Central Nervous System Scintigraphy
David H. Lewis
Jon Umlauf
2-D (Planar Brain Scans)
Before the advent of CT, the planar nuclear medicine brain scan, using radiopharmaceuticals such as Technetium-99metastable (Tc-99m) diethylenetriaminepentaacetic acid (DTPA) or Tc-99m-glucoheptonate (GH), was used to detect a breakdown in the blood–brain barrier (BBB). Currently, planar brain imaging using agents that cross the intact blood brain barrier, such as Tc-99m-hexamethylpropyleneamine oxime (HMPAO) or Tc-99m-ethyl cysteinate dimer (ECD), can detect absence of cerebral blood flow, which is characteristic of brain death. The normal blood brain barrier protects the CNS by preventing entry of harmful substances. Most materials are excluded from the CNS on the basis of molecular size and chemical characteristics. Active transport mechanisms are present for certain key nutrients such as glucose.
Radiopharmaceutical. Planar brain scanning for the detection of the BBB breakdown is typically performed with either Tc-99m bound to DTPA or GH. Any agent that does not normally cross the blood brain barrier can potentially be employed, although agents of cellular size (e.g., radiolabeled red blood cells) will be excluded even by a damaged blood brain barrier.
Technique. A dose of 15 to 20 mCi of Tc-99m-DTPA or GH is injected into an arm vein. Flow images are typically obtained at a rate of one image every 3 seconds for a total of 60 seconds, with the camera anterior to the head. Anterior, posterior, and lateral static images are subsequently obtained; vertex images are often useful. These are obtained by placing the camera at the vertex of the skull. A lead collar is employed to exclude radiation from the radiopharmaceutical localized below the neck. Immediate static images are useful to evaluate blood pool abnormalities, whereas delayed static images after clearance of the background activity are of greater value to detect breakdown of the blood brain barrier.
Interpretation. Interpretation of the static images depends primarily on detecting or excluding radiopharmaceutical localization within the brain parenchyma. Some activity is invariably present from the radiopharmaceutical within the soft tissues of the scalp and within intracerebral blood vessels. Increased or asymmetric localization indicates breakdown of the BBB. This finding is entirely nonspecific, being present in conditions as diverse as cerebral infarction, primary or metastatic tumor, and infectious processes. For this reason, clinical information is essential for interpretation. The presence of a lenticular photo-enhanced (or occasionally photopenic) rim can be used to diagnose subdural hematoma.
The normal radionuclide angiogram is characterized by prompt symmetric perfusion. Asymmetric flow in the carotid arteries may indicate occlusive disease. The so-called flip-flop sign (decreased activity in the arterial phase and increased activity in the venous phase) may be seen in carotid occlusion. Vascular malformations, high-grade or vascular tumors, such as glioblastoma multiforme and meningioma, and inflammatory processes have increased flow. Low-grade or benign tumors, areas of porencephaly or edema, and occlusive processes have decreased flow. The complete absence of brain activity, including the cerebellum, in the presence of prompt common carotid and scalp flow is consistent with brain death.
The traditional brain scan with Tc-99m-DTPA or GH has largely been superseded by planar perfusion brain scans in clinical practice to corroborate the impression of brain death. There is little doubt that modern brain radiopharmaceuticals such as Tc-99m HMPAO or ECD provide with greater assurance, the status of cerebral blood flow. These scans are also much easier to interpret although more costly to perform due to the higher expense of the radiopharmaceuticals employed. Total cessation of the cerebral blood flow including posterior fossa structures can be demonstrated with this technique, which is required by the Uniform Determination of Death Act. (National Conference of Commissioners on Uniform State Laws, 1981) (1).
Brain Death. Criteria for Tc-99m brain perfusion radiopharmaceutical planar scanning has been retrospectively validated (2). Brain perfusion agents have advantages over conventional agents such as Tc-99m-GH or Tc-99m-DTPA and are not as dependent on the quality of bolus injection, are easier to interpret, and allow evaluation of posterior fossa blood flow (3,4). Radionuclide cerebral angiography without brain perfusion radiopharmaceuticals requires rapid acquisition of dynamic images in technically challenging situations and cannot image flow in posterior fossa. Interpretation may be difficult or equivocal.
