Simultaneous acquisition PET/MR imaging combines the anatomic capabilities of cardiac MR imaging with quantitative capabilities of both PET and MR imaging. Cardiac PET/MR imaging has the potential not only to assess cardiac tumors but also to provide thorough assessment of myocardial ischemia, infarction, and function and specific characterization of cardiomyopathies, such as cardiac sarcoid. In this article, the authors start with a discussion of the technical challenges specific to cardiovascular PET/MR imaging followed by a discussion of the use of PET/MR imaging in various cardiovascular conditions.
Key points
- •
Early efforts of cardiovascular PET/MR imaging research emphasized accurate magnetic resonance (MR)-based attenuation correction of PET data.
- •
PET/MR imaging in cardiology shows promise in infarct characterization, ischemia, and atherosclerosis evaluation.
- •
Utility of PET/MR in other cardiovascular conditions, such as cardiac sarcoidosis and myocarditis, also shows promise.
Introduction
Simultaneous acquisition PET/MR imaging combines the anatomic capabilities of cardiac MR imaging with quantitative capabilities of both PET and MR imaging. Cardiac PET/MR imaging has the potential to provide thorough assessment of myocardial ischemia, infarction ( Fig. 1 ), and function as well as characterization of cardiomyopathies such as cardiac sarcoid and myocarditis. In this article, the authors discuss the technical challenges specific to cardiovascular PET/MR imaging followed by a discussion of the use PET/magnetic resonance (MR) in various cardiovascular conditions.
Although much of the early PET/MR imaging research centered on oncology and neurology applications, the number of research publications on PET/MR imaging cardiovascular applications has increased over the last few years. Simultaneous acquisition cardiac PET/MR imaging combines the reference standard for myocardial perfusion (PET) with the reference standard for functional assessment (gated cine MR imaging). In addition, cardiac PET/MR has the ability to assess viability both by 18 F-fluorodeoxyglucose ( 18 F-FDG) PET and by MR imaging, specifically assessing regions of myocardial infarction and fibrosis by late gadolinium enhancement (LGE) or T1 mapping. Myocardial edema can be evaluated by T2 MR imaging, and molecular information on inflammation and macrophage presence can be targeted by novel PET tracers.
Limitations to routine clinical use of cardiac PET/MR imaging have included validation of MR imaging for PET attenuation correction and methods to motion correct both PET and MR imaging. Although multiple articles have been now been published validating MR imaging for attenuation correction in cardiac imaging, and methods exist to fuse diastolic-acquired list-mode data to diastolic MR imaging data, PET optimal respiratory and cardiac motion correction methods are still in the process of being explored.
Box 1 lists some of the advantages and limitations of PET/MR as it pertains to cardiovascular imaging.
Potential advantages of PET/MR over PET/CT in cardiovascular imaging:
- •
Reduced radiation dose in comparison to PET/CT
- •
Combined metabolic, functional, and high-contrast anatomic imaging
- •
Viability assessment by both metabolic and anatomic methods
- •
Combined PET and MR imaging examination is shorter than separately performed examinations
- •
Potential for myocardial blood flow comparison by both MR imaging and PET stress perfusion
Potential limitations of PET/MR in cardiovascular imaging:
- •
Smaller inner bore of the PET/MR imaging scanner may exclude oversized patients
- •
No breath-hold during PET acquisition means that alternate MR imaging methods of respiratory motion suppression are necessary
- •
Technical challenges with dual respiratory and cardiac motion correction
- •
Contraindications to MR imaging (such as implantable defibrillators or MR–non-compatible pacemakers)
- •
Non-MR imaging–compatible generators (Rubidium-92) are a challenge
Introduction
Simultaneous acquisition PET/MR imaging combines the anatomic capabilities of cardiac MR imaging with quantitative capabilities of both PET and MR imaging. Cardiac PET/MR imaging has the potential to provide thorough assessment of myocardial ischemia, infarction ( Fig. 1 ), and function as well as characterization of cardiomyopathies such as cardiac sarcoid and myocarditis. In this article, the authors discuss the technical challenges specific to cardiovascular PET/MR imaging followed by a discussion of the use PET/magnetic resonance (MR) in various cardiovascular conditions.
