FIGURE 13.35 Synovial cyst. T2-weighted fat-suppressed A: axial and B: coronal MR images show a polylobulated mass with high signal intensity with a linear component (arrowhead) that extends to the anterior elbow joint. C: T1-weighted fat-suppressed MR image after the administration of intravenous contrast shows low signal intensity within the lesion verifying that it is a simple fluid collection without solid components.
FIGURE 13.36 Primary synovial osteochondromatosis. A: T1-weighted coronal, B: T1-weighted sagittal, and C: T2-weighted fat-suppressed axial MR images through the elbow show multiple, uniformly sized intra-articular ossified bodies (curved arrows) evenly distributed throughout the joint. There is superimposed degenerative change in the radiocapitellar (arrowhead) and ulnotrochlear joints (arrow).
MRI is the imaging method of choice for the diagnosis, surgical planning, and evaluation of recurrence. Although not pathognomonic, MRI features in PVNS provide the diagnosis in over 95% of cases. Clumps of hemosiderin-laden macrophage deposits demonstrate low signal intensity on T1- and T2-weighted images as a result of the paramagnetic effects of the iron in the ferric (3+) state. This is the most reliable diagnostic feature. Gradient imaging, like in the case of hemophilia, may further enhance imaging of these findings as a result of the magnetic susceptibility effects (154, 155). A large joint effusion is usually present but is nonspecific in nature.
Less characteristic MRI findings in PVNS include bone erosions and extra-articular spread of lesions. T2-hyperintense signal may also be seen as the result of subchondral cysts or reactive bone marrow edema. Additionally, focal deposits of lipid-laden macrophages can result in fat signal intensity.
In addition to facilitating the diagnosis, MRI is also useful in characterizing the extent of disease by the detection of extra-articular spread as well as assessing the status of the adjacent ligamentous and fibrocartilaginous structures. Common extra-articular sites of spread include the popliteus tendon sheath, posterior to the posterior cruciate ligament, the semimembranosus bursa, and previous arthroscopy portals.
The treatment of diffuse PVNS is a complete synovectomy. The high recurrence rate, reported to range from 33% to 46%, precludes localized surgical treatment. The exact reasons for such a high rate of recurrence is unclear but may be related to the difficulty in performing a complete synovectomy. A recent hypothesis also suggests a high rate of recurrence resulting from the failure to identify and resect extra-articular lesions. The latter hypothesis, if correct, increases the importance of carefully addressing the bursa and tendon sheaths about the joint (156).
Focal Nodular Synovitis
Localized nodular synovitis, like its more diffuse counterpart, PVNS, is a rare synovial inflammatory disorder whose etiology is uncertain. Although this disorder shares similar histopathologic characteristics and patient population as PVNS, the MRI characteristics of the localized form are distinct from PVNS. Moreover, the reader should be aware that it is of paramount importance to distinguish these two entities because their surgical planning, treatment, and response to treatment differ dramatically (154, 158, 159).
The MRI characteristics of localized nodular synovitis vary. The classic appearance is a rounded or ovoid solitary lesion that demonstrates different degrees of low signal on T1- and T2-weighted images depending on the concentration of hemosiderin occupying the lesion. The lesion typically is more conspicuous on gradient imaging as a result of the paramagnetic effect of iron molecules in the ferric state. There is usually heterogeneous enhancement after the intravenous administration of contrast material, which likely relates to the rich capillary network occupying the fibrous stroma (154).
The most commonly affected regions are tendon sheaths about small articulations of the fingers and toes. When intra-articular disease is present, it is almost always localized to the knee. Specifically, the most common location is the infrapatellar (Hoffa’s) fat pad. Other affected areas about the knee are the suprapatellar pouch and the intercondylar notch.
The importance of distinguishing localized nodular synovitis from PVNS cannot be overemphasized. MRI of PVNS usually shows a more exuberant pattern of hemosiderin deposition manifested as frond-like low-signal irregularities residing within the joint spaces. This finding is absent in localized nodular synovitis; in fact, only a small portion of the synovium is involved. In addition, PVNS-induced masses insinuate themselves throughout the articulation resulting in marked constriction. The localized form manifests as a solitary mass, which grows outward and may have an associated pedicle. In a recent study by Huang et al. (158), it has been noted that when the lesion grows in excess of 5 cm, knee extension is more likely to be restricted. Joint effusions in this disease are usually small or absent.
The treatment of choice for localized nodular synovitis is local excision of the solitary mass. When compared with PVNS, localized nodular synovitis never requires a total synovectomy and the rate of recurrence is exquisitely low, usually less than 5%. Excision can usually be accomplished with arthroscopic guidance; however, an open procedure may be warranted in certain instances.
Amyloidosis
Amyloidosis is an infiltrative disorder characterized by the deposition of a pathologic proteinaceous substance (amyloid) in various tissues and organs in the body ultimately resulting in physiological derangement. Amyloidosis is further characterized into multiple subtypes depending on the chemical nature of the protein that makes up the amyloid fibril. Fifteen different biochemical subtypes of amyloid have been described to date. The histopathologic appearance, however, is uniformly characterized by apple green birefringence of Congo red-stained amyloid on polarized light microscopy irrespective of the biochemical subtype. The most common biochemical subtype in amyloid arthropathy is B2 microglobulin (160–162).
Amyloid arthropathy has been well documented as a complication in patients on long-term hemodialysis. This disorder develops as a result of accumulation of high concentrations of the normal serum protein B2 microglobulin in the synovium, bone, and soft tissues about the joint. Although amyloid deposition can be histologically documented in the joints of patients on short-term hemodialysis, radiographic manifestations usually occur at least 5 years after treatment begins. Joint involvement is bilateral and fairly symmetric and although any joint may be involved, this entity has a predilection for the shoulders, wrists, hips, and knees but can occur in any articulation. MRI characteristics of amyloid are not unlike other deposition diseases that involve the synovium (160–162).
MRI demonstrates soft tissue nodules of low to intermediate signal on all pulse sequences with associated joint effusions. These nodules are most commonly seen around the elbow, hand, and wrist. In fact, amyloid deposition has been implicated as the cause of carpal tunnel syndrome in patients on long-term dialysis.
Additionally, discrete periarticular intraosseous lytic foci and subchondral cystic changes are also a characteristic finding on conventional radiographs. On MRI, these lesions are dark on T1-weighted images and dark, bright, or intermediate signal on T2-weighted images. The exact nature of these lesions is not known. Some experts speculate that these lesions may represent focal amyloid deposits. Others believe these are synovial fluid collections that develop as a result of extrinsic compression from the pericapsular soft tissue deposits.
Involvement of fibrocartilaginous structures has also been documented on MRI. Specifically, thickening of the supraspinatus and infraspinatus tendons of the rotator cuff as well as the iliofemoral portion of the hip capsule have been reported. Tendon rupture or tears, however, are not associated with this disease (160–162).