Radionuclide scintigraphy is not affected by drug intoxication, hypothermia, or hypovolemia; however, these conditions do affect the clinical assessment of brain death. In the presence of brain death, the radioactive bolus stops at the base of the skull because of increased intracranial pressure that exceeds cerebral perfusion pressure. It is important to have a good bolus injection, and if distinct activity is not identified in the common carotid artery, the injection should be repeated. Absence of intracerebral arterial flow and no visualization of major venous sinuses on subsequent static images support a diagnosis of brain death (Fig. 62.1). The “hot nose sign,” due to increased collateral blood flow in the nasal area, could be a secondary sign in brain death, but is considered nonspecific.
Cerebrospinal Fluid Studies
Cerebrospinal fluid (CSF) is formed in the choroid plexus as an ultrafiltrate of plasma. It flows from the ventricles through the foramina of the fourth ventricle and ascends over the convexities of the brain to be absorbed by the arachnoid villa. Processes that impede flow over the convexities or absorption of the fluid by the villi result in communicating hydrocephalus. Tracer techniques are ideal for imaging of this process, because they are injected in small amounts and do not alter the CSF flow. Processes that obstruct the outflow from a ventricle are more difficult to assess by these techniques because injection can be made directly into the ventricle. Patency and flow in the therapeutic shunts and reservoirs can easily be evaluated by injecting the tracer directly into the device.
Radiopharmaceuticals and Technique. The standard cisternogram is performed by intrathecal injection of a sterile, pyrogen-free radiopharmaceutical. The only approved agent currently marketed for this purpose is Indium (In)-111 DTPA (half-life = 2.8 days). The injection of 0.5 mCi follows a spinal tap performed in the standard manner. Initial images may be obtained to ensure intrathecal injection. Subsequently, the radiopharmaceutical ascends to the basilar cisterns in approximately 4 hours and flows over the convexities within 24 hours in a normal individual. Images of the basilar cisterns are obtained at 4 to 6 hours. If images at 24 hours show ascent over the convexities with activity in the interhemispheric fissure and relative clearance of the basilar cisterns, imaging may be terminated. Otherwise, images should be obtained at 48 and 72 hours (5).
Application to Hydrocephalus. Standard cisternography is performed primarily to evaluate for normal pressure hydrocephalus and for CSF leak. Normal pressure hydrocephalus is a form of communicating hydrocephalus clinically associated with ataxia, dementia, and urinary incontinence. Cisternography demonstrates early localization of activity within the lateral ventricles, persisting beyond 24 hours and delayed clearance over the convexities (Fig. 62.2). Although these findings indicate an increased likelihood of a clinical response to shunting, they are not univariate predictors of outcome (6). Other forms of communicating hydrocephalus (such as might result from radiation therapy or intrathecal chemotherapy) can also be evaluated with cisternography.
Application to CSF Leak. Cisternography has high sensitivity for CSF leak and remains the procedure of choice for this condition. The sensitivity results from the ability of tracer technique to detect very small amounts of activity that may be intermittently leaking. Imaging is performed between 1 and 3 hours after injection and also at 24 and perhaps 48 hours. Patient and camera position are chosen to maximize the likelihood of detection, with lateral views for CSF rhinorrhea and anterior views for CSF otorrhea. Cotton pledgets should be placed in the nostrils after intrathecal injection of tracer. These are counted at 4 to 6 hours in a well counter. A serum sample from peripheral blood drawn concurrently is also counted. Pledget activity exceeding 1.5 times the serum concentration is evidence for CSF rhinorrhea (7). However, because this imaging method does not give morphologic information about the leakage, DiChiro et al. suggested use of imaging as well (8).
Application to Shunts and Reservoirs. CSF shunt and reservoir studies are performed by direct injection of radiotracer into the device. The demonstration of a patent ventriculoperitoneal, ventriculoatrial, or ventriculopleural shunt is an important study for neurosurgeons contemplating shunt revision. Ommaya reservoirs for delivering chemotherapy can be evaluated in this manner as well.