Although much of the early PET/MR imaging research centered on oncology and neurology applications, the number of research publications on PET/MR imaging cardiovascular applications has increased over the last few years. Simultaneous acquisition cardiac PET/MR imaging combines the reference standard for myocardial perfusion (PET) with the reference standard for functional assessment (gated cine MR imaging). In addition, cardiac PET/MR has the ability to assess viability both by 18 F-fluorodeoxyglucose ( 18 F-FDG) PET and by MR imaging, specifically assessing regions of myocardial infarction and fibrosis by late gadolinium enhancement (LGE) or T1 mapping. Myocardial edema can be evaluated by T2 MR imaging, and molecular information on inflammation and macrophage presence can be targeted by novel PET tracers.
Limitations to routine clinical use of cardiac PET/MR imaging have included validation of MR imaging for PET attenuation correction and methods to motion correct both PET and MR imaging. Although multiple articles have been now been published validating MR imaging for attenuation correction in cardiac imaging, and methods exist to fuse diastolic-acquired list-mode data to diastolic MR imaging data, PET optimal respiratory and cardiac motion correction methods are still in the process of being explored.
Box 1 lists some of the advantages and limitations of PET/MR as it pertains to cardiovascular imaging.
Potential advantages of PET/MR over PET/CT in cardiovascular imaging:
- •
Reduced radiation dose in comparison to PET/CT
- •
Combined metabolic, functional, and high-contrast anatomic imaging
- •
Viability assessment by both metabolic and anatomic methods
- •
Combined PET and MR imaging examination is shorter than separately performed examinations
- •
Potential for myocardial blood flow comparison by both MR imaging and PET stress perfusion
Potential limitations of PET/MR in cardiovascular imaging:
- •
Smaller inner bore of the PET/MR imaging scanner may exclude oversized patients
- •
No breath-hold during PET acquisition means that alternate MR imaging methods of respiratory motion suppression are necessary
- •
Technical challenges with dual respiratory and cardiac motion correction
- •
Contraindications to MR imaging (such as implantable defibrillators or MR–non-compatible pacemakers)
- •
Non-MR imaging–compatible generators (Rubidium-92) are a challenge
Technical challenges of PET/MR cardiovascular imaging
The technical challenges of cardiac PET/MR imaging are unique.
- 1.
In terms of attenuation correction : there are few bony structures in the immediate vicinity of the heart, making accurate MR-attenuation correction easier to achieve in comparison neurologic imaging.
- 2.
In terms of motion correction : 2 types of motions, including respiratory and cardiac motions, need to be tracked and motion-corrected for optimal image registration.
- 3.
Specific to cardiac PET imaging, optimizing myocardial radiotracer uptake is necessary especially if 18 F-FDG will be used, and patient preparation protocols are needed to either enhance or suppress myocardial glucose uptake, depending on the clinical question.
Attenuation Correction
Imaging a variety of organ systems requires different sets of MR sequences and PET radiotracers to generate the maximal complementary information between anatomy, function, and specific molecular signals. Accurate quantitation of radiopharmaceutical uptake is highly dependent on accurate attenuation correction of the PET signals, in the absence of a conventional computed tomographic (CT)-based μ-map. Accurate attenuation correction is crucial because quantitative measurements of standardized uptake values (SUVs) are routinely required in clinical practice to make meaningful interpretations of the PET data. In addition, in cardiac PET myocardial perfusion, absolute myocardial blood flow can be quantified. The ability to quantify blood flow provides PET with a clear advantage over traditional single photon emission computed tomography (SPECT), which does not allow for dynamic acquisition and, thus, can provide only relative perfusion information.
An early emphasis in PET/MR cardiac research has been on addressing the validity of Dixon MR-based attenuation correction. One particularly important challenge in attenuation correction in PET/MR is that PET intensity adjacent to bony structures can be underestimated, leading to underdetection of weakly positive PET signal sources. Fortunately, there are few major bony structures in the proximity of the heart, thus diminishing the concern in cardiac PET/MR imaging. A second issue is the validation of the MR-attenuation correction methods available in PET/MR for ability to correctly provide an attenuation map of the beating heart with accurate coregistration to the average position of the heart during free-breathing. Early validation studies showed feasibility of the 4-compartment method MR-based attenuation correction method in quantifying SUVs using PET/MR. In a 4-compartment method, air, soft tissue, fat, and lung are separated and assigned different attenuation coefficients, using a model based on a T1-weighted Dixon sequence. Because this process is based on proton spin precession under changing magnetic fields and not on electron density as is the CT-based method for attenuation correction, which directly relates to photon attenuation, PET SUV derived by PET/MR required validation against PET SUV derived by PET/CT.