Tumors and Tumor-like Disorders of Soft Tissues
The evaluation of soft tissue tumors has been revolutionized by the advent of MRI with its excellent soft tissue contrast and multiplanar imaging capabilities. The primary objectives of the imaging evaluation of soft tissue lesions include the detection of the suspected lesion and the formulation of a diagnosis or set of differential considerations. Despite sophisticated imaging techniques such as MRI, the imaging appearance of the majority of soft tissue lesions remains nonspecific with a correct histologic diagnosis reached on the basis of imaging studies alone in only approximately one fourth to one third of cases (163–166). The majority of soft tissue lesions in the upper extremity are nonspecific to this region. Most of the lesions that do show a predilection for the upper extremity are unique to the hand and wrist. Although a complete discussion of soft tissue lesions is outside the scope of this work, a brief discussion of lesions that have characteristic MRI findings or are relatively specific to the region of the elbow follows. With regard to the former, often the tissue of origin in soft tissue lesions can be surmised by signal characteristics, morphology as well as proximity to adjacent structures. In addition, the soft tissue elements common to most regions of the body include fat, vascular structures, nerve tissue, muscle, and synovium.
FIGURE 13.37 Intramuscular lipoma. A: Coronal and B: axial T1-weighted MR images through demonstrate a high-signal–intensity mass (curved arrow) within the supinator muscle.
Lipoma
Although not specific to the elbow, the lipoma is the most common soft tissue tumor (Fig. 13.37). It is a benign lesion composed of mature adipose tissue. Soft tissue lipomas are categorized by anatomic location as either superficial (subcutaneous) or deep, the former being much more common. After the trunk, the shoulder and upper arm is the most common location. They are unusual in the hand and foot. These lesions present in middle age (fifth and sixth decades of life), although there is a wide spectrum of age at presentation. They generally present as painless, slow-growing masses. On physical examination, they are soft, usually encapsulated, with a distinct lobular pattern.
The MRI characteristics are those of a well-defined mass with signal characteristics that parallel fat on all pulse sequences. There is no discernible enhancement after the administration of intravenous gadolinium (147).
Differentiation from low-grade liposarcoma can be challenging. The presence of thick, irregular internal septation, internal nodularity, or patchy nonadipose elements within the lesion suggest possible malignancy. Internal enhancement after intravenous gadolinium administration may also correlate with low-grade malignancy.
Vascular Lesions
The angiomatoses are a common cause of soft tissue mass. Included in this category are hemangiomas and lymphangiomas, glomus tumors, hemangioendotheliomas, hemangiopericytomas, angiosarcomas, and Kaposi’s sarcoma (165). The classification of these lesions is somewhat controversial with very different approaches discussed in the literature. Both systems are presented, the first in the setting of elbow lesions and the second approach discussed in the setting of finger lesions.
The first approach considers hemangioma one of the most frequent soft tissue tumors and advocates a classification system based on the type of vascular channel that dominates the histologic appearance. Capillary hemangiomas are comprised solely of capillaries and are generally located in the superficial soft tissues. If histologic analysis shows widely dilated capillaries, the tumor is called a cavernous hemangioma. Cavernous hemangiomas predominate in the deep soft tissues and commonly contain calcifications in the form of phleboliths. If a vascular tumor has thicker walls and contains smooth muscle cells, it is called a venous hemangioma. Venous hemangiomas are often deep and involve the muscles of the extremities or retroperitoneum (Fig. 13.38). Slow blood flow typifies venous hemangiomas, and phleboliths may be seen. When histologic analysis shows abnormal communication of arteries and veins, the term arteriovenous hemangioma or arteriovenous malformation is often used. This type of lesion is often associated with shunting of blood and high blood flow (167–170).
Based on this classification system, MRI findings of such vascular lesions typically show poorly marginated lesions comprised of tubular structures. These structures are isointense relative to muscle on T1-weighted MR images. Areas of high signal intensity can also be seen on T1-weighted MR images that could be related to interposed fat or to slow-flowing blood. Fat overgrowth can be encountered in vascular neoplasms and, in some cases, can be so prominent as to be indistinguishable from lipoma. On T2-weighted MR images, soft tissue hemangiomas reveal a well-marginated mass with very high signal intensity in areas of vascular components, whereas regions of adipose tissue are intermediate intensity and isointense to subcutaneous fat. In some cases, pathologic subtypes can be recognized by MRI. A mass composed primarily of large vascular spaces suggests a cavernous hemangioma, whereas a venous hemangioma shows predominantly serpentine vascular channels. Hemangiomas show prominent enhancement after intravenous administration of gadolinium. Hemorrhage also occurs in hemangiomas. Phleboliths, as previously noted, are also commonly encountered but better identified by radiographs or computed tomography. On MRI, they appear as low-signal–intensity foci on all pulse sequences. If the hemangioma is located adjacent to bone, periosteal reaction can occur (167–170).
FIGURE 13.38 Vascular lesion. A: T2-weighted fat-suppressed axial and B: T1-weighted coronal MR images through the forearm demonstrate a large intramuscular mass with serpentine areas of high signal intensity and interspersed fat on the T1-weighted images. Based on the location and the dilated nature of the tubular high-signal–intensity structures (arrowheads), the diagnosis of venous hemangioma was suggested and confirmed on pathology. C: T1-weighted fat-suppressed axial MR image after the administration of intravenous contrast shows enhancement of the tubular structures (arrowheads) consistent with the vascular nature of the lesion.
Neurogenic Lesions
Neoplasms of neurogenic origin are not uncommon soft tissue tumors. They account for approximately 12% of all benign and 8% of all malignant soft tissue neoplasms (167–172). In many cases, imaging characteristics suggest aneurogenicorigin. These features include the ability to visualize a nerve entering or exiting the lesion as well as MRI signal characteristics both before and after the intravenous administration of gadolinium. Benign peripheral nerve sheath tumors are typically divided into two major groups: schwannoma and neurofibroma, schwannoma slightly less common than neurofibroma.
Schwannomas are found commonly in the cutaneous nerves of the head and neck and the flexor surfaces of the extremities, especially the peroneal and ulnar nerves. They are slow-growing, nonaggressive neoplasms that present as painless masses (usually less than 5 cm in size) without neurologic symptoms. They are solitary in the vast majority of cases. In 5% of cases, they can be plexiform and associated with von Recklinghausen disease. Pathologically, a schwannoma has a true capsule composed of perineurium. When large nerves are affected, the tumor is eccentric with the nerve displaced to the periphery of the mass. Histologically, schwannomas are composed of intermixed Antoni A (cellular and arranged in short bundles or interlacing fascicles) and Antoni B (less cellular and organized with more myxoid component) areas. Degenerative changes such as cyst formation, calcification, hemorrhage, hyalinization (fibrosis) can be seen in schwannomas, which are then labeled ancient schwannomas (165, 173).
Neurofibromas are divided to solitary (the vast majority) or those associated with neurofibromatosis 1 (von Recklinghausen disease). Neurofibromas, similar to schwannomas, are slow-growing, painless masses located in superficial tissues and often less than 5 cm in size. In contradistinction to schwannoma, however, these lesions lack a capsule. Pathologically, these lesions cannot be separated from the nerve. Microscopically, they contain interlacing bundles of elongated cells with wavy, darkly staining nuclei without Antoni A and B areas as noted in schwannoma (165, 173).
