Before the development of mammography, the detection of abnormalities in the breast was largely based on visual inspection and palpation. This has dramatically changed in the last half of the 20th century. The successive development of mammography, ultrasound, and breast magnetic resonance imaging (MRI) has made imaging of the breast indispensable. Moreover, the development of image-guided needle biopsy has virtually obviated the need for surgery of benign breast lesions.
Imaging Techniques and Image-Guided Biopsy
Mammography
Mammography is an x-ray-based technique that makes use of the different absorption rates of fatty and fibroglandular tissue (FGT) in the breast. Because the breast consists only of soft tissues, a low-energy spectrum is used. Typical settings for mammography are between 25 and 35 kV. Whereas original mammography consisted of little more than an x-ray tube placed against the breast with a film on the opposite side, currently mammography is performed with dedicated mammography machines.
In addition to the x-ray tube and detector, these machines also include a compression mechanism that is used to flatten the breast on the detector plate. This leads to a better spread of the FGT and thus better interpretability of the resulting image. Furthermore, it also lowers the dose needed for high-quality images, as that is mainly dependent on the breast thickness. Besides, it prevents motion artifacts that would otherwise obscure the fine details in the image.
A standard mammogram consists of two views per breast. The standard views are mediolateral oblique (MLO) and craniocaudal (CC). As the glandular structures in the breast have a teardrop-like configuration with the tail in the direction of the axilla, the MLO view is usually perpendicular to this and therefore shows most of the breast parenchyma as well as part of the pectoral muscle. The CC view depicts part of the medial breast that is inaccessible in the MLO view and also enables a somewhat higher compression, as it does not include muscles, which is beneficial to discriminate small masses from FGT. Strict quality control is usually employed for these acquisitions. Images are considered adequate when the whole fibroglandular disc is depicted, the nipple shows in profile, and no folds are present. In MLO acquisitions, the pectoral muscle should be visible from the level of the nipple; in CC acquisitions, the pectoral muscle should just be visible on the dorsal side of the breast, although particularly the latter often shows infeasible.
In case the standard views do not sufficiently show the breast tissue, several additional views are commonly performed. These include nipple views to show the retroareolar area, the axillary view (Cleopatra view) that better depicts the axillary tail, and the cleavage view that shows the medial part of both breasts. In women with breast implants, an Eklund view is sometimes performed, in which the prosthesis is pushed to the back and only the FGT of the breast is compressed.
Mammography requires a very high spatial resolution to accurately depict the structures in the breast. Typical voxel sizes are below 100 × 100 microns. Although tumors detected with mammography are on average about 15 to 20 mm in size, the high resolution enables accurate assessment of the lesion margins. Moreover, the high resolution enables the depiction of calcifications in the breast that may be associated with breast cancer. These calcifications commonly have a size of less than 200 microns and are therefore only visible when such high spatial resolutions are achieved.
In the second decade of the 21st century, digital breast tomosynthesis (DBT) emerged as the better mammogram. In DBT, the same machinery is used but the x-ray tube is moved over the breast in a limited angular range (15–50 degrees, depending on the machine used). During this movement, multiple acquisitions are obtained, which are subsequently reconstructed to provide a pseudo-3D stack of images that provides some depth information of the imaged breast. This sometimes enables visualization of lesions that would otherwise be hidden in the normal FGT, and conversely may enable discarding potential lesions that are caused by the projection of overlapping fibroglandular structures. Consequently, it was reported that sensitivity for cancer increases; specificity also increases, albeit especially the latter is strongly dependent on the threshold used for mammographic recall. It is possible to create a synthetic mammogram from the tomographic projections that, when used in conjunction with the tomosynthesis stack, obviates the creation of a regular mammography, which limits the radiation dose applied.
When evaluating a mammogram or DBT, the first objective is to assess whether the obtained views meet the quality criteria for each view. Subsequently, the normal breast configuration is assessed. This includes an assessment of the relative amount of FGT in the breast, which is commonly referred to as density. Density is typically described according to the Breast Imaging Reporting and Data System (BI-RADS) that discerns these four categories: (1) almost entirely fatty tissue, (2) scattered FGT, (3) heterogeneous FGT, and (4) extremely dense FGT ( Fig. 2.1 ). However, there are currently several commercial applications available that automatically determine density from a mammogram and have been shown to be more stable than human assessment. With increasing density, the sensitivity of mammography for breast cancer decreases, as tumors may be masked by overprojecting FGT, and this effect is still present when using DBT. In addition, the risk of developing breast cancer increases with increasing density.