Nensa and colleagues first reported that in 10 patients who underwent sequential cardiac PET/CT and PET/MR, there was no significant difference in myocardial tracer uptake between PET/CT and PET/MR images (paired t -test, P = .95). Similar findings were reported by Lau and colleagues. In 30 patients who underwent sequential 18 F-FDG PET/CT and PET/MR imaging, a strong correlation was noted between CT-based attenuation correction SUV and MR-based attenuation correction SUV (Pearson correlation, R 2 = 0.97).
Although the MR phased array coil, through its attenuation effect, may cause an approximately 3% reduction of myocardial SUV, in both studies above, the SUVs derived by MR-attenuation correction were slightly higher than SUVs derived by CT-attenuation correction. This finding is likely a systematic bias, because in both studies the PET/CT scan was performed before the PET/MR scan; thus, there was more time for 18 F-FDG to be taken up into the myocardium in the PET/MR data set. Overall, however, for general clinical examination, the 2-point Dixon MR examination has been determined to be suitable for myocardial attenuation correction. An area where PET/MR imaging could provide advantages above PET/CT in attenuation correction is in the development of MR-based motion attenuation correction methods to ensure an accurate respiratory phase matching between PET and MR-attenuation correction data sets, as is discussed later.
Motion Correction
Simultaneous PET/MR imaging of the heart offers the opportunity to account for both respiratory and cardiac motion. Failure to appropriately account for this motion can lead to errors in segmentation and produce lower signal-to-noise ratios, thus reducing the quantitative and the qualitative accuracy of a cardiac PET/MR examination. More importantly, motion correction that includes both respiratory and cardiac motion has the potential to improve registration accuracy of PET/MR imaging datasets and to improve PET quantitation accuracy. A recent review article by Fayad and colleagues summarizes the current state of motion correction in cardiac PET/MR imaging.
Usually, cardiac PET/MR motion correction includes acquiring respiratory- and/or cardiac-gated PET data sets along with their associated synchronized anatomic MR data set. Cardiac motion synchronization (gating) is typically achieved by electrocardiogram gating. On the other hand, respiratory motion synchronization can either be achieved by use of various sensors, either internal to the sequence, such as an MR navigator echo, or externally such as spirometry, respiratory belt, or optical monitoring system. Alternatively, respiratory data can be extracted from the acquired PET or MR data directly. Both the external sensor and the data-driven approach seem to yield comparable results in noncardiac PET/MR examinations. To date, most facilities use the external device approach for respiratory motion-gating PET data.