With MRI, these lesions show a fusiform shape, and a nerve is often identified entering or exiting the mass as a tubular structure (165, 174, 175). Long-axis MRI is optimal to illustrate this appearance. In theory, distinction between neurofibroma and schwannoma should be possible with MRI. Classically, the nerve is eccentric to the mass in schwannoma rather than being central to it or obliterated by it in the setting of neurofibroma. Some studies report the ability to identify the exact nerve/mass relationship in 65% of cases (175). Some neurogenic tumors have a discrete margin, and thin, low-signal–intensity rim representing a fibrous pseudocapsule (175). This is more frequent with schwannoma. Many neurogenic tumors also have a peripheral rim of fat (176). This may result from their origin in the neurovascular bundle and subsequent centripetal growth allowing the rim of surrounding fat to remain intact. This finding is referred to as the “split-fat” sign and is seen with both schwannoma and neurofibroma. MR images of neurogenic neoplasms show signal intensity similar to or slightly higher than that of muscle on T1-weighted images. Typically, there is high signal intensity on corresponding T2-weighted images with variable heterogeneity. In some cases, a central low-signal–intensity region on T2-weighted images with a surrounding rim of high signal intensity can be identified within the lesion (Fig. 13.39). This is referred to as the target sign and corresponds pathologically to central fibrous components and peripheral myxomatous elements within the lesion (174, 177, 178). The target sign is most frequently encountered in neurofibromas. Careful inspection of intermediate- to low-signal–intensity areas of the lesions on proton density- and T2-weighted images often reveal small ring-like areas with slightly higher signal intensity in their periphery. This is the so-called fascicular sign and the oretically reflects fascicular bundles of tissue seen in both neurogenic tumors and normal nerves (165). Enhancement of neurogenic lesions after the administration of intravenous contrast material is both variable and nonspecific (Fig. 13.40). Both benign and malignant peripheral nerve sheath tumors can show minimal to marked enhancement either focally or diffusely (179). Muscle atrophy can be associated with neurogenic neoplasms. It is usually noted in a distal muscle group and is manifested as increased amounts of fat within the muscle on T1-weighted images. Stull et al. (179) noted distal muscle atrophy in 23% of peripheral neurogenic tumors.
Cat Scratch Disease
Cat scratch disease is an infectious disorder that occurs frequently in children and adolescents. The entity is characterized by lymphadenitis and the formation of pustules or papules after the scratch of an animal, usually a cat. In most instances, the diagnosis is facilitated by the characteristic clinical history.
After an incubation period of 7 to 12 days, one or more red papules develop at the site of cutaneous inoculation, often within the line of the previous scratch. They persist until the development of lymphadenopathy, which generally occurs in 1 to 4 weeks. Chronic lymphadenitis is the hallmark of cat scratch disease. Sites most frequently involved include the axillary, cervical, submandibular, preauricular, epitrochlear, femoral, and inguinal lymph node groups. On MR images, an ill-defined mass with surrounding edema in the epitrochlear region of the elbow suggests epitrochlear lymphadenopathy. On T2-weighted images, the lymph nodes are high signal but have a nonspecific appearance (Fig. 13.41) (110, 180, 181). Involvement of more than one node group occurs in 10% to 20% of cases. Affected nodes are often tender, red, and indurated. Between 10% and 40% of nodes eventually suppurate with sinus tract formation to the skin surface. The duration of enlargement is 4 to 6 weeks, in some cases persisting up to 12 months. The cause is by a pleomorphic Gram-negative bacillus (Bartonella henselae). This disease was first described in 1950 by Debre et al. The diagnosis is generally made on clinical grounds by verifying the presence of at least three of the following four features (diagnostic criteria): (i) history of exposure to animal scratch; (ii) demonstration of dermal or ocular inoculation; (iii) presence of a single or regional area of lymphadenopathy; and (iv) a positive cat scratch disease skin test. Other criteria include biopsy of the skin lesion or lymph nodes with demonstration of histopathologic findings characteristic of the disease (polymorphonuclear cells, inflammation with possible necrosis, clusters of epithelioid histiocytes) (182–184).
FIGURE 13.39 Schwannoma with target sign. A: T1-weighted, B: T2-weighted, and C: T1-weighted axial postintravenous contrast MR images show a large median nerve mass (arrowhead) with variable central signal intensity (curved arrow) consistent with the target sign.
FIGURE 13.40 Stump neuroma. A: T1-weighted coronal MR image shows a forearm amputation in a patient presenting with stump pain. B: T2-weighted fat-suppressed axial and C: T1-weighted fat-suppressed postintravenous contrast axial MR images show an enlarged radial nerve lesion (curved arrow) that enhances. Subtle heterogeneous high signal is noted in the muscles of the stump.
FIGURE 13.41 Cat scratch disease. A: T1-weighted axial and B: T2-weighted coronal MR images through the elbow show an elliptical mass (asterisk) with adjacent fluid collection (curved arrow). C: T1-weighted axial MR image acquired after the intravenous administration of contrast shows enhancement in the mass (asterisk) and confirms the presence of fluid collection (curved arrow). These findings are consistent with an enlarged epitrochlear lymph node with adjacent inflammatory fluid collection.
References
1. Dellon AL. Musculotendinous variations about the medial humeral epicondyle. J Hand Surg (Br). 1986;11:175–181.
2. Williams P, Warwick R, Dyson M, et al., eds. Gray’s Anatomy. New York, NY: Churchill Livingstone; 1989.
3. Morrey BF. The Elbow and Its Disorders. Philadelphia, Pa: WB Saunders; 1993:7–42.
4. Jamieson RW, Anson BJ. The relation of the median nerve to the heads of origin of the pronator teres muscle, a study of 300 specimens. Q Bull Northwest Univ Med Sch. 1952;26:34–35.
5. Boles CA, Kannam S, Cardwell AB. The forearm: anatomy of muscle compartments and nerves. AJR Am J Roentgenol. 2000;174:151–159.
6. Mazurek MT, Shin AY. Upper extremity peripheral nerve anatomy: current concepts and applications. Clin Orthop Relat Res. 2001;383:7–20.
7. Rosenberg ZS, Bencardino J, Beltran J. MR features of nerve disorders at the elbow. Magn Reson Imaging Clin N Am. 1997;5:545–565.
8. Spinner M. The arcade of Frohse and its relationship to posterior interosseous nerve paralysis. J Bone Joint Surg Br. 1968;50:809–12.
9. Bordalo-Rodrigues M, Rosenberg ZS. MR imaging of entrapment neuropathies at the elbow. Magn Reson Imaging Clin N Am. 2004;12:247–263, vi.
10. Rosenberg ZS, Beltran J, Cheung YY, et al. The elbow: MR features of nerve disorders. Radiology. 1993;188:235–240.
11. Maravilla KR, Bowen BC. Imaging of the peripheral nervous system: evaluation of peripheral neuropathy and plexopathy. AJNR Am J Neuroradiol. 1998;19:1011–1023.
12. Kim YS, Yeh LR, Trudell D, et al. MR imaging of the major nerves about the elbow: cadaveric study examining the effect of flexion and extension of the elbow and pronation and supination of the forearm. Skeletal Radiol. 1998;27:419–426.
13. Bozentka DJ. Cubital tunnel syndrome pathophysiology. Clin Orthop Relat Res. 1998;351:90–94.
14. Gelberman RH, Yamaguchi K, Hollstien SB, et al. Changes in interstitial pressure and cross-sectional area of the cubital tunnel and of the ulnar nerve with flexion of the elbow. An experimental study in human cadavera. J Bone Joint Surg Am. 1998;80:492–501.