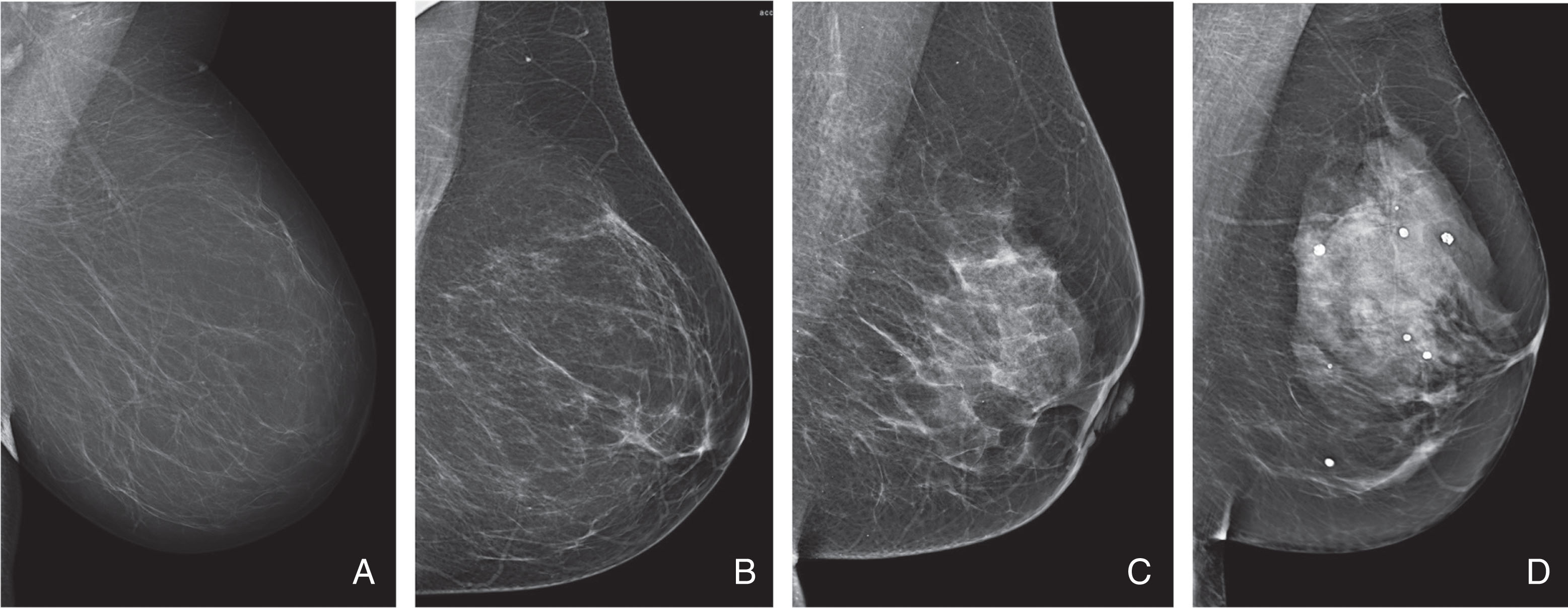
Lesions depicted on mammography and DBT are also reported according to the BI-RADS lexicon. Lesions are being classified as either a mass, calcifications, architectural distortion, or asymmetry. Masses typically have convex margins and are visible in two directions. Assessment is based on their shape, margin, and density. A typical malignant mass is irregular in shape, has a spiculated margin, and is hyperdense compared with the FGT ( Fig. 2.2 ). Typical benign masses are oval, well circumscribed, and hypo- to isodense to the normal breast parenchyma. Calcifications can roughly be divided between calcifications that are certainly benign and those that may be associated with malignant breast lesions. Calcifications that are associated with malignant breast disease in general develop within the ductal structures, either due to impaction of locally trapped fluids or to local necrosis and subsequent calcification. Consequently, these calcifications are tiny and grouped. Single calcifications are hardly ever a cause for worry. Coarse calcifications are likewise virtually always related to benign abnormalities.
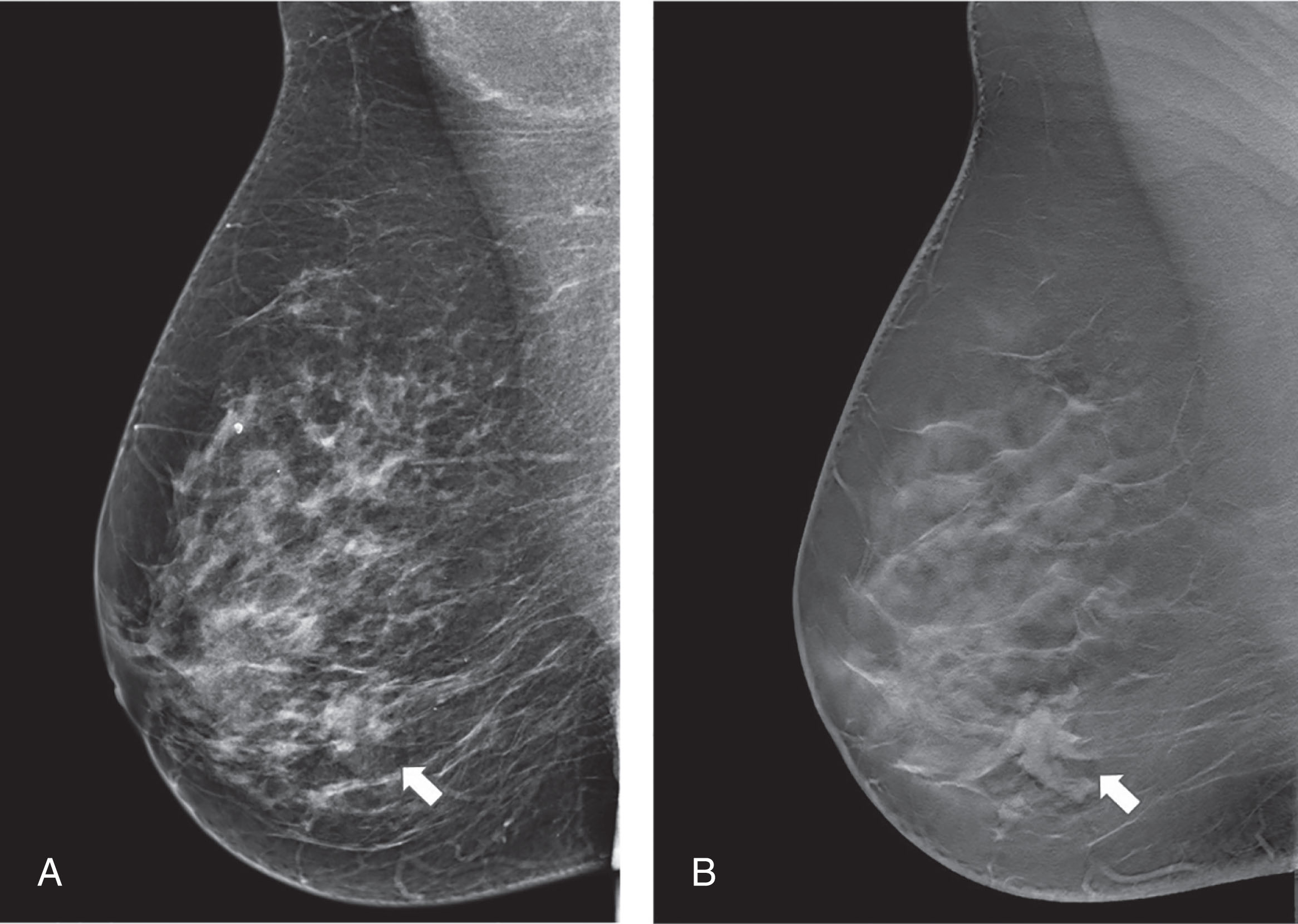
Ultrasound
Ultrasound imaging makes apt use of ultrasonic waves that are emitted by piezoelectric crystals that are typically embedded in handheld transducers. Common frequencies for clinical ultrasound range between 4 and 20 MHz. The emitted sound waves reflect on tissue transition boundaries and are received by the emitting transducer. Assuming a fixed speed of sound of ultrasound waves in soft tissues (roughly 1500 m/s), the time between emission and reception of the reflection can be used to calculate the depth of the tissue boundary that is causing the reflection, which is subsequently translated into an image.
