction method in 3 patients receiving high-dose 90Y radioimmunotherapy [14]. The patients were imaged at 1, 24, 48, 72, 144 and 166 h by SPECT/CT after a pre-therapeutic injection of 300 MBq of 111In-ibritumomab. Patients received a 90Y-ibritumomab activity computed to deliver 12Gy to the liver based on the pharmacokinetics measured with the 111In SPECT/CT and were imaged at the same time points postinjection by bremsstrahlung SPECT/CT. The absolute relative differences between organ absorbed dose computed from 111In and 90Y SPECT/CT were 8.8 ± 13.7, 8.9 ± 4.0 and 51.7 ± 18.9 (mean ± std in %), for the liver, kidneys and lungs, respectively. This showed that this bremsstrahlung SPECT/CT correction method can be used for the body region below the lungs: for the time being, the ESSE method does not account for tissue density variation, such as present in the slices crossing the lungs.
In order to improve the count rate for applications where the activity is modest, such as 90Y radioimmunotherapy, Rong et al. [15] computed by Monte Carlo the CDR and ESSE for the wide 100–500 keV acquisition window. The computation was performed using separate treatment of photons in various energy ranges and in various logical categories. Evaluation in an elliptical phantom containing three spheres of 5.5, 3.3 and 1.5 cm diameter, with specific activity 10-, 10- and 20-fold that of the surrounding background, showed a quantification accuracy of 7, 9.7 and 10.2 %, respectively.
However, in medical imaging it is always profitable to improve the hardware performance in order to acquire the right events, rather than to correct for the contaminating events afterwards. Indeed, this last solution inevitably results in a higher noise level regarding the statistical nature of primary and contaminating photon. Amazingly, other choices of collimators than MEGP have been considered only very recently.