15. Fritz RC, Boutin RD. Magnetic resonance imaging of the peripheral nervous system. Phys Med Rehabil Clin N Am. 2001;12:399–432.
16. Kuntz C 4th, Blake L, Britz G, et al. Magnetic resonance neurography of peripheral nerve lesions in the lower extremity. Neurosurgery. 1996;39:750–756; discussion 756–757.
17. Murphey MD, Smith WS, Smith SE, et al. From the archives of the AFIP. Imaging of musculoskeletal neurogenic tumors: radiologic–pathologic correlation. Radiographics. 1999;19: 1253–1280.
18. Seddon H. Nerve injuries. Med Bull (Ann Arbor). 1965;31:4–10.
19. Ogino T, Minami A, Kato H. Diagnosis of radial nerve palsy caused by ganglion with use of different imaging techniques. J Hand Surg (Am). 1991;16:230–235.
20. Hashizume H, Nishida K, Nanba Y, et al. Intraneural ganglion of the posterior interosseous nerve with lateral elbow pain. J Hand Surg (Br). 1995;20:649–651.
21. Jinkins JR, Roeder MB. MRI of benign lumbosacral nerve root enhancement. Semin Ultrasound CT MR. 1993;14:446–454.
22. Lane JI, Koeller KK, Atkinson JL. Enhanced lumbar nerve roots in the spine without prior surgery: radiculitis or radicular veins? AJNR Am J Neuroradiol. 1994;15:1317–1325.
23. Sugimoto H, Ohsawa T. Ulnar collateral ligament in the growing elbow: MR imaging of normal development and throwing injuries. Radiology. 1994;192:417–422.
24. West GA, Haynor DR, Goodkin R, et al. Magnetic resonance imaging signal changes in denervated muscles after peripheral nerve injury. Neurosurgery. 1994;35:1077–1085; discussion 1085–1086.
25. Padua L, Aprile I, Caliandro P, et al. Natural history of ulnar entrapment at elbow. Clin Neurophysiol. 2002;113:1980–1984.
26. De Jesus R, Dellon AL. Historic origin of the ‘Arcade of Struthers.’ J Hand Surg (Am). 2003;28:528–531.
27. Kato H, Hirayama T, Minami A, et al. Cubital tunnel syndrome associated with medial elbow ganglia and osteoarthritis of the elbow. J Bone Joint Surg Am. 2002;84:1413–1419.
28. Matev B. Cubital tunnel syndrome. Hand Surg. 2003;8:127–131.
29. O’Hara JJ, Stone JH. Ulnar nerve compression at the elbow caused by a prominent medial head of the triceps and an anconeus epitrochlearis muscle. J Hand Surg (Br). 1996;21:133–135.
30. Vanderpool DW, Chalmers J, Lamb DW, et al. Peripheral compression lesions of the ulnar nerve. J Bone Joint Surg Br. 1968;50:792–803.
31. Britz GW, Haynor DR, Kuntz C, et al. Ulnar nerve entrapment at the elbow: correlation of magnetic resonance imaging, clinical, electrodiagnostic, and intraoperative findings. Neurosurgery. 1996;38:458–465; discussion 465.
32. Mobbs RJ, Rogan C, Blum P. Entrapment neuropathy of the ulnar nerve by a constriction band: the role of MRI. J Clin Neurosci. 2003;10:374–375.
33. Ruocco MJ, Walsh JJ, Jackson JP. MR imaging of ulnar nerve entrapment secondary to an anomalous wrist muscle. Skeletal Radiol. 1998;27:218–221.
34. Childress HM. Recurrent ulnar-nerve dislocation at the elbow. Clin Orthop Relat Res. 1975;108:168–173.
35. Spinner RJ, Goldner RD. Snapping of the medial head of the triceps and recurrent dislocation of the ulnar nerve. Anatomical and dynamic factors. J Bone Joint Surg Am. 1998;80:239–247.
36. Rolfsen L. Snapping triceps tendon with ulnar neuritis. Report on a case. Acta Orthop Scand. 1970;41:74–76.
37. O’Driscoll SW, Jaloszynski R, Morrey BF, et al. Origin of the medial ulnar collateral ligament. J Hand Surg (Am). 1992;17:164–168.
38. Nishida J, Shimamura T, Ehara S, et al. Posterior interosseous nerve palsy caused by parosteal lipoma of proximal radius. Skeletal Radiol. 1998;27:375–379.
39. Spinner RJ, Lins RE, Collins AJ, et al. Posterior interosseous nerve compression due to an enlarged bicipital bursa confirmed by MRI. J Hand Surg (Br). 1993;18:753–756.
40. el-Hadidi S, Burke FD. Posterior interosseous nerve syndrome caused by a bursa in the vicinity of the elbow. J Hand Surg (Br). 1987;12:23–24.
41. White SH, Goodfellow JW, Mowat A. Posterior interosseous nerve palsy in rheumatoid arthritis. J Bone Joint Surg Br. 1988;70:468–471.
42. Westkaemper JG, Varitimidis SE, Sotereanos DG. Posterior interosseous nerve palsy in a patient with rheumatoid synovitis of the elbow: a case report and review of the literature. J Hand Surg (Am). 1999;24:727–731.
43. Field JH. Posterior interosseous nerve palsy secondary to synovial chondromatosis of the elbow joint. J Hand Surg (Am). 1981;6:336–338.
44. Madhavan P, Leslie IJ. Intracapsular chondroma of the elbow producing a posterior interosseous nerve palsy. J Hand Surg (Br). 1998;23:107–108.
45. Busa R, Adani R, Marcuzzi A, et al. Acute posterior interosseous nerve palsy caused by a synovial haemangioma of the elbow joint. J Hand Surg (Br). 1995;20:652–654.
46. Yanagisawa H, Okada K, Sashi R. Posterior interosseous nerve palsy caused by synovial chondromatosis of the elbow joint. Clin Radiol. 2001;56:510–514.
47. Taniguchi Y, Yoshida M, Tamaki T. Posterior interosseous nerve syndrome due to pseudogout. J Hand Surg (Br). 1999;24:125–127.
48. Chen WS, Eng HL. Posterior interosseous neuropathy associated with tuberculous arthritis of the elbow joint: report of two cases. J Hand Surg (Am). 1994;19:611–613.
49. Lubahn JD, Cermak MB. Uncommon nerve compression syndromes of the upper extremity. J Am Acad Orthop Surg. 1998;6:378–386.
50. Lister GD, Belsole RB, Kleinert HE. The radial tunnel syndrome. J Hand Surg (Am). 1979;4:52–59.
51. Sponseller PD, Engber WD. Double-entrapment radial tunnel syndrome. J Hand Surg (Am). 1983;8:420–423.
52. Barnum M, Mastey RD, Weiss AP, et al. Radial tunnel syndrome. Hand Clin. 1996;12:679–689.
53. Roles NC, Maudsley RH. Radial tunnel syndrome: resistant tennis elbow as a nerve entrapment. J Bone Joint Surg Br. 1972;54:499–508.
54. Verhaar J, Spaans F. Radial tunnel syndrome. An investigation of compression neuropathy as a possible cause. J Bone Joint Surg Am. 1991;73:539–544.
55. Verhaar JA. Tennis elbow. Anatomical, epidemiological and therapeutic aspects. Int Orthop. 1994;18:263–267.
56. Werner CO. Lateral elbow pain and posterior interosseous nerve entrapment. Acta Orthop Scand Suppl. 1979;174:1–62.
57. Posner MA. Compressive neuropathies of the ulnar nerve at the elbow and wrist. Instr Course Lect. 2000;49:305–317.
58. Johnson RK, Spinner M, Shrewsbury MM. Median nerve entrapment syndrome in the proximal forearm. J Hand Surg (Am). 1979;4:48–51.
59. Hartz CR, Linscheid RL, Gramse RR, et al. The pronator teres syndrome: compressive neuropathy of the median nerve. J Bone Joint Surg Am. 1981;63:885–890.
60. Olehnik WK, Manske PR, Szerzinski J. Median nerve compression in the proximal forearm. J Hand Surg (Am). 1994;19:121–126.
61. Posner MA. Compressive ulnar neuropathies at the elbow: I. Etiology and diagnosis. J Am Acad Orthop Surg. 1998;6:282–288.
62. al-Naib I. Humeral supracondylar spur and Struthers’ ligament. A rare cause of neurovascular entrapment in the upper limb. Int Orthop. 1994;18:393–394.
63. Kessel L, Rang M. Supracondylar spur of the humerus. J Bone Joint Surg Br. 1966;48:765–769.
64. Lahey MD, Aulicino PL. Anomalous muscles associated with compression neuropathies. Orthop Rev. 1986;15:199–208.
65. Spinner M. Injuries to the Major Branches of Peripheral Nerves of the Forearm. Philadelphia, Pa: WB Saunders; 1978:234.
66. Boe S, Holst-Nielsen F. Intra-articular entrapment of the median nerve after dislocation of the elbow. J Hand Surg (Br). 1987;12:356–358.
67. Mody BS. A simple clinical test to differentiate rupture of flexor pollicis longus and incomplete anterior interosseous paralysis. J Hand Surg (Br). 1992;17:513–514.
68. Bredella MA, Tirman PF, Wischer TK, et al. Reactive synovitis of the knee joint: MR imaging appearance with arthroscopic correlation. Skeletal Radiol. 2000;29:577–582.
69. Adam G, Dammer M, Bohndorf K, et al. Rheumatoid arthritis of the knee: value of gadopentetate dimeglumine-enhanced MR imaging. AJR Am J Roentgenol. 1991;156:125–129.
70. Beltran J, Caudill JL, Herman LA, et al. Rheumatoid arthritis: MR imaging manifestations. Radiology. 1987;165:153–157.
71. Kursunoglu-Brahme S, Riccio T, Weisman MH, et al. Rheumatoid knee: role of gadopentetate-enhanced MR imaging. Radiology. 1990;176:831–835.
72. Singson RD, Zalduondo FM. Value of unenhanced spin-echo MR imaging in distinguishing between synovitis and effusion of the knee. AJR Am J Roentgenol. 1992;159:569–571.
73. Boegard T, Johansson A, Rudling O, et al. Gadolinium-DTPA-enhanced MR imaging in asymptomatic knees. Acta Radiol. 1996;37:877–882.
74. Konig H, Sieper J, Wolf KJ. Rheumatoid arthritis: evaluation of hypervascular and fibrous pannus with dynamic MR imaging enhanced with Gd-DTPA. Radiology. 1990;176:473–477.
75. Whitten CG, Moore TE, Yuh WT, et al. The use of intravenous gadopentetate dimeglumine in magnetic resonance imaging of synovial lesions. Skeletal Radiol. 1992;21:215–218.
76. Winalski CS, Aliabadi P, Wright RJ, et al. Enhancement of joint fluid with intravenously administered gadopentetate dimeglumine: technique, rationale, and implications. Radiology. 1993;187:179–185.
77. Ostergaard M, Lorenzen I, Henriksen O. Dynamic gadolinium-enhanced MR imaging in active and inactive immunoinflammatory gonarthritis. Acta Radiol. 1994;35:275–281.
78. Ostergaard M, Gideon P, Henriksen O, et al. Synovial volume—a marker of disease severity in rheumatoid arthritis? Quantification by MRI. Scand J Rheumatol. 1994;23:197–202.
79. Ostergaard M, Stoltenberg M, Henriksen O, et al. The accuracy of MRI-determined synovial membrane and joint effusion volumes in arthritis. A comparison of pre- and post-aspiration volumes. Scand J Rheumatol. 1995;24:305–311.
80. Ostergaard M. Different approaches to synovial membrane volume determination by magnetic resonance imaging: manual versus automated segmentation. Br J Rheumatol. 1997;36:1166–1177.
81. Bjorkengren AG, Geborek P, Rydholm U, et al. MR imaging of the knee in acute rheumatoid arthritis: synovial uptake of gadolinium-DOTA. AJR Am J Roentgenol. 1990;155:329–332.
82. Ostergaard M, Klarlund M. Importance of timing of post-contrast MRI in rheumatoid arthritis: what happens during the first 60 minutes after IV gadolinium-DTPA? Ann Rheum Dis. 2001;60:1050–1054.
83. Ostergaard M, Szkudlarek M. Magnetic resonance imaging of soft tissue changes in rheumatoid arthritis wrist joints. Semin Musculoskelet Radio l. 2001;5:257–274.
84. Ostergaard M, Gideon P, S rensen K, et al. Scoring of synovial membrane hypertrophy and bone erosions by MR imaging in clinically active and inactive rheumatoid arthritis of the wrist. Scand J Rheumatol. 1995;24:212–218.
85. Tamai K, Yamato M, Yamaguchi T, et al. Dynamic magnetic resonance imaging for the evaluation of synovitis in patients with rheumatoid arthritis. Arthritis Rheum. 1994;37:1151–1157.
86. Reiser MF, Bongartz GP, Erlemann R, et al. Gadolinium DTPA in rheumatoid arthritis and related diseases: first results with dynamic magnetic resonance imaging. Skeletal Radiol. 1989;18:591–597.
87. Clunie G, Hall-Craggs MA, Paley MN, et al. Measurement of synovial lining volume by magnetic resonance imaging of the knee in chronic synovitis. Ann Rheum Dis. 1997;56:526–534.
88. Eckhardt BP, Hernandez RJ. Pigmented villonodular synovitis: MR imaging in pediatric patients. Pediatr Radiol. 2004;34:943–947.
89. Fowler KA, Chung CB. Normal MR imaging anatomy of the elbow. Magn Reson Imaging Clin N Am. 2004;12:191–206.
90. Cotten A, Jacobson J, Brossmann J, et al. MR arthrography of the elbow: normal anatomy and diagnostic pitfalls. J Comput Assist Tomogr. 1997;21:516–522.
91. Clarke RP. Symptomatic, lateral synovial fringe (plica) of the elbow joint. Arthroscopy. 1988;4:112–116.
92. Duparc F, Putz R, Michot C, et al. The synovial fold of the humeroradial joint: anatomical and histological features, and clinical relevance in lateral epicondylalgia of the elbow. Surg Radiol Anat. 2002;24:302–307.
93. Awaya H, Schweitzer ME, Feng SA, et al. Elbow synovial fold syndrome: MR imaging findings. AJR Am J Roentgenol. 2001;177:1377–1381.
94. Skaf AY, Boutin RD, Dantas RW, et al. Bicipitoradial bursitis: MR imaging findings in eight patients and anatomic data from contrast material opacification of bursae followed by routine radiography and MR imaging in cadavers. Radiology. 1999;212:111–116.
95. Cimmino MA, Bountis C, Silvestri E, et al. An appraisal of magnetic resonance imaging of the wrist in rheumatoid arthritis. Semin Arthritis Rheum. 2000;30:180–195.
96. Ostergaard M, Hansen M, Stoltenberg M, et al. Quantitative assessment of the synovial membrane in the rheumatoid wrist: an easily obtained MRI score reflects the synovial volume. Br J Rheumatol. 1996;35:965–971.
97. Lee J, Lee SK, Suh JS, et al. Magnetic resonance imaging of the wrist in defining remission of rheumatoid arthritis. J Rheumatol. 1997;24:1303–1308.
98. Foley-Nolan D, Stack JP, Ryan M, et al. Magnetic resonance imaging in the assessment of rheumatoid arthritis—a comparison with plain film radiographs. Br J Rheumatol. 1991;30:101–106.
99. Tonolli-Serabian I, Poet JL, Dufour M, et al. Magnetic resonance imaging of the wrist in rheumatoid arthritis: comparison with other inflammatory joint diseases and control subjects. Clin Rheumatol. 1996;15:137–142.
100. McQueen FM, Stewart N, Crabbe J, et al. Magnetic resonance imaging of the wrist in early rheumatoid arthritis reveals a high prevalence of erosions at four months after symptom onset. Ann Rheum Dis. 1998;57:350–356.
101. Klarlund M, Ostergaard M, Gideon P, et al. Wrist and finger joint MR imaging in rheumatoid arthritis. Acta Radiol. 1999;40:400–409.
102. Schweitzer M, Morrison WB. Arthropathies and inflammatory conditions of the elbow. Magn Reson Imaging Clin N Am. 1997;5:603–617.
103. Imhof H, Nöbauer-Huhmann IM, Gahleitner A, et al. Pathophysiology and imaging in inflammatory and blastomatous synovial diseases. Skeletal Radiol. 2002;31:313–333.
104. Graif M, Schweitzer ME, Deely D, et al. The septic versus nonseptic inflamed joint: MRI characteristics. Skeletal Radiol. 1999;28:616–620.
105. Lund PJ, Chan KM, Unger EC, et al. Magnetic resonance imaging in coccidioidal arthritis. Skeletal Radiol. 1996;25:661–665.
106. Strouse PJ, Londy F, DiPietro MA, et al. MRI evaluation of infectious and non-infectious synovitis: preliminary studies in a rabbit model. Pediatr Radiol. 1999;29:367–371.
107. Erdman WA, Tamburro F, Jayson HT, et al. Osteomyelitis: characteristics and pitfalls of diagnosis with MR imaging. Radiology. 1991;180:533–539.
108. Brown JJ, vanSonnenberg E, Gerber KH, et al. Magnetic resonance relaxation times of percutaneously obtained normal and abnormal body fluids. Radiology. 1985;154:727–731.
109. Sawlani V, Chandra T, Mishra RN, et al. MRI features of tuberculosis of peripheral joints. Clin Radiol. 2003;58:755–762.
110. Jbara M, Patnana M, Kazmi F, et al. MR imaging: arthropathies and infectious conditions of the elbow, wrist, and hand. Magn Reson Imaging Clin N Am. 2004;12:361–379, vii.
111. Ho G Jr, Tice AD. Comparison of nonseptic and septic bursitis. Further observations on the treatment of septic bursitis. Arch Intern Med. 1979;139:1269–1273.
112. Floemer F, Morrison WB, Bongartz G, et al. MRI characteristics of olecranon bursitis. AJR Am J Roentgenol. 2004;183:29–34.
113. Stell IM. Septic and non-septic olecranon bursitis in the accident and emergency department—an approach to management. J Accid Emerg Med. 1996;13:351–353.
114. Karanjia ND, Stiles PJ. Cubital bursitis. J Bone Joint Surg Br. 1988;70:832–833.
115. Liessi G, Cesari S, Spaliviero B, et al. The US, CT and MR findings of cubital bursitis: a report of five cases. Skeletal Radiol. 1996;25:471–475.
116. Jacoby RK, Jayson MI, Cosh JA. Onset, early stages, and prognosis of rheumatoid arthritis: a clinical study of 100 patients with 11-year follow-up. BMJ. 1973;2:96–100.
117. Willems K, De Smet L. The Kudo total elbow arthroplasty in patients with rheumatoid arthritis. J Shoulder Elbow Surg. 2004;13:542–547.
118. Narváez JA, Narváez J, Roca Y, et al. MR imaging assessment of clinical problems in rheumatoid arthritis. Eur Radiol. 2002;12:1819–1828.
119. Savnik A, Malmskov H, Thomsen HS, et al. Magnetic resonance imaging of the wrist and finger joints in patients with inflammatory joint diseases. J Rheumatol. 2001;28:2193–2200.
120. Jevtic V, Watt I, Rozman B, et al. Distinctive radiological features of small hand joints in rheumatoid arthritis and seronegative spondyloarthritis demonstrated by contrast-enhanced (Gd-DTPA) magnetic resonance imaging. Skeletal Radiol. 1995;24:351–355.
121. McGonagle D, Gibbon W, Emery P. Classification of inflammatory arthritis by enthesitis. Lancet. 1998;352:1137–1140.
122. Scarpa R, Ames PR, della Valle G, et al. A rare enthesopathy in psoriatic oligoarthritis. Acta Derm Venereol Suppl (Stockh). 1994;186:74–75.
123. Smith DL, Campbell SM, Wernick R. ‘Tumoral’ enthesopathy: a juxtacortical osteosarcoma simulation. J Rheumatol. 1991;18:1631–1634.
124. Taniguchi Y, Yoshida M, Tamaki T. Cubital tunnel syndrome associated with calcium pyrophosphate dihydrate crystal deposition disease. J Hand Surg (Am). 1996;21:870–874.
125. Bencardino JT, Hassankhani A. Calcium pyrophosphate dihydrate crystal deposition disease. Semin Musculoskelet Radiol. 2003;7:175–185.
126. Steinbach LS. Calcium pyrophosphate dihydrate and calcium hydroxyapatite crystal deposition diseases: imaging perspectives. Radiol Clin North Am. 2004;42:185–205, vii.
127. Burke BJ, Escobedo EM, Wilson AJ, et al. Chondrocalcinosis mimicking a meniscal tear on MR imaging. AJR Am J Roentgenol. 1998;170:69–70.
128. Abreu M, Johnson K, Chung CB, et al. Calcification in calcium pyrophosphate dihydrate (CPPD) crystalline deposits in the knee: anatomic, radiographic, MR imaging, and histologic study in cadavers. Skeletal Radiol. 2004;33:392–398.
129. Hayes CW, Rosenthal DI, Plata MJ, et al. Calcific tendinitis in unusual sites associated with cortical bone erosion. AJR Am J Roentgenol. 1987;149:967–970.
130. Hayes CW, Conway WF. Calcium hydroxyapatite deposition disease. Radiographics. 1990;10:1031–1048.
131. Yang I, Hayes CW, Biermann JS. Calcific tendinitis of the gluteus medius tendon with bone marrow edema mimicking metastatic disease. Skeletal Radiol. 2002;31:359–361.
132. Yosipovitch G, Yosipovitch Z. Acute calcific periarthritis of the hand and elbows in women. A study and review of the literature. J Rheumatol. 1993;20:1533–1538.
133. Yu JS, Chung C, Recht M, et al. MR imaging of tophaceous gout. AJR Am J Roentgenol. 1997;168:523–527.
134. Ruiz ME, Erickson SJ, Carrera GF, et al. Monoarticular gout following trauma: MR appearance. J Comput Assist Tomogr. 1993;17:151–153.
135. Chung CB, Mohana-Borges A, Pathria M. Tophaceous gout in an amputation stump in a patient with chronic myelogenous leukemia. Skeletal Radiol. 2003;32:429–431.
136. Yulish BS, Lieberman JM, Strandjord SE, et al. Hemophilic arthropathy: assessment with MR imaging. Radiology. 1987;164:759–762.
137. Rand T, Trattnig S, Male C, et al. Magnetic resonance imaging in hemophilic children: value of gradient echo and contrast-enhanced imaging. Magn Reson Imaging. 1999;17:199–205.
138. Pagnoux C, Lhotellier L, Marek JJ, et al. Synovial cysts of the proximal tibiofibular joint: three case reports. Joint Bone Spine. 2002;69:331–333.
139. Ortega R, Fessell DP, Jacobson JA, et al. Sonography of ankle ganglia with pathologic correlation in 10 pediatric and adult patients. AJR Am J Roentgenol. 2002;178:1445–1449.
140. McCarthy CL, McNally EG. The MRI appearance of cystic lesions around the knee. Skeletal Radiol. 2004;33:187–209.
141. Ehara S. Communication of the ganglion with the joint and the tendon sheath. AJR Am J Roentgenol. 2003;180:541–542; author reply 542.
142. Andonopoulos AP, Meimaris N, Yiannopoulos G, et al. Large synovial cysts originating from the sternoclavicular joints in a patient with rheumatoid arthritis. Ann Rheum Dis. 2003;62:1119–1120.
143. Hill CL, Gale DR, Chaisson CE, et al. Periarticular lesions detected on magnetic resonance imaging: prevalence in knees with and without symptoms. Arthritis Rheum. 2003;48:2836–2844.
144. Niki Y, Matsumoto H, Otani T, et al. Gigantic popliteal synovial cyst caused by wear particles after total knee arthroplasty. J Arthroplasty. 2003;18:1071–1075.
145. Tschirch FT, Schmid MR, Pfirrmann CW, et al. Prevalence and size of meniscal cysts, ganglionic cysts, synovial cysts of the popliteal space, fluid-filled bursae, and other fluid collections in asymptomatic knees on MR imaging. AJR Am J Roentgenol. 2003;180:1431–1436.
146. Binkovitz LA, Berquist TH, McLeod RA. Masses of the hand and wrist: detection and characterization with MR imaging. AJR Am J Roentgenol. 1990;154:323–326.
147. Shankman S, Kolla S, Beltran J. MR imaging of tumors and tumor-like lesions of the upper extremity. Magn Reson Imaging Clin N Am. 2004;12:349–359.
148. Malghem J, Vande berg BC, Lebon C, et al. Ganglion cysts of the knee: articular communication revealed by delayed radiography and CT after arthrography. AJR Am J Roentgenol. 1998;170:1579–1583.
149. Burrafato V, Campanacci DA, Franchi A, et al. Synovial chondromatosis in a lumbar apophyseal joint. Skeletal Radiol. 1998;27:385–387.
150. Wittkop B, Davies AM, Mangham DC. Primary synovial chondromatosis and synovial chondrosarcoma: a pictorial review. Eur Radiol. 2002;12:2112–2119.
151. Kim SH, Hong SJ, Park JS, et al. Idiopathic synovial osteochondromatosis of the hip: radiographic and MR appearances in 15 patients. Korean J Radiol. 2002;3:254–259.
152. Tuckman G, Wirth CZ. Synovial osteochondromatosis of the shoulder: MR findings. J Comput Assist Tomogr. 1989;13:360–361.
153. Hughes TH, Sartoris DJ, Schweitzer ME, et al. Pigmented villonodular synovitis: MRI characteristics. Skeletal Radiol. 1995;24:7–12.
154. Masih S, Antebi A. Imaging of pigmented villonodular synovitis. Semin Musculoskelet Radiol. 2003;7:205–216.
155. Patkar D, Prasad S, Shah J, et al. Pigmented villonodular synovitis: magnetic resonance features of an unusual case of bilateral hip joint involvement. Australas Radiol. 2000;44: 458–459.
156. Ohnuma M, Sugita T, Kawamata T, et al. Pigmented villonodular synovitis of the knee with lesions of the bursae. Clin Orthop Relat Res. 2003;414:212–218.
157. Steinbach LS, Neumann CH, Stoller DW, et al. MRI of the knee in diffuse pigmented villonodular synovitis. Clin Imaging. 1989;13:305–316.
158. Huang GS, Lee CH, Chan WP, et al. Localized nodular synovitis of the knee: MR imaging appearance and clinical correlates in 21 patients. AJR Am J Roentgenol. 2003;181:539–543.
159. Stacy GS, Heck RK, Peabody TD, et al. Neoplastic and tumor-like lesions detected on MR imaging of the knee in patients with suspected internal derangement: Part 2, articular and juxtaarticular entities. AJR Am J Roentgenol., 2002;178:595–599.
160. Sheldon PJ, Forrester DM. Imaging of amyloid arthropathy. Semin Musculoskelet Radiol. 2003;7:195–203.
161. Escobedo EM, Hunter JC, Zink-Brody GC, et al. Magnetic resonance imaging of dialysis-related amyloidosis of the shoulder and hip. Skeletal Radiol. 1996;25:41–48.
162. Amoroso E, Vitale C, Silvestro A. Spinal-cord compression due to extradural amyloidosis of the cervico-occipital hinge, in a hemodialysed patient. A case report. J Neurosurg Sci. 2001;45:120–124.
163. Berquist TH, Ehman RL, King BF, et al. Value of MR imaging in differentiating benign from malignant soft-tissue masses: study of 95 lesions. AJR Am J Roentgenol. 1990;155:1251–1255.
164. Crim JR, Seeger LL, Yao L, et al. Diagnosis of soft-tissue masses with MR imaging: can benign masses be differentiated from malignant ones? Radiology. 1992;185:581–586.
165. Kransdorf MJ, Murphey MD. Imaging of Soft Tissue Tumors. Philadelphia, Pa: WB Saunders; 1997:429.
166. Sundaram M, McLeod RA. MR imaging of tumor and tumor-like lesions of bone and soft tissue. AJR Am J Roentgenol. 1990;155:817–824.
167. Allen PW, Enzinger FM. Hemangioma of skeletal muscle. An analysis of 89 cases. Cancer. 1972;29:8–22.
168. Cohen JM, Weinreb JC, Redman HC. Arteriovenous malformations of the extremities: MR imaging. Radiology. 1986;158:475–479.
169. Hawnaur JM, Whitehouse RW, Jenkins JP, et al. Musculoskeletal haemangiomas: comparison of MRI with CT. Skeletal Radiol. 1990;19:251–258.
170. Murphey MD, Fairbairn KJ, Parman LM, et al. From the archives of the AFIP. Musculoskeletal angiomatous lesions: radiologic–pathologic correlation. Radiographics. 1995;15: 893–917.
171. Kransdorf MJ. Benign soft-tissue tumors in a large referral population: distribution of specific diagnoses by age, sex, and location. AJR Am J Roentgenol. 1995;164:395–402.
172. Kransdorf MJ. Malignant soft-tissue tumors in a large referral population: distribution of diagnoses by age, sex, and location. AJR Am J Roentgenol. 1995;164:129–134.
173. Stout A. The peripheral manifestations of the specific nerve sheath tumor (neurilemmoma). Am J Cancer. 1935;24:751–796.
174. Suh JS, Abenoza P, Galloway HR, et al. Peripheral (extracranial) nerve tumors: correlation of MR imaging and histologic findings. Radiology. 1992;183:341–346.
175. Cerofolini E, Landi A, DeSantis G, et al. MR of benign peripheral nerve sheath tumors. J Comput Assist Tomogr. 1991;15:593–597.
176. Cohen LM, Schwartz AM, Rockoff SD. Benign schwannomas: pathologic basis for CT inhomogeneities. AJR Am J Roentgenol. 1986;147:141–143.
177. Burk DL Jr, Brunberg JA, Kanal E, et al. Spinal and paraspinal neurofibromatosis: surface coil MR imaging at 1.5 T1. Radiology. 1987;162:797–801.
178. Mann FA, Murphy WA, Totty WG, et al. Magnetic resonance imaging of peripheral nerve sheath tumors. Assessment by numerical visual fuzzy cluster analysis. Invest Radiol. 1990;25:1238–1245.
179. Stull MA, Moser RP Jr, Kransdorf MJ, et al. Magnetic resonance appearance of peripheral nerve sheath tumors. Skeletal Radiol. 1991;20:9–14.
180. Holt PD, de Lang EE. Cat scratch disease: magnetic resonance imaging findings. Skeletal Radiol. 1995;24:437–440.
181. Hopkins KL, Simoneaux SF, Patrick LE, et al. Imaging manifestations of cat-scratch disease. AJR Am J Roentgenol. 1996;166:435–438.
182. Gregory DW, Decker MD. Cat scratch disease: an infection beyond the lymph node. Am J Med Sci. 1986;292:389–390.
183. Gianott F. A case of benign lymphoreticulosis (cat-scratch disease) with unusual herpetiform vesiculopustular eruption at the inoculation site [in Italian]. G Ital Dermatol. 1963;104:379–386.
184. Windsor JJ. Cat-scratch disease: epidemiology, aetiology and treatment. Br J Biomed Sci. 2001;58:101–110.
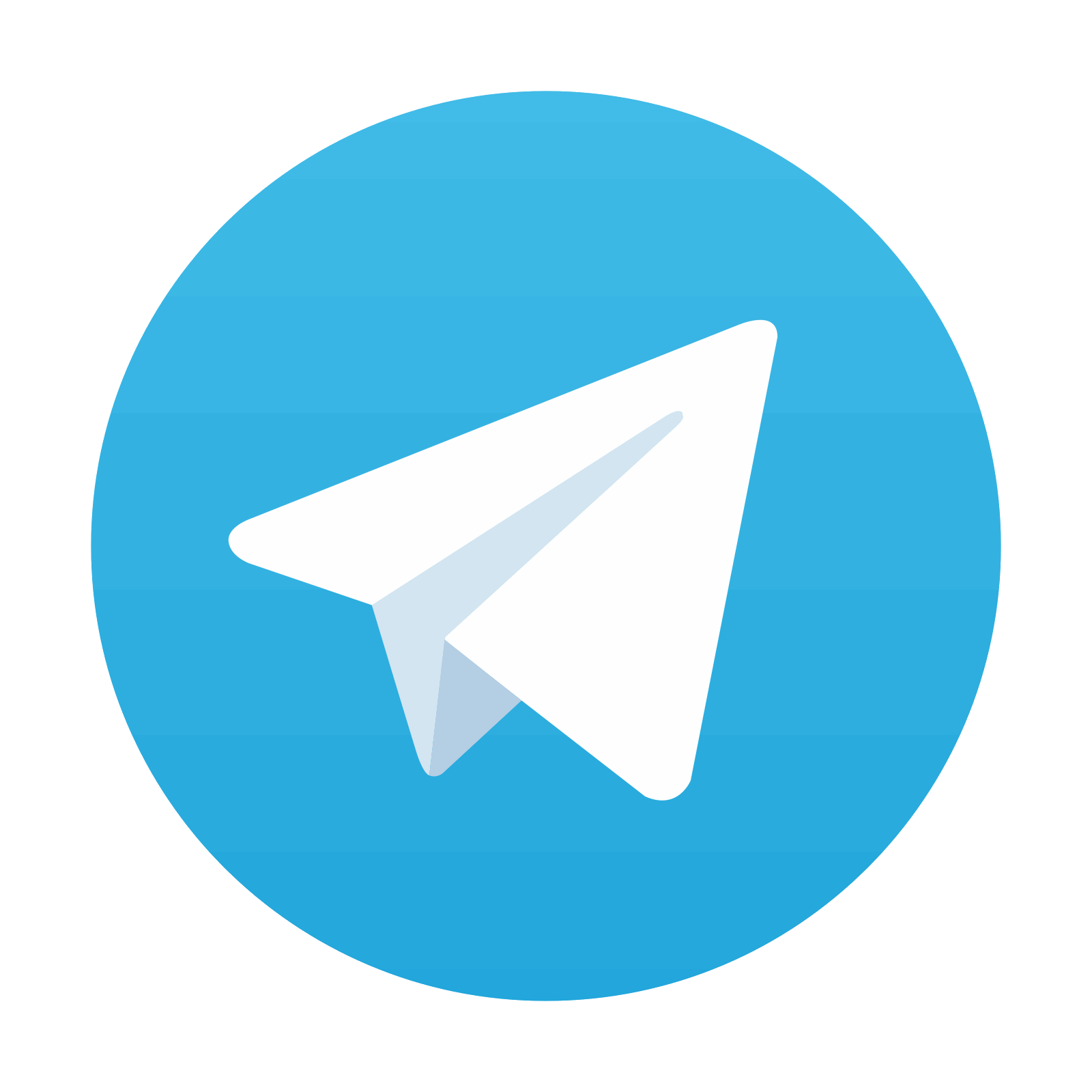
Stay updated, free articles. Join our Telegram channel

Full access? Get Clinical Tree
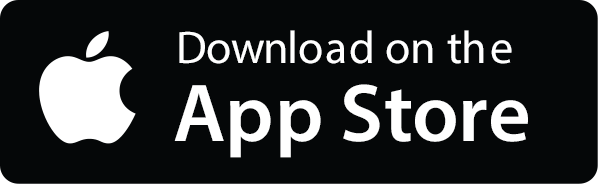
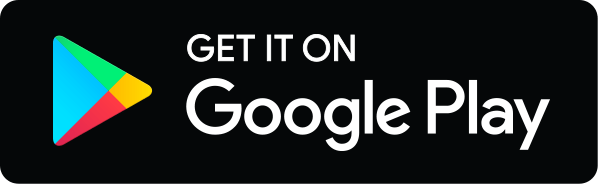