Radiopharmaceuticals and Technique. While In-111 DTPA and Tc-99m-DTPA are acceptable agents, Tc-99m-pertechnetate can also be used. A small volume of radiopharmaceutical such as 0.1 to 0.2 mCi Tc-99m-pertechnetate in 0.1 mL of normal saline is typically administered. Maintenance of sterile techniques during the injection is critical. It is also critical to understand the specific device being evaluated, as shunts often contain check valves and reservoir capacities with various specifications. A patient may also have several shunt tubes, some of which may be known to be occluded. In general, it is best to have direct input from the neurosurgeon involved in the case to ensure that the maximum amount and correct information is obtained. The shunt reservoir can be accessed using a butterfly needle and the opening pressure of the CSF obtained. Then, using the same tubing, a small amount of CSF, up to 0.5 to 1 cc may be withdrawn for lab studies before the tracer injection. Before injection of the radiotracer, the patient is moved under the camera. Dynamic gamma camera imaging for 10 minutes is usually first performed in the supine position. If radiotracer drains from the reservoir, then in cases of ventriculoperitoneal shunts, an abdominal image is obtained. If the shunt leads elsewhere, such as atrium, images of thyroid and chest are done. When there is lack of spontaneous drainage in the supine position, provocative drainage maneuvers such as sitting the patient upright, walking the patient for 1 to 2 minutes, or pumping the shunt reservoir 3 to 5 times may demonstrate shunt patency. When shunt patency requires provocative maneuvers, the shunt function may be described as positional. Positional shunts have occasionally been found to be obstructed at revision.
Shunts are evaluated primarily for patency. If the proximal portion is occluded manually (or contains a check valve), flow through the distal limb can be evaluated. The tracer should flow freely into the peritoneum (for ventriculoperitoneal shunts). Delayed flow or persistent activity at the shunt tip suggests malfunction. Diffusion into the peritoneum confirms a patent shunt system. A T1/2 of clearance from the reservoir can be calculated and should usually be less than 8 minutes (9). If the CSF flow is disturbed and opening pressure is low, the blockage is in the proximal limb. If opening pressure is high (more than 20 cm H2O), the obstruction is distal to the reservoir.
In instances of distal malfunction of the shunt system, the clearance curve is flat and the half-time clearance of the reservoir indicates infinite values. For complete distal obstruction or rupture of abdominal catheter, the isotope fails to migrate through the tubing, whereas in partial distal obstruction due to relatively high abdominal pressure or obstruction of the valve, extremely low radionuclide clearance is encountered. Peritoneal loculations are typically characterized by stagnation of radioactivity at initial site of appearance within the abdominal cavity and absence of uniform diffusion in the peritoneum.
Demonstration of shunt patency depends on many factors, including patient’s CSF production, proportion of CSF circulating through normal pathways, resting intraventricular pressure, patient’s position before the test, opening pressure of the shunt system, length of tubing, and variations of intraventricular pressure due to coughing, straining, or crying.
Demonstration of proximal limb flow is not necessary to prove shunt patency (10). Inability to measure intraventricular pressure, to aspirate CSF freely, or to inject the isotope into the ventricle was considered of some help in assessing proximal shunt obstruction.
Overshunting is also described when the opening pressure is low and T1/2 in the supine position is less than 1 minute. Overshunting may be an indication for shunt valve adjustment or possibly shunt revision. Typically, patients with overshunting have headache that progresses in severity from morning to evening.
Cerebral Perfusion Imaging
Cerebral perfusion imaging is one of many recent advances in functional neuroimaging. Anatomic studies fail to provide functional information that can be used to localize variations in regional blood flow that define many disease states. Radiopharmaceuticals used to study regional cerebral blood flow must have three properties to be effective. They must cross the blood-brain barrier, their extraction ratio must be
very high, and largely stable at varying rates of flow so that their initial distribution will be proportional to regional cerebral blood flow, and they must be retained within the brain in their initial distribution long enough for statistically valid tomographic images to be obtained. The radiotracers are lipophilic to cross the BBB by passive diffusion with nearly complete first pass extraction in the brain. The agents in widespread use for SPECT imaging are Tc-labeled agents such HMPAO and ECD. The PET agent most commonly used for brain blood flow is O-15 water that has a 2-minute T1/2 that requires an onsite cyclotron.
very high, and largely stable at varying rates of flow so that their initial distribution will be proportional to regional cerebral blood flow, and they must be retained within the brain in their initial distribution long enough for statistically valid tomographic images to be obtained. The radiotracers are lipophilic to cross the BBB by passive diffusion with nearly complete first pass extraction in the brain. The agents in widespread use for SPECT imaging are Tc-labeled agents such HMPAO and ECD. The PET agent most commonly used for brain blood flow is O-15 water that has a 2-minute T1/2 that requires an onsite cyclotron.