The first ultrasound studies of the breast were reported in 1952. Since then, the quality of ultrasound images has dramatically improved. The current conversion of reflected sound waves into an image is really fast, and ultrasound images can therefore be viewed in real time. For breast imaging, typically, linear, high-frequency transducers (14 MHz or more) are used that create high-resolution images of all the tissue that is directly under the probe. The downside of the use of high-frequency probes is that the penetration depth is limited, and in women with very large breasts, it may be required to use lower frequency probes to visualize the deeper parts of the breast.
Breast ultrasound is mainly a targeted technique, which means that it is used to evaluate focal abnormalities primarily detected by other means. This includes both clinical symptoms (palpable lesions) and findings from other imaging tests. Assessment of ultrasound images is performed using the BI-RADS lexicon for breast ultrasound. Most lesions in ultrasound can be characterized as a mass, for which typically shape, margin, orientation, internal echo pattern, and posterior acoustic features are reported ( Fig. 2.3 ). Associated features include architectural distortion, duct changes, and skin abnormalities. Typical malignant lesions present as irregular hypoechoic masses with posterior acoustic shadowing. Benign lesions tend to be oval, with circumscribed margins and a parallel orientation. Posterior acoustic features are commonly absent. Fluid-rich lesions will show posterior acoustic enhancement.
