Interventions
Dose (mg)
Active comparator
Follow-upmonths
∆LDL-C(%)
∆HDL-C(%)
Target vessels
Referencenumber
Simvastatin
5–20
Diet management
3
−29.5
+14.9
Aorta, carotid arteries
[19]
Atorvastatin
20
Atorvastatin 5 mg
6
−38.5
+13.5
Aorta, femoral arteries
[94]
Atorvastatin
40
None
3
+11.1
0
Aorta, iliofemoral arteries
[95]
Atorvastatin
80
Atorvastatin 10 mg
12
−38.4
−0.4
Aorta, carotid arteries
[22]
Fluvastatin
20
None
3
−39.8
+36.0
Aorta, carotid arteries
[99]
Pitavastatin
4
None
3
+5.9
+46.4
Coronary artery
[110]
Pioglitazone
15–30
Glimepiride 0.5–4 mg
4
−1.8
+11.3
Aorta, carotid arteries
[100]
Pioglitazone
15–30
Glimepiride 0.5–4 mg
4
−1.8
+9.4
Coronary artery
[112]
Dalcetrapib
600
Placebo
12
+3.8
+33.9
Aorta, carotid arteries
[21]
Lifestylemodification
Dietary counseling,physical exercise
None
17
−6.5
+13.5
Aorta, carotid, subclavian,and iliac arteries
[93]
The first randomized clinical study has been reported that vascular FDG uptake was attenuated by simvastatin or dietary modification for 3 months [19]. FDG activity in the carotid arteries and thoracic aorta was validated using merged FDG-PET and CT images (Fig. 16.1). The 3-month follow-up scan revealed the significant resolution of FDG activity within plaques in the simvastatin group, but not in the diet group (Fig. 16.2). Although simvastatin was found to reduce LDL-C levels by approximately 30 %, the extent of the reduction in FDG uptake within atherosclerotic plaques did not correlate with that of LDL-C levels (Fig. 16.3, upper right). These findings suggested that simvastatin could attenuate vascular inflammation in a LDL-C independent manner. The extent of the reduction in FDG activity in these plaques was positively correlated with that of the approximately 15 % increase in HDL-C levels (Fig. 16.3, lower right) and led us to speculate about the anti-inflammatory properties of HDL-C and/or simvastatin [19]. Alternatively, lifestyle modifications have been shown to induce favorable effects on blood pressure and lipid profiles, thereby decreasing arterial FDG activity over 17 months in response to atherogenic risk reductions [93]. This study also demonstrated that the extent of reductions in arterial FDG activity correlated with the approximately 15 % increase in HDL-C levels [93]. Rosuvastatin was also found to decrease the volume of atheroma in association with the approximately 15 % increase in HDL-C levels in an IVUS study [96], which further suggested that the anti-inflammatory effects of statins may be partly mediated by elevations in HDL-C levels.
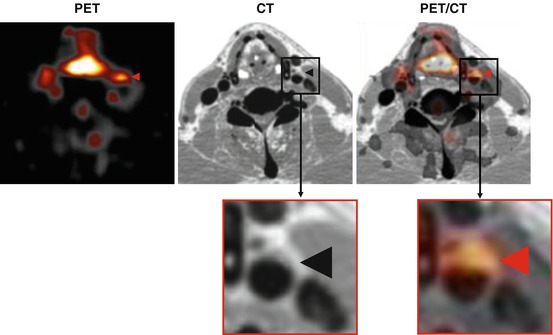
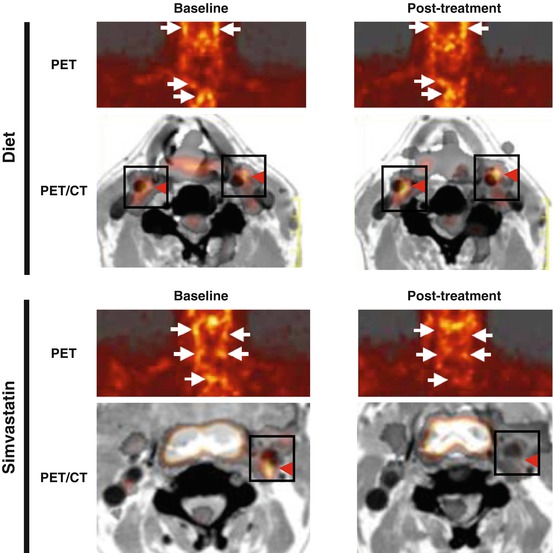
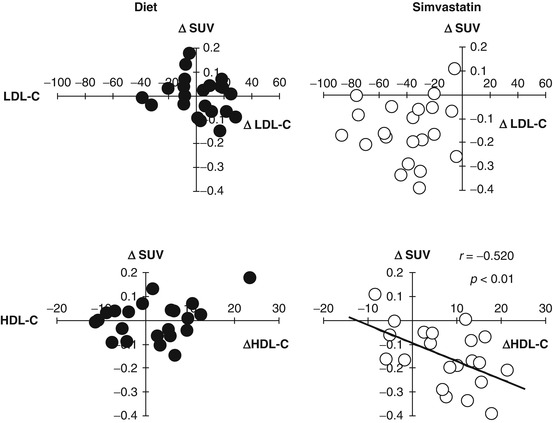
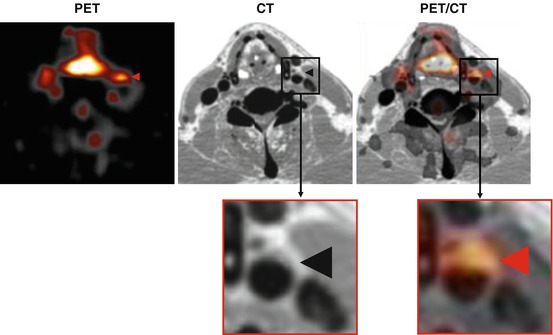
Fig. 16.1
Representative transaxial images of FDG-PET (left), enhanced CT (middle), and the co-registration of PET and CT (PET/CT) (right) showing FDG uptake in the right carotid plaque (arrowheads). FDG 18F-fluorodeoxyglucose, PET positron-emission tomography, CT computerized tomography
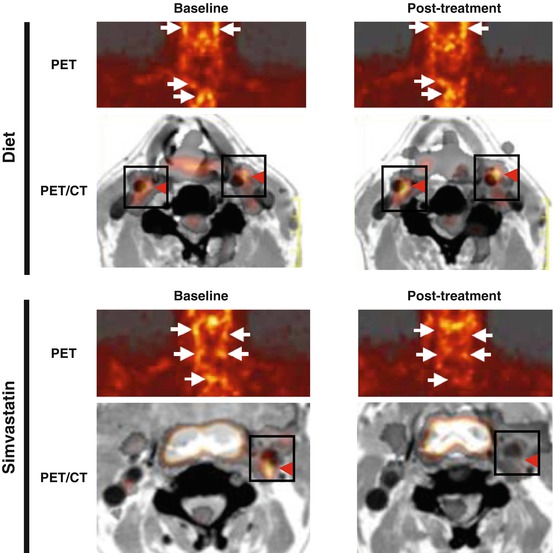
Fig. 16.2
FDG-PET images at baseline (left) and after 3 months of an intervention (right) with a dietary modification (top) or simvastatin therapy (bottom). The dietary modification alone had no effect on FDG uptake (top, arrows) in the carotid arteries and aortic arch. On the other hand, the uptake of FDG was markedly reduced by the simvastatin treatment (bottom, arrows). Serial co-registered PET and CT images allowed the identification of FDG localization in the carotid arteries (red arrowheads) (Reproduced, with permission, from Rudd and Tahara et al. [20]). FDG 18F-fluorodeoxyglucose, PET positron-emission tomography, CT computerized tomography
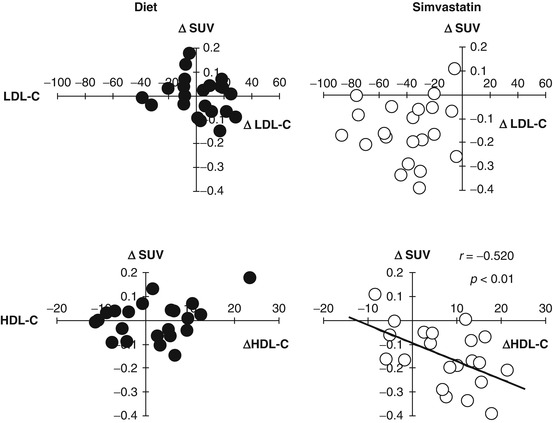
Fig. 16.3
Correlations between changes in plaque FDG activity and alterations in HDL cholesterol (∆HDL-C) and LDL cholesterol (∆LDL-C) after a 3-month treatment with dietary management alone (diet) or simvastatin. ∆SUV as FDG activity within plaques only correlated with ∆HDL-C in the statin group (Reproduced, with permission, from Tahara et al. [19]). HDL-C high-density lipoprotein cholesterol, LDL-C low-density lipoprotein cholesterol, SUV standardized uptake value
Statins have been shown to reduce the risk of cardiovascular morbidity and mortality in both primary and secondary prevention studies in proportion to reductions in LDL-C levels and the circulating markers of inflammation [97]. Furthermore, the intensification of statin therapy has also reduced the incidence of major cardiovascular events [98]. However, it remains unknown whether high-dose statin treatment could result in greater reductions in atherosclerotic inflammation than low-dose statins. Several very recent studies using PET/CT imaging demonstrated that atorvastatin produced significant dose-dependent reductions in vascular FDG activity [22, 94, 95]. In accordance with the findings of previous studies, changes in vascular FDG activity did not correlate with changes in LDL-C. Fluvastatin also attenuated the activity of FDG in the carotid arteries and thoracic aorta [99] (Fig. 16.4). Compared to atorvastatin, these favorable effects appear not to be class specific. These findings suggest that serial FDG-PET images can be used to monitor improvements in the inflammatory component of atherosclerotic lesions in response to therapeutic interventions.
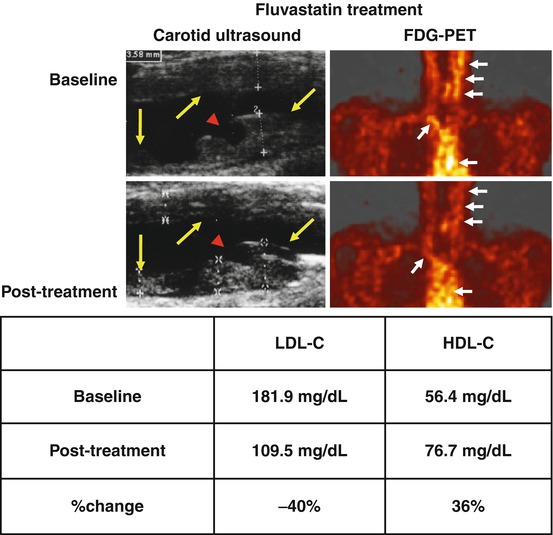
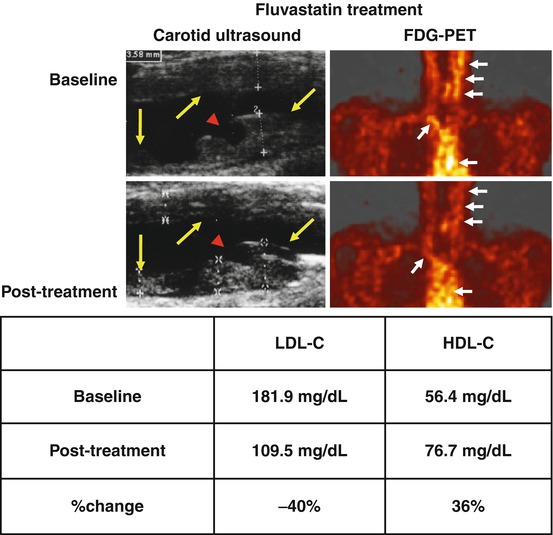
Fig. 16.4
Carotid artery ultrasound imaging revealed carotid atherosclerosis in a patient with a recent history of acute cardiovascular events. The plaque had a homogeneous ultrasonic appearance with an irregular surface at baseline. A ruptured plaque showing discontinuity of the surface on the left carotid artery was detected (arrowhead). The patient received 20 mg fluvastatin, and a marked resolution of abnormal FDG uptake was observed after the treatment for 3 months (post treatment) with a concomitant reduction in LDL-C levels and elevation in HDL-C levels. Yellow arrows denote carotid plaques, and the red arrowhead denotes atherosclerotic plaque ulceration. White arrows indicate vascular FDG uptake (Reproduced and modified, with permission, from Tahara et al. [99]). FDG 18F-fluorodeoxyglucose, PET positron-emission tomography, CT computerized tomography
PET is a promising modality for the future development of new therapeutic strategies including molecular targeted therapies and remains one of the most important tools for probing human disease processes. A previous study using FDG-PET imaging of the carotid arteries revealed that vascular inflammation was clearly associated with metabolic syndrome [54]; vascular FDG activity was independently associated with waist circumference, hypertension medication, carotid IMT, insulin resistance, CRP, and HDL-C. Furthermore, age- and gender-adjusted vascular FDG activity increased in proportion to the number of conditions associated with metabolic syndrome [54].
Pioglitazone has been shown to exert other beneficial metabolic effects including not only improvements in insulin resistance, which has been implicated in metabolic syndrome, but also lipid profiles, such as increased HDL-C levels [83–88]. FDG-PET/contrasted CT has been used to evaluate the effects of pioglitazone on plaque inflammation [100]. A recent study reported that FDG activity in the carotid arteries and ascending aortas of the aortic arch in patients with impaired glucose tolerance or type 2 diabetes was significantly lower following a 4-month treatment with pioglitazone than with glimepiride in a glucose-lowering independent manner, whose changes were inversely associated with those in plasma HDL-C levels (Fig. 16.5) [100]. It is also well known that not only high levels of LDL-C but also low levels of HDL-C are significant predictors of atherosclerotic CVD [101]. These studies have provided a valuable insight into how patients with low levels of HDL-C become vulnerable to cardiovascular events at the vascular level [19, 93, 100].
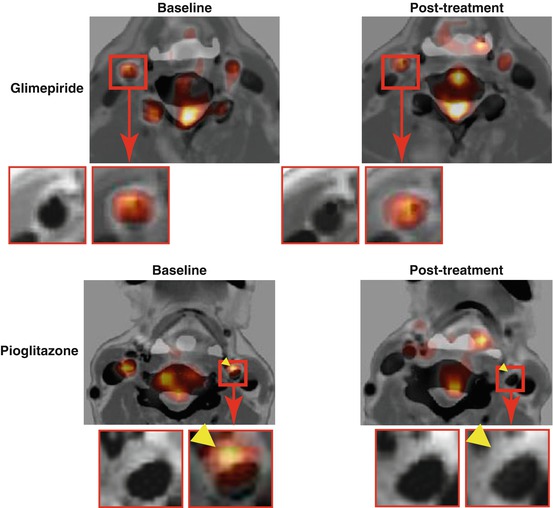
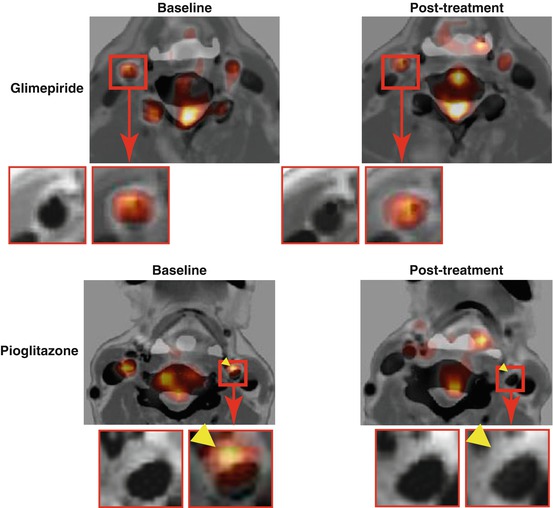
Fig. 16.5
Treatment effects of pioglitazone and glimepiride on FDG uptake in atherosclerotic plaques. Representative FDG-PET/CT with contrast media images (left) at baseline and (right) after a 4-month treatment with (bottom) pioglitazone or (top) glimepiride. A reduction was observed in the uptake of FDG by the atherosclerotic plaque with the pioglitazone treatment (arrowheads) (Reproduced, with permission, from Mizoguchi and Tahara et al. [100]). FDG 18F-fluorodeoxyglucose, PET positron-emission tomography, CT computerized tomography
16.8 New Drugs to Increase HDL-C Levels
Cholesteryl ester transfer protein (CETP) mediates the transfer of cholesteryl esters from HDL to other lipoproteins (e.g., LDL) and also the transfer of triglycerides to HDL. The inhibition of CETP has been shown to increase HDL-C levels and decrease LDL-C cholesterol levels [102]. Accordingly, the increase in HDL-C levels induced by the inhibition of CETP may represent an attractive approach for reducing vascular inflammation.
The first class CETP inhibitor, torcetrapib, did not cause atherosclerotic plaque regression, as established by both IVUS-derived percent atheroma volume [103] and carotid IMT [104], in spite of its potent effects including a more than 15 % relative decrease in LDL-C levels and a more than 50 % relative increase in HDL-C levels. However, the clinical development of torcetrapib was discontinued when the Investigation of Lipid Level Management to Understand its Impact in Atherosclerotic Events (ILLUMINATE) trial found that torcetrapib was associated with an increased risk of CVD events and all-cause death [105].
The second class CETP inhibitor, dalcetrapib, increased HDL-C levels by approximately 30 % without affecting LDL-C or triglyceride levels, and this was associated with a reduction in carotid arterial and aortic wall inflammation, as evaluated by FDG-PET/CT, over 6 months relative to that with the placebo (dal-PLAQUE) [21]. No significant differences were observed in blood pressure and the frequency of adverse events between dalcetrapib and the placebo. These findings indicated that dalcetrapib and/or HDL-C increases could have therapeutic effects on inflammatory activity in aortic wall vessels. However, a study on the effects of dalcetrapib on atherosclerotic disease in patients with coronary artery disease (dal-OUTCOMES) was terminated after an interim analysis of the trial revealed that dalcetrapib did not significantly reduce CVD events [106], which was similar to the findings of the ILLUMINATE trial [105].
The third class CETP inhibitor, anacetrapib, decreased LDL-C levels by 36 % and increased HDL-C levels by 38 % without increasing blood pressure [107]. Therefore, more potent CETP inhibitors may be able to improve the outcomes of patients with ACS. The REVEAL (randomized evaluation of the effects of anacetrapib through lipid modification, a large-scale, randomized placebo-controlled trial of the clinical effects of anacetrapib among people with established vascular disease) cardiovascular outcome study is currently ongoing to determine whether anacetrapib can reduce the incidence of major coronary events in statin-treated patients with a history of CVD [108].
HDL-C is believed to protect against atherosclerosis by promoting reverse cholesterol transport and anti-inflammatory effects. These multiple mechanisms of action make HDL-C a complex therapeutic target with potential for the treatment of patients with atherosclerosis. Not only an increase in the plasma levels of HDL-C but also improvements in HDL function are new therapeutic strategies currently being developed.
16.9 Measuring FDG Activity in the Coronary Artery
Measuring FDG activity in coronary arteries is limited by uptake into the myocardium; however, this can be reduced by a long fast and/or a low-carbohydrate and high-fat diet prior to an FDG scan. In a recent retrospective study, these preparations were found to adequately suppress the myocardial uptake of FDG in two thirds of patients [109]. FDG activity in the coronary arteries and aortic arch has been associated with the presence of angiographically proven coronary artery disease [109]. Cardiac or respiratory gating could greatly impact on spatial and temporal resolution for coronary artery FDG activity.
There is a recent report of a patient with Q-wave MI who had a right coronary artery aneurysm with a contrast-enhanced effect and focal FDG uptake in the aneurysm, as demonstrated by CT angiography and FDG-PET (Fig. 16.6, top) [110]. After a 3-month treatment of pitavastatin, an attenuation in the intense contrast effect and almost complete resolution of FDG uptake in the aneurysm were observed with a concomitant reduction in serum inflammatory markers and elevation in HDL-C levels (Fig. 16.6, bottom) [110]. These findings are encouraging and indicate that prospective and serial FDG-PET imaging, even for coronary artery inflammation, may become possible in the future.
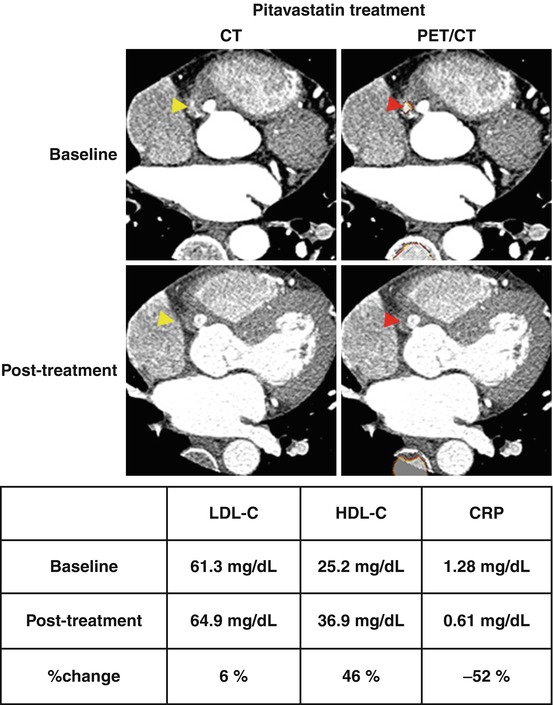
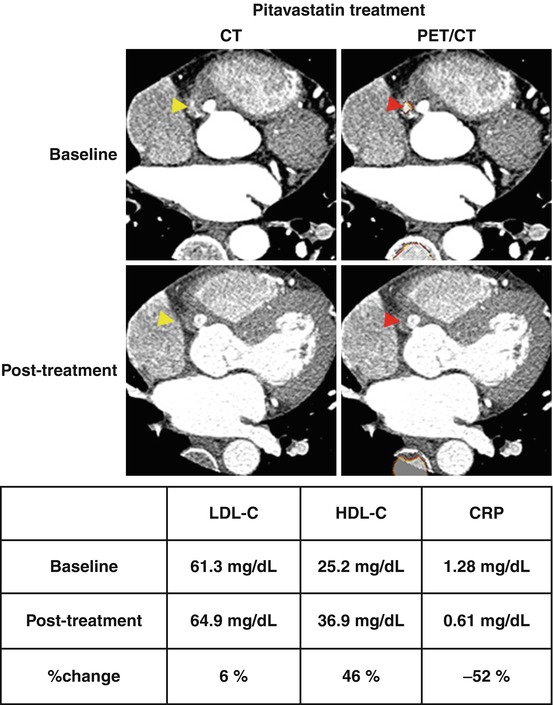
Fig. 16.6
Multislice CT angiography revealed an RCA aneurysm and intense contrast effect in the aneurysm, which may have been indicative of inflammation (left above, yellow arrowhead). FDG-PET and co-registered CT scans revealed focal FDG uptake at the contrast-intense site in the RCA aneurysm (left below, red arrowhead). A 3-month treatment with 4 mg pitavastatin resolved the contrast intensity in the RCA aneurysm in follow-up CT angiography (right above, yellow arrowhead) and completely resolved abnormal FDG uptake in FDG-PET imaging (right below, red arrowhead) with a concomitant reduction in CRP levels and elevation in HDL-C levels (Reproduced and modified, with permission, from Tahara et al. [110]). RCA right coronary artery, CRP C-reactive protein, PET positron-emission tomography, CT computerized tomography, LDL-C low-density lipoprotein cholesterol
A recent prospective study demonstrated that FDG activity in the left main coronary artery was higher in patients with recent ACS than in those with stable disease [111]. Pioglitazone was very recently shown to attenuate FDG activity in the left main coronary artery in patients with impaired glucose tolerance or diabetes in a glucose-lowering independent manner, which suggests that pioglitazone may protect against cardiac events by suppressing coronary artery inflammation (Fig. 16.7) [112].
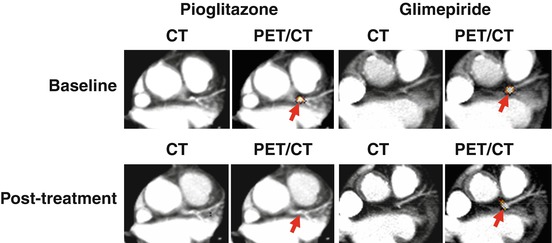
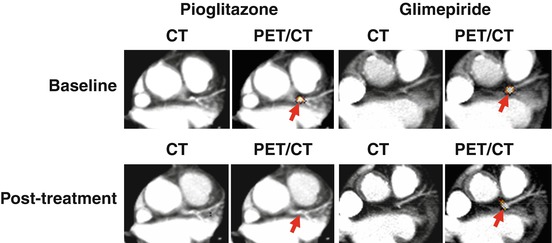
Fig. 16.7
Representative PET/CT fused images revealed the effects of pioglitazone and glimepiride on FDG uptake in the LM. A reduction was observed in FDG uptake in the LM with pioglitazone, but not with glimepiride (arrows) (Reproduced, with permission, from Nitta and Tahara et al. [112]). LM left main coronary artery, PET positron-emission tomography, CT computerized tomography
16.10 Concluding Remarks
The assessment of atherosclerotic plaques by imaging modalities is essential for the identification of atherosclerotic plaques in humans. Various invasive and noninvasive imaging modalities are currently available. Far from merely detecting stenosis, conventional imaging modalities can characterize plaque size and plaque composition, and most are employed as end points in therapeutic trials. The ultimate goal is to detect high-risk plaques that are vulnerable to rupture and thrombosis. The use of molecular imaging to provide functional and cellular information may enhance the risk stratification of atherosclerotic CVD beyond anatomical imaging. The area of molecular imaging has been rapidly advancing in recent years. The ability of noninvasive molecular imaging modalities such as FDG-PET to provide early insights into the efficacy of therapeutic interventions is more attractive than invasive morphological observations. Further studies will greatly facilitate the process of drug development and translate next-generation therapeutics into clinical medicine.
References
1.
Virmani R, Kolodgie FD, Burke AP, Farb A, Schwartz SM. Lessons from sudden coronary death: a comprehensive morphological classification scheme for atherosclerotic lesions. Arterioscler Thromb Vasc Biol. 2000;20(5):1262–75.PubMed
4.
Spagnoli LG, Bonanno E, Sangiorgi G, Mauriello A. Role of inflammation in atherosclerosis. J Nucl Med. 2007;48(11):1800–15.PubMed
5.
Tahara N, Imaizumi T, Virmani R, Narula J. Clinical feasibility of molecular imaging of plaque inflammation in atherosclerosis. J Nucl Med. 2009;50(3):331–4.PubMed
6.
Libby P. Inflammation in atherosclerosis. Arterioscler Thromb Vasc Biol. 2012;32(9):2045–51.PubMedCentralPubMed
7.
Libby P, Ridker PM, Hansson GK. Progress and challenges in translating the biology of atherosclerosis. Nature. 2011;473(7347):317–25.PubMed
8.
Rodriguez-Granillo GA, García-García HM, Mc Fadden EP, Valgimigli M, Aoki J, de Feyter P, Serruys PW. In vivo intravascular ultrasound-derived thin-cap fibroatheroma detection using ultrasound radiofrequency data analysis. J Am Coll Cardiol. 2005;46(11):2038–42.PubMed
9.
Valgimigli M, Rodriguez-Granillo GA, Garcia-Garcia HM, Malagutti P, Regar E, de Jaegere P, de Feyter P, Serruys PW. Distance from the ostium as an independent determinant of coronary plaque composition in vivo: an intravascular ultrasound study based radiofrequency data analysis in humans. Eur Heart J. 2006;27(6):655–63.PubMed
10.
Kubo T, Imanishi T, Takarada S, Kuroi A, Ueno S, Yamano T, Tanimoto T, Matsuo Y, Masho T, Kitabata H, Tsuda K, Tomobuchi Y, Akasaka T. Assessment of culprit lesion morphology in acute myocardial infarction: ability of optical coherence tomography compared with intravascular ultrasound and coronary angioscopy. J Am Coll Cardiol. 2007;50(10):933–9.PubMed
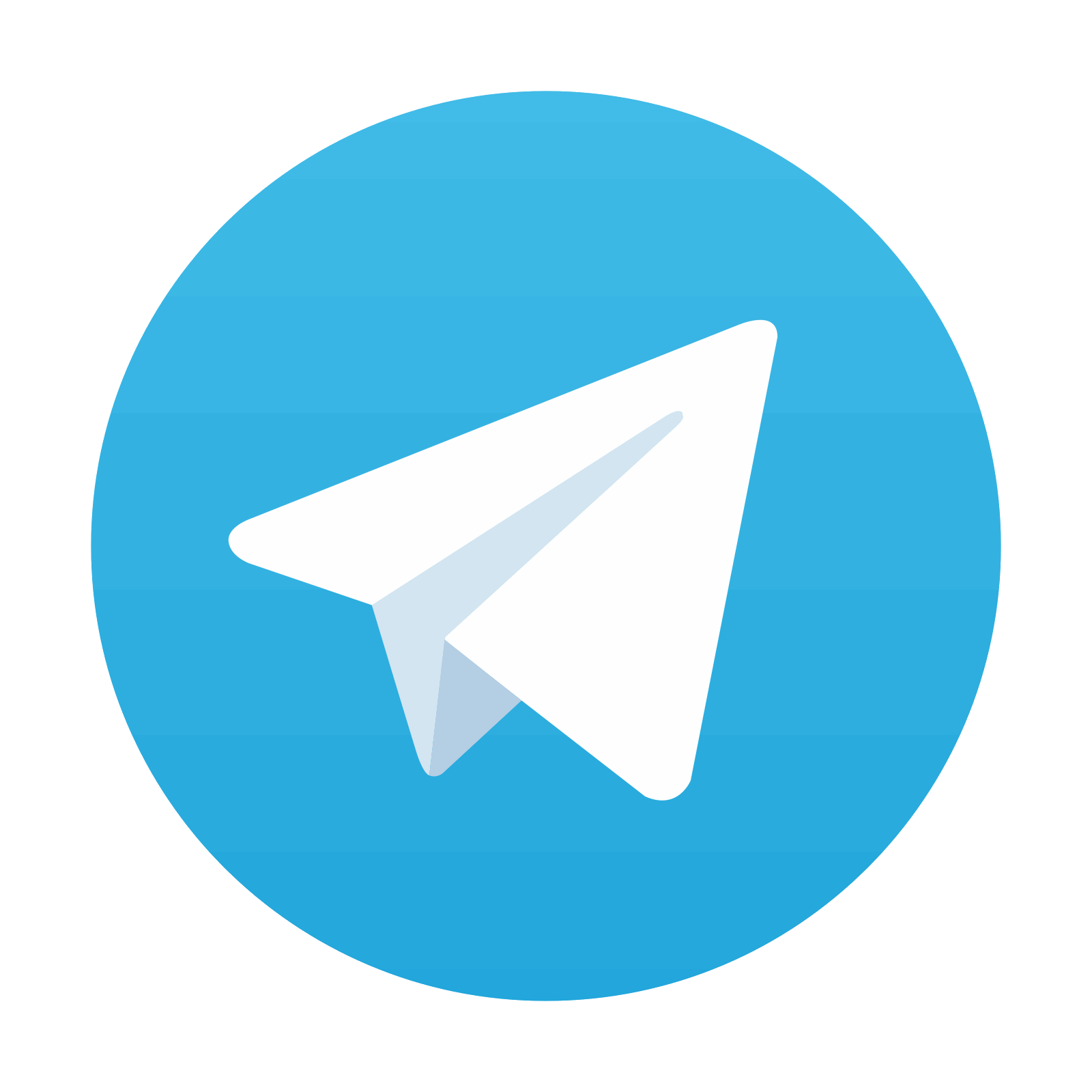
Stay updated, free articles. Join our Telegram channel

Full access? Get Clinical Tree
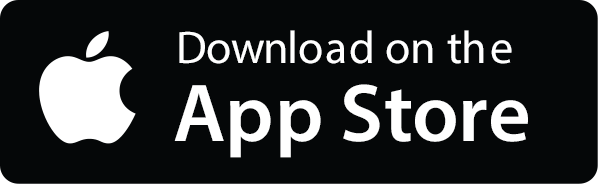
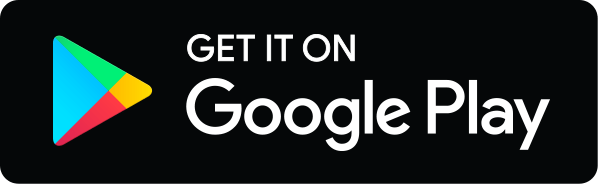