Positron Emission Tomography
Receptor Imaging. Neuroimaging with PET allows qualitative and quantitative evaluation of receptor systems within the brain. Adrenergic, cholinergic, dopaminergic, serotonergic, benzodiazepine, and opioid receptors have been extensively evaluated. PET allows true biochemical assessment of properties of these receptor systems, such as affinity, saturation and nonspecific bindings, as well as more general information about distribution and uptake kinetics. These unique capabilities of the PET technique provide an extremely valuable research tool for evaluation of brain biochemistry and development of both imaging agents and therapeutic pharmaceuticals. However, these studies are expensive, experimental, and time consuming. Experimental uses should be clearly differentiated from proven clinical indications; the associated ethical issues have been assessed and summarized by the Brain Imaging Council of the Society of Nuclear Medicine (11).
Metabolic Imaging. F-18 FDG-PET is an indirect marker of neuronal activity and allows for quantification of cerebral glucose metabolism. Clinical indications for F-18 FDG-PET include the evaluation of epilepsy, dementia, glioma, and traumatic brain injury. PET use in epilepsy is predominantly in the presurgical evaluation of partial complex seizures that fail to respond to a trial of antiepileptic drugs. In mesial temporal lobe epilepsy, up to 85% of these patients can be cured surgically if the focus of the abnormal brain is limited to a single temporal lobe. FDG scanning must be performed interictally in seizure patients, as the 1.83-hour half-life of F-18 does not allow the radiotracer to be held available for ictal scanning. Interictally, the seizure focus is usually hypometabolic. This is now thought to be due to interruptions with adjacent neurons, which reduces neural activity and thus metabolism. Loss of neural connections can also result in decreased metabolism in connected and more distant sites (diaschisis). Sites of temporal epilepsy are identified as hypometabolic foci in 70% to 85% (12) of interictal scans with a false-positive rate of only 5% (13). Dementia evaluation is predominantly performed to separate dementia of Alzheimer type from other causes of dementia such as frontotemporal lobe dementia, vascular dementia, and pseudodementia due to depression. While the only unequivocal test remains brain biopsy, detection of Alzheimer disease (AD) with PET has a sensitivity and specificity of 78% to 83% and 78% to 94%, respectively (14), and is considered very reliable when applied to an appropriate population at risk. Patterns of activity with F-18 FDG are similar to those described for SPECT agents subsequently in this review. The role of PET and SPECT scanning in evaluation of brain tumors has been discussed elsewhere and will not be repeated here. Although PET is certainly a useful technique in each of these considerations, there are techniques available for SPECT imaging in each of these conditions, which have similar sensitivity and specificity at decreased cost (15). SPECT cameras and the appropriate imaging pharmaceuticals are more likely to occupy a continuing role in routine clinical application of functional brain imaging. For this reason, the technique and interpretation of these studies is not discussed in detail. The interested reader is referred to one of the many excellent reviews available for PET brain imaging (16).
Single-Photon Emission Computed Tomography
Radiopharmaceuticals. Much of the early work in this area was performed with Xe-133. This inhaled gas dissolves in blood to an extent adequate for imaging. The rapidity of perfusion and diffusion of this agent makes rapid imaging essential. Therefore, multiprobe-type cameras have predominantly been employed. This tracer is not well suited to rotating camera SPECT technique. For this reason, and because of difficulties in handling and recovering a gaseous agent, this agent has largely been superseded by other radiopharmaceuticals.
Iodinated amphetamines tagged with iodine 123 replaced radioxenon for a time. These agents readily cross the blood brain barrier. Both uptake and blood brain barrier diffusion are reversible. This agent, therefore, will slowly redistribute over time. Iodoamphetamine is also immediately sequestered by and slowly released from the lung. This effectively yields slow intra-arterial injection over a period of hours. Because of these phenomena, iodoamphetamine images represent integration of all brain activity from the time of injection until completion of imaging. Because iodine-123 is cyclotron produced and has a relatively short half-life (13.2 hours), availability in the past has proved problematic. This agent also has been largely superseded and is no longer commercially available in the United States. Agents currently in widespread use include Tc-99m-labeled HMPAO (exametazime/HMPAO) and ECD (bicisate/ECD).