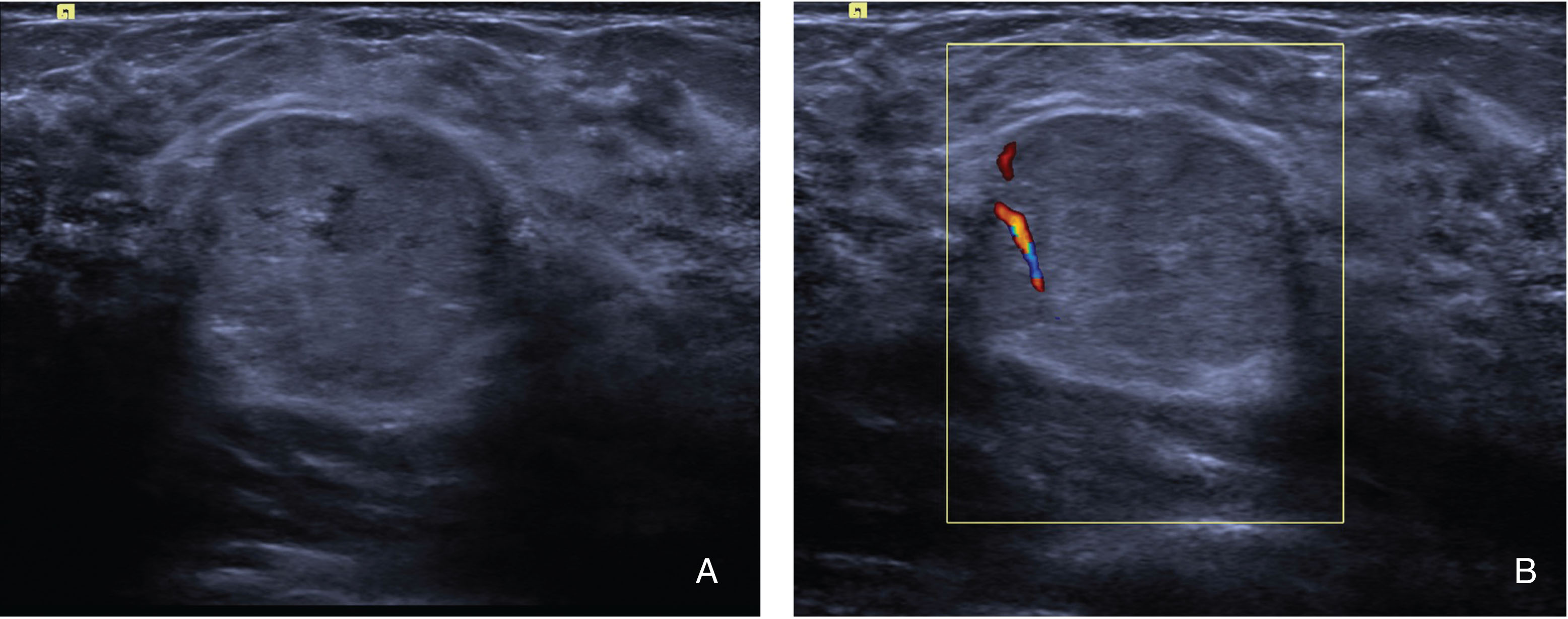
To improve the classification of lesions on ultrasound, the use of Doppler and elastography can be considered. Malignant lesions have a stronger vascularization than benign lesions and a different orientation of feeding vessels (more perpendicular to the lesion), which can be used to strengthen the suspicion. However, the flow in peritumoral vessels is often too slow to be picked up by even state-of-the-art high-resolution Doppler techniques, which implies that absence of Doppler signals is not very informative. Elastography may be used to probe the stiffness of a lesion, as malignant lesions are commonly stiffer than benign lesions; this may be used to avoid biopsy in very soft lesions that are not very suspicious on morphological evaluation but would warrant further evaluation.
MRI
Breast MRI was first employed in the early 1980s. Initial studies used T1 and T2 weighting and showed a similar sensitivity for the detection of breast cancer as could be obtained with mammography. Breast MRI only became viable in clinical practice after the introduction of gadolinium-based contrast media for MRI. These proved to be a real game changer. Contrast-enhanced breast MRI was soon shown to have a much higher comparative sensitivity for breast cancer than either mammography or ultrasound, which is still true despite significant improvements in the other techniques.
Gadolinium-based contrast agents are paramagnetic and therefore enhance T1-weighted relaxation, thus leading to a signal increase in T1-weighted images.
Despite the limited flow signals seen in Doppler ultrasound, virtually all breast cancers are highly vascularized. It is this feature that is exploited by contrast-enhanced imaging. Vessels in malignant breast lesions are different from those observed in healthy tissue. They are the result of angiogenic stimulation by the tumor, and these vessels typically are not properly developed; moreover, they are commonly incapable of dealing with the increased pressure that occurs due to the arteriovenous shunting that occurs in a tumor. Neovascularization is therefore characterized by tortuous vessels with improper endothelial lining, widened interendothelial gaps, and even absence of the basal membrane. In these vessels the applied gadolinium chelates (usually at a dose of 0.1 mmol/kg) can easily extravasate and thus induce a signal change in the extravascular extracellular space.
Current breast MRI thus heavily relies on contrast-enhanced T1-weighted imaging. Adequate enhancement is reached in most lesions approximately 1 to 2 minutes after contrast administration, which is commonly referred to as peak enhancement. This is because in many malignant lesions, maximum enhancement occurs around this moment and contrast starts washing out of the tissue after this time ; however, benign lesions and normal FGT generally reach their peak enhancement only later.
In a standard T1-weighted image, fat also has a high signal, and it can therefore be difficult to observe small enhancing lesions on a postcontrast T1-weighted image only. In practice, this is tackled by two approaches: fat suppression and image subtraction.
Fat suppression, either using fat saturation, water selective excitation, or a Dixon-based approach, enables direct evaluation of the postcontrast acquisitions and is commonly used to observe lesions in the context of the surrounding breast parenchyma. However, it should be realized that some other structures in the breast may also have a high T1 signal that is not affected by fat saturation and may thus resemble enhancing lesions (e.g., commonly a high intensity is observed in the larger ducts due to protein-containing fluid). Subtraction (postcontrast minus precontrast) only shows enhancing structures but is susceptible to motion artifacts. However, when registration of pre- and postcontrast acquisitions is good, subtracted images can be used to generate a maximum intensity projection that enables a direct visualization of most enhancing structures in the breast, which is a good start for the evaluation of breast MRI acquisitions.