The simple approach of respiratory gating for motion correction of PET data in PET/MR imaging is not without limitations: mainly, the gated images have a lower statistical count, and respiratory motion is not always predictably one-dimensional. As a result, various more elaborate motion estimation models have been proposed, which require multiple steps that include acquiring a motion surrogate, estimating or modeling with subsequent correction. These multistep correction algorithms may be image-based, reconstruction-based, or raw data–based estimation models, but these algorithms are still in an experimental phase and have not been fully adopted for routine clinical use. Much work remains to validate these more sophisticated algorithms for their validity and superiority in cardiac PET/MR motion correction.
Optimizing Myocardial PET Signal Uptake
18 F-FDG is a glucose analogue widely used in oncology to assess increased glucose metabolism in malignant lesions. It is also used in cardiac PET imaging to diagnose myocardial infarction and viability and in cardiac inflammatory and granulomatous diseases, such as cardiac sarcoidosis and infective endocarditis. For assessment of inflammatory conditions, suppression of 18 F-FDG uptake in the normal myocardium is necessary so that areas of inflammation can be detected. In these situations, a high-fat, no-carbohydrate patient preparation can be used as per the following protocol :
- •
At least 2 high-fat, no-carbohydrate meals before fasting
- •
Fasting of at least 4 hours
- •
Intravenous heparin (50 IU/kg) 1 hour before imaging may be given if the patient only had one high-fat meal before fasting
On the other hand, for myocardial viability assessment, it is desirable to enhance myocardial 18 F-FDG uptake in order to detect the nonviable, nonglucose using myocardial tissue. For example, in patients with known myocardial infarction, increased myocardial glucose uptake can better differentiate viable and nonviable myocardium. In such instances, a carbohydrate-loading patient preparation protocol can be done on the day of imaging :
- •
Fasting of at least 6 hours
- •
One hour before imaging, 75 g of glucose to be administered orally
- •
Blood glucose level checked 30 minutes before imaging, with administration of intravenous insulin (low-dose sliding scale) if blood glucose level >150 mg/dL
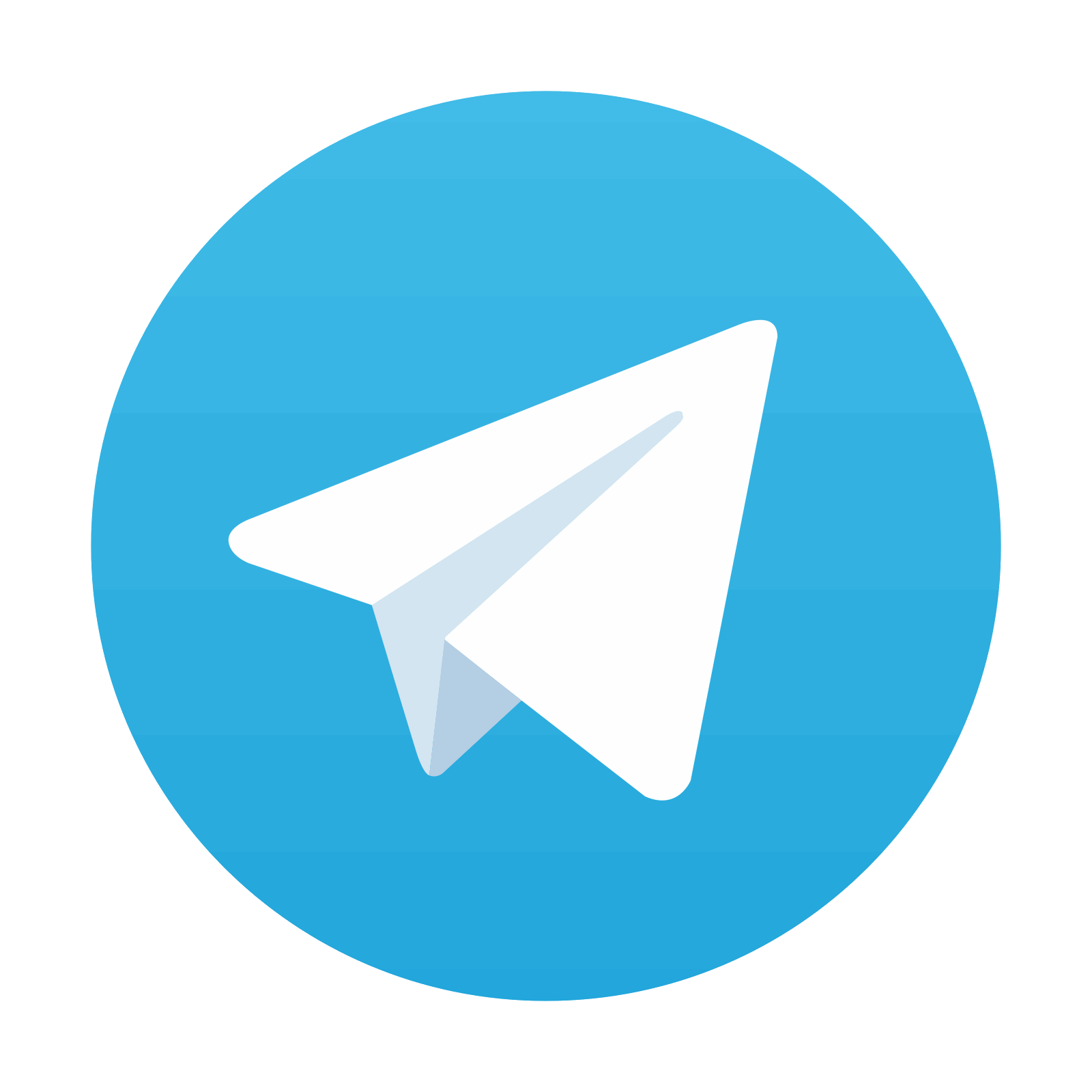
Stay updated, free articles. Join our Telegram channel

Full access? Get Clinical Tree
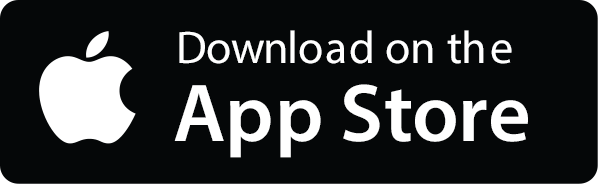
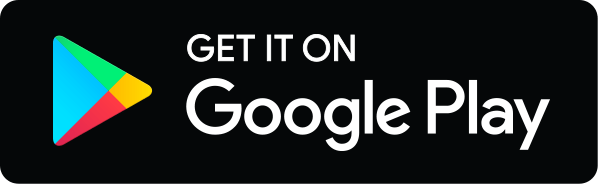