Tc-99m-HMPAO is an agent of the chemical microsphere type. This agent crosses the blood brain barrier and is trapped within the brain substance. The mechanisms proposed for trapping have included change in the ionic state, binding to glutathione, and chemical decomposition (17). For purposes of scan interpretation, it is only necessary to understand that this agent essentially crosses the blood brain barrier irreversibly. Unlike iodinated amphetamine, this agent provides a “snapshot” of brain activity for a short period after injection (<10 minutes, with peak activity usually within 1 minute from IV injection). The HMPAO is available as a kit that is combined with the generator-produced, freshly eluted pertechnetate before use. Availability is thus not problematic. The initial form of this agent was unstable in aqueous solution. It had to be used within 30 minutes after preparation, which made quality control procedures difficult. A stabilized form is now available, which can be used for 4 hours after aqueous preparation.
Tc-99m-ECD is also the agent of the “chemical microsphere” type (18). This agent, unlike Tc-99m-HMPAO, tends to not localize in areas of luxury perfusion (19) (Fig. 62.3), although there are rare exceptions (20). Although there are a number of subtle differences between Tc-99m-ECD and HMPAO, the remaining differences are not of routine clinical relevance. Tc-99m ECD is stable in aqueous solution for at least 6 hours and is therefore preferable when attempting ictal injection and scanning of seizure disorders due to this practical issue of stability. Tc-99m-HMPAO does have a slightly better extraction efficiency at higher flow rates than ECD. Both agents have their proponents and are in common use (21).
Technique. Brain perfusion SPECT scans are preferably performed with a multidetector rotating camera. A dual head-rotating camera can be employed, but single head cameras are not generally recommended at this time. Cameras limited to brain work are not generally necessary due to improvements in equipment since the first edition of this book, although they
may be useful in practices with a large brain scanning referral base. High-resolution collimators should be employed (22). The key issues for imaging are distance from the brain to the detector head and total counts acquired. One should try to achieve the smallest possible radius of rotation camera but remaining cognizant of patient comfort and safety.
may be useful in practices with a large brain scanning referral base. High-resolution collimators should be employed (22). The key issues for imaging are distance from the brain to the detector head and total counts acquired. One should try to achieve the smallest possible radius of rotation camera but remaining cognizant of patient comfort and safety.
Before the procedure, the physician should review history, recorded neurologic physical examination, and a mini mental status examination if available. Historical data of importance include symptoms and duration, history of stroke, head injury or seizure, any medications (especially psychotropic anticonvulsants), and whether CT or MR scans have been performed. Neurologic examination should include cognitive and motor examination and cranial nerve examination. Mental status examination should address orientation, registration, attention, recall, and language functions.
The scanning agent is injected with a patient in a controlled resting state. This usually involves a supine, resting patient with closed eyes in a quiet room (or a room with low-level noise) and indirect lighting (see Society of Nuclear Medicine procedure guidelines). Alternatively, tracer can be injected with seizure ictus or acute stroke. The intravenous line should be established in advance and all instructions and questions should be dealt with before injection to avoid unintended stimulation of brain activity. The patient should remain in this controlled environment for at least 5 minutes after injection. A measure of 15 to 30 mCi of Tc-99m-HMPAO or Tc-99m-ECD should be employed (0.2 to 0.3 mCi/Kg in pediatric patients). A delay after injection of no less than 60 minutes and preferably 90 minutes should be employed with Tc-99m-HMPAO to allow for background clearance. No less than 10 minutes and preferably 60 minutes should elapse before Tc-99m-ECD imaging with SPECT. Quality control on the radiopharmaceutical should be performed before injection, according to the package insert. Careful patient monitoring is mandatory throughout the scan, because patients considered for the study often have dementia, neurologic dysfunction, stroke, or another condition limiting cooperation, which would require monitoring.
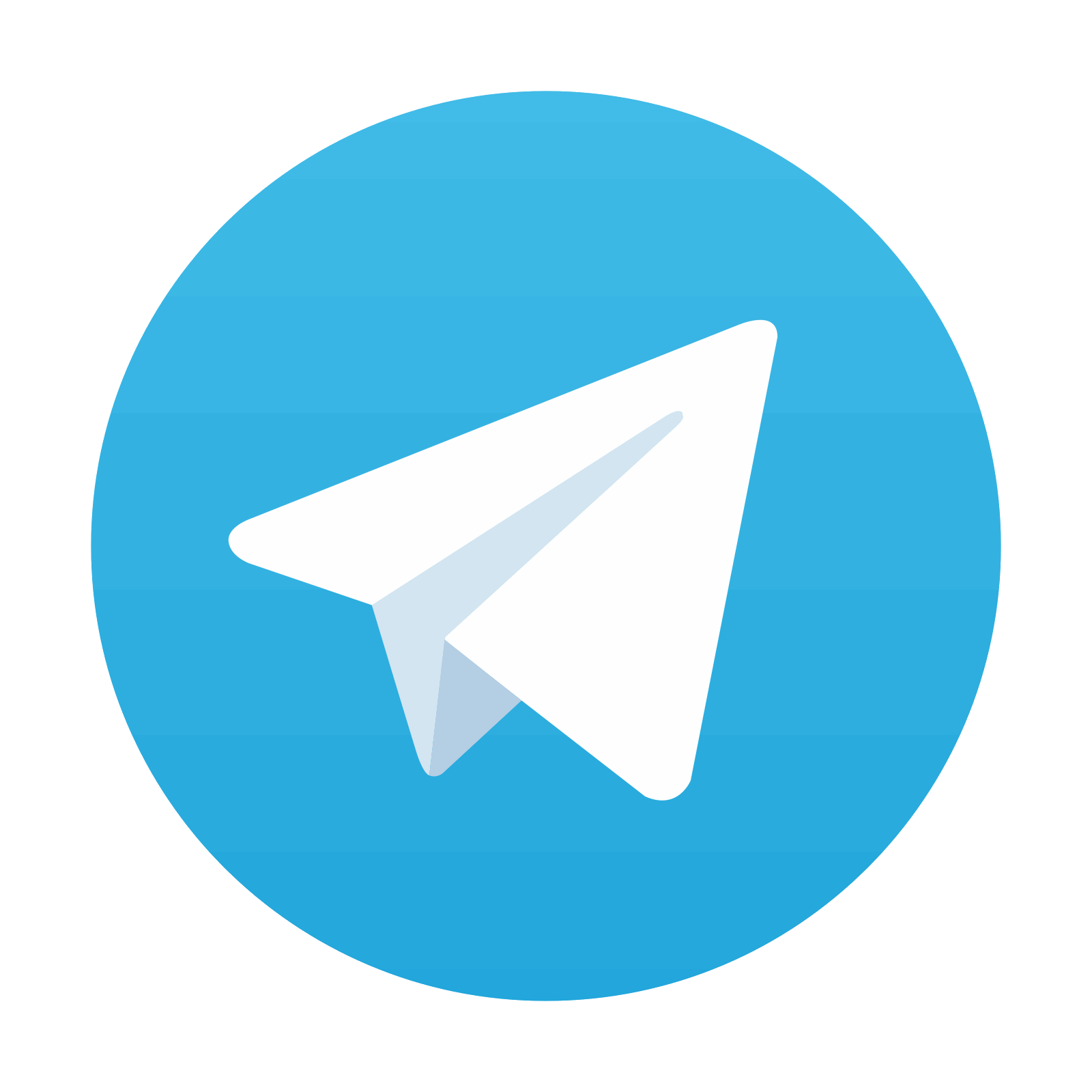
Stay updated, free articles. Join our Telegram channel

Full access? Get Clinical Tree
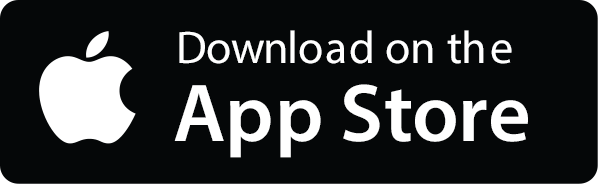
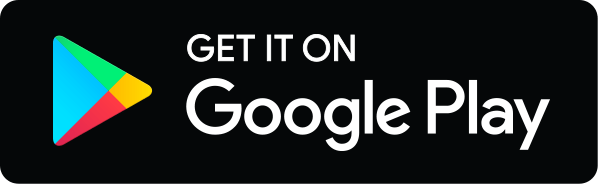