Evaluation of breast MRI according to the BI-RADS lexicon starts with the assessment of the amount of FGT and the amount of background parenchymal enhancement (BPE). BPE is assessed in series obtained approximately 90 to 120 seconds after contrast administration and classified as minimal, mild, moderate, or marked. The stronger BPE, the more likely that enhancing lesions are missed, albeit this effect is not as well documented as the effect of density in mammography (marked BPE is relatively rare). BPE is dependent on the menstrual cycle and roughly lowest between day 3 and 14, albeit variation is large. To optimize the value of breast MRI, it is commonly recommended to plan the examination accordingly. It should, however, be noted that in women taking oral contraceptives, the normal menstrual cycle is often absent, and also in women with hormone-releasing intrauterine devices (IUDs) the cycle is not normal (the use of such IUDs tends to lead to quite strong BPE).
Enhancing lesions in the breast are classified as focus, mass, or nonmass enhancement. For masses, further morphological description includes shape, margin, and internal enhancement pattern; for nonmass enhancement, distribution and internal enhancement pattern are reported. Foci are generally smaller than 5 mm, but more importantly, they are not further classifiable as either mass or nonmass enhancement. Like in mammography and ultrasound, most cancers present as an irregular mass with an irregular or spiculated margin. Nonmass enhancement corresponding to malignancy is typically segmental in distribution and shows clumped internal enhancement.
In addition, the physiological enhancement behavior of a lesion is evaluated. As described earlier, the fact that cancers commonly have very leaky vessels leads to rapid early enhancement and wash-out after approximately 2 minutes. This is commonly referred to as a type 3 curve, and is clearly associated with malignancy ( Fig. 2.4 ). A type 2 curve shows a plateau in the late phase (i.e., there is a balance between inflow and outflow of contrast), and a type 1 curve describes continuously increasing enhancement during the period of imaging (typically about 7 minutes). A type 1 curve is rare in malignant lesions but may occur in lesions without extensive neovascularization (e.g., low-grade ductal carcinoma in situ [DCIS] or very diffusely growing invasive lobular carcinoma [ILC]). As the enhancement curves provide a proxy for the leakiness of the vessels in a lesion, similar information can be obtained using ultrafast MRI acquisitions from the inflow phase of contrast (i.e., before peak enhancement is reached). When vessels are large and leaky and shunting occurs, enhancement in a lesion is both early (typically within 10 seconds from the descending aorta) and fast (>13.5%/s), which is typical for malignant lesions. Vice versa, when vessels are not so leaky and shunting is absent, enhancement is later (>15 seconds) and slower (<6.4%/s).
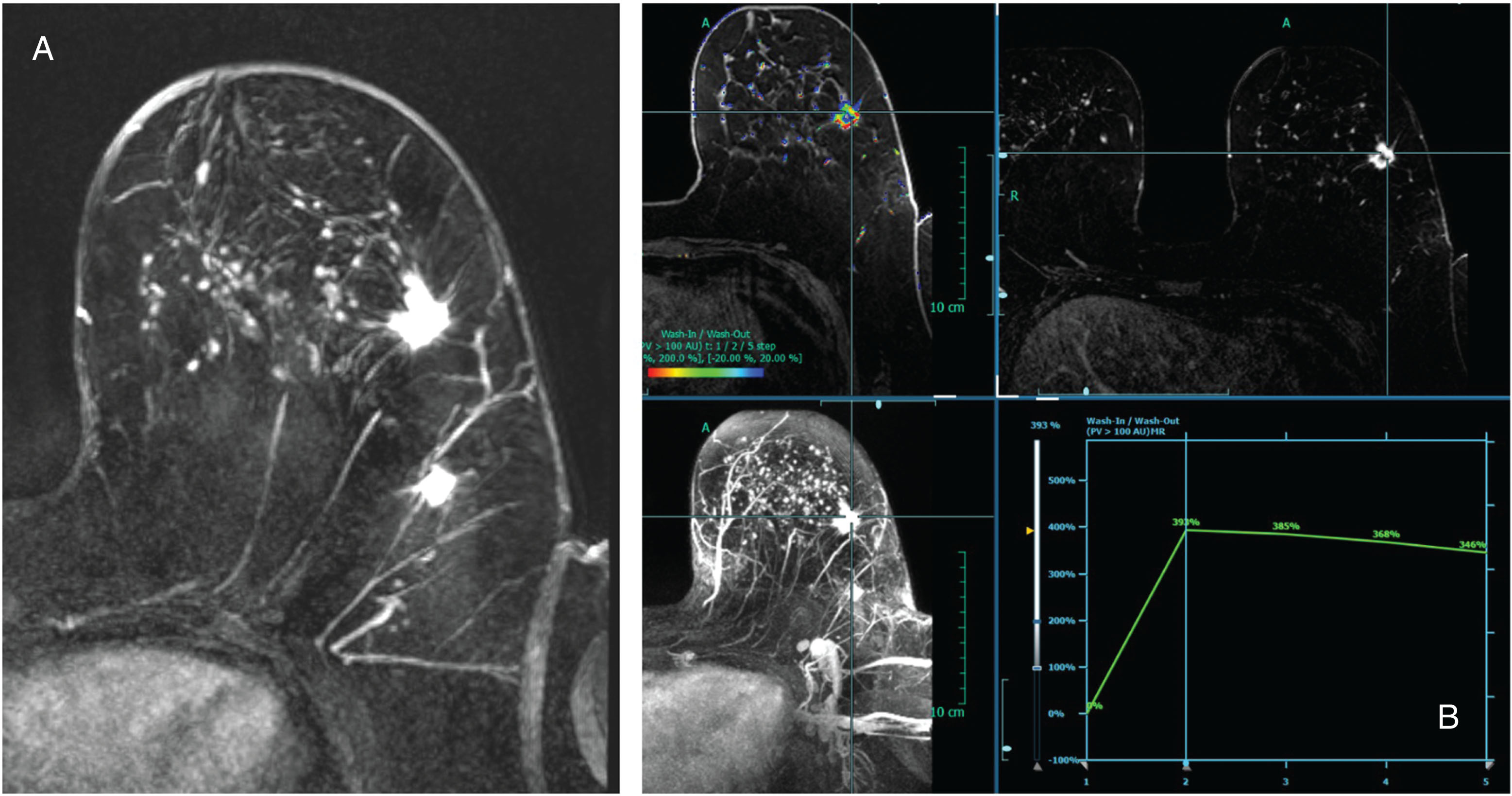
Other contrasts in breast MRI currently provide mainly supplemental value. Besides diffusion-weighted imaging (DWI), which is the topic of this book and therefore not discussed in detail in this chapter, T2-weighted acquisitions are commonly obtained. Fat-saturated T2-weighted acquisitions are particularly good for the documentation of cysts. Non-fat-saturated T2-weighted images often better allow the visualization of the architectural distortion that is caused by the tumor in the breast and the presence or absence of edema surrounding a lesion. Most malignant lesions are relatively hypointense at T2-weighted acquisitions due to a low water content. However, especially high-grade lesions may have a more intermediate T2 signal. Central necrosis may be visualized as high signal within an otherwise low signal intensity lesion. Rapidly enhancing oval masses with a sharp margin and a high T2 signal in young women are usually myxoid fibroadenomas, albeit especially in women at increased risk, care must be taken not to misclassify rapidly growing cancers.
Image-Guided Biopsy
Despite the many classifying features that can be collected from multimodal and multiparametric imaging many breast lesions cannot be fully classified by imaging alone. In lesions that have a likelihood of more than 2% to be malignant (BI-RADS 4; see later), tissue sampling is generally recommended. Besides, in case the imaging features are clearly malignant tissue, sampling is still required to determine optimal treatment, as that is also dependent on receptor status and tumor grade. Hence biopsy is indispensable in breast radiology, and an essential part of the toolbox of each breast radiologist.
Tissue sampling should be an image-guided procedure, and due to the real-time feedback that can be obtained by using ultrasound, this is in general the first choice. Under ultrasound guidance, many different needles can be used. For cytological procedures, a standard 21 G injection needle is usually employed. However, the reported accuracy of cytology is highly variable and largely dependent on the experience of the pathologists involved. Moreover, in malignant lesions, cytology is not able to differentiate between in situ and invasive disease. Consequently, for the assessment of breast lesions, cytology should be used selectively and should be followed by histological tumor sampling when findings are not conclusively benign.
For histological sampling under ultrasound guidance, typically 14 G large-core needles are used that obtain samples with a diameter of about 1 mm (usually about 2 cm in length). The fact that it is possible to document the position of the needle within the lesion enables relatively straightforward radiological-pathological correlation ( Fig. 2.5 ). Typically, two to three cores are sufficient to obtain a reliable diagnosis. Most care should be taken in very small lesions (<5 mm) and solid components in predominantly cystic lesions. Very small lesions may move when firing the needle when they are stiffer than the surrounding parenchyma. Images could, in this case, deceptively show the needle in the lesion when in fact it moved just on its side, and therefore only regular breast tissue is sampled. In predominantly cystic lesions, biopsy might break the wall of the cyst and therefore render small solid components suddenly invisible.
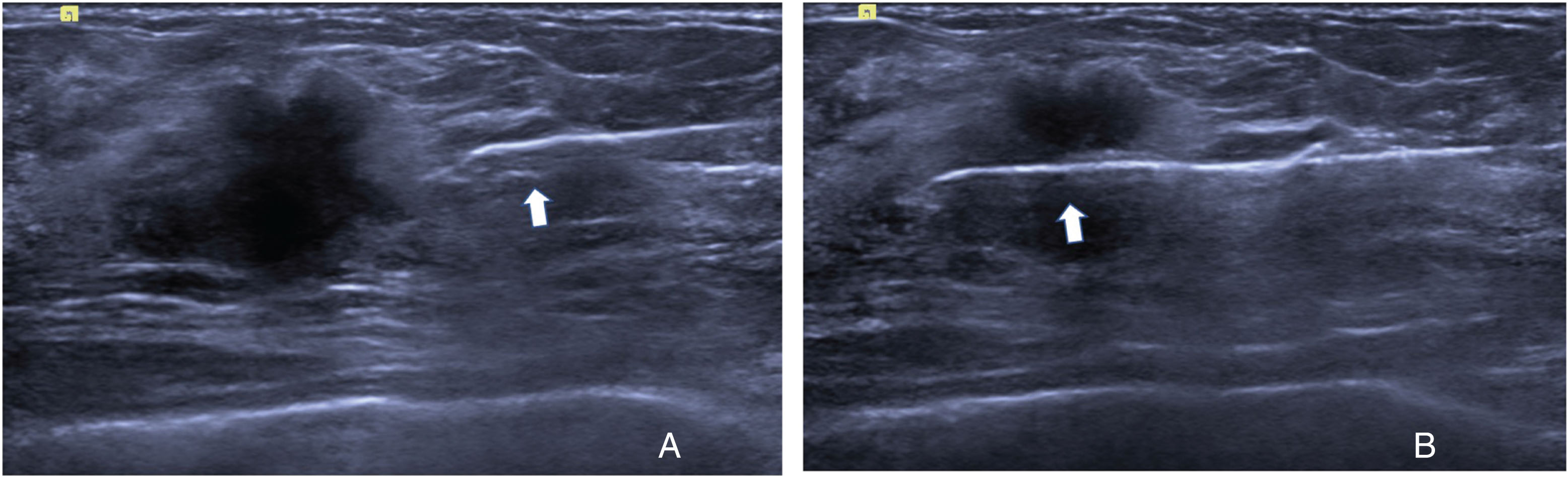
To overcome these issues, it might be feasible to use larger (11–7 G) vacuum-assisted needles. These should be positioned underneath the lesion to be biopsied, with the biopsy chamber pointing toward the transducer. This enables verification of the representativeness of the biopsy sample and therefore may prevent sampling errors.
The same technique can be used to sample more tissue, when an initial large core biopsy reveals lesions of uncertain malignant potential, such as atypical ductal hyperplasia or complex sclerosing lesions. Using this approach, it is also possible to remove lesions completely, which is mainly practiced for the removal of symptomatic fibroadenomas.
Not all lesions are visible with ultrasound, and therefore guidance of the other imaging modalities employed should always be possible. Typical lesions that are only visible on mammography and DBT are grouped calcifications that only rarely have a clear ultrasound correlate. Such calcifications are typically associated with the presence of DCIS but are not very specific, thus these should be biopsied under mammographic or DBT guidance. Classic mammographic guidance makes use of stereotactic views obtained under slight angulation of the x-ray tube to obtain depth information, DBT inherently obtains these views and therefore provides the required depth information automatically. Different from ultrasound guidance, direct imaging feedback of the needle position in the lesion is not possible; rather, correlation is obtained by controlling the needle position before biopsy and the location of the hematoma and marker after the procedure. Furthermore, the lesions are typically more diffuse and therefore harder to classify for pathologists. To obtain a reliable diagnosis, more tissue should be removed than is typically done under ultrasound guidance. Vacuum-assisted biopsy is most common, and six 9 G cores seems to be the minimum. To guarantee that the targeted lesion is indeed biopsied, specimen x-rays are acquired that should show that at least some of the targeted calcifications are removed.
The relatively high sensitivity of MRI for breast cancer implies also that MRI leads to the detection of lesions that have no correlate on other imaging tests and hence should be biopsied under MRI guidance. These include in general small masses and areas of nonmass enhancement. The biopsy procedure is in essence similar to that for stereotactic biopsy. The true 3D nature of MRI makes it relatively easier to check the position of the needle relative to the lesion; however, there is still no real-time imaging feedback. The fact that usually small or diffuse lesions are targeted makes the use of vacuum-assisted needles indispensable. Different from mammographically detected lesions, there is no possibility to check whether the obtained specimen truly includes the targeted lesion. Accuracy of the procedure is therefore checked by assessing whether the location of the hematoma after biopsy corresponds to the location of the original lesion ( Fig. 2.6 ).
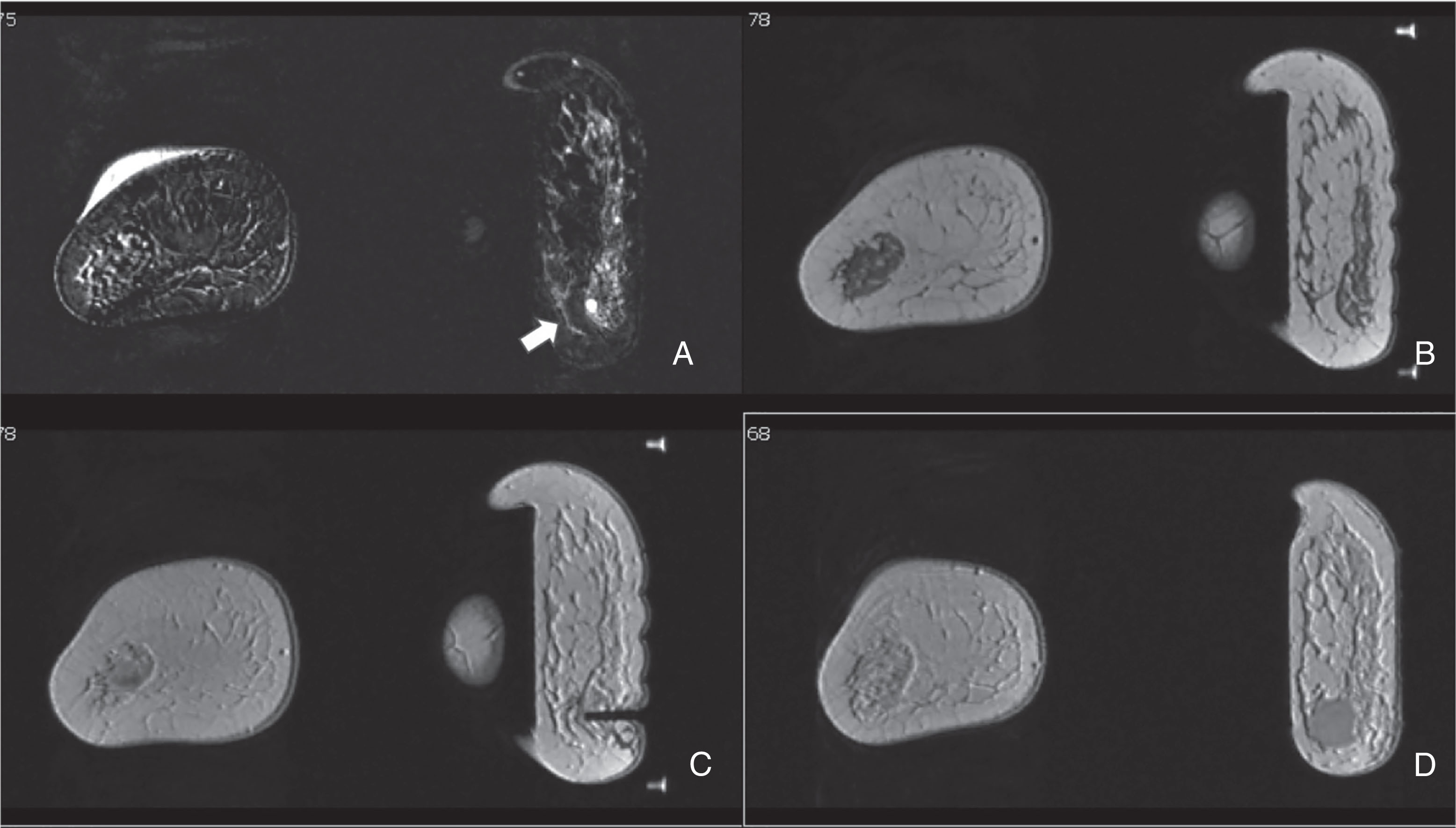
Using Conventional Breast Imaging in Clinical Practice
The type and amount of breast imaging used is highly dependent on the clinical scenario and the questions to be answered. In general, four categories can be discerned: (1) screening of asymptomatic women, (2) assessment of patients with breast findings, (3) staging and monitoring of patients with proven breast cancer, and (4) follow-up of treated patients. The latter basically returns to the first situation (i.e., surveillance is a form of screening). In each setting the demands of imaging differ, and consequently the respective roles of the different imaging modalities vary.
BI-RADS Scores
Assessment of images should lead to a clear evaluation that can easily be understood by others, and that heralds the next steps in the evaluation program. There are several systems around that try to summarize the findings in a number that is simple to interpret. By far, the most commonly used approach is the BI-RADS score, which runs from 0 to 6. BI-RADS scores and their meaning are given in Table 2.1 .
BI-RADS 0 | Incomplete | Additional imaging necessary |
BI-RADS 1 | Negative | No abnormalities |
BI-RADS 2 | Benign | 0% likelihood of malignancy |
BI-RADS 3 | Probably benign | <2% likelihood of malignancy |
BI-RADS 4 | Suspicious for malignancy | 2%–94% likelihood of malignancy |
BI-RADS 5 | Highly suggestive of malignancy | >95% likelihood of malignancy |
BI-RADS 6 | Known biopsy-proven malignancy |
BI-RADS 4 is further subdivided in 4 A (2%–9%), 4B (10%–49%), and 4 C (50%–94%). In essence, BI-RADS scores 4 and 5 imply that a lesion should be biopsied. For lesions interpreted as BI-RADS 3, short-term follow-up may be recommended. However, to downgrade a lesion from BI-RADS 3 to BI-RADS 2 requires 2 years of negative follow-up, and many women prefer biopsy over this extensive surveillance.
Screening of Asymptomatic Women
In 1968 the World Health Organization listed criteria that should be applicable when offering screening for a disease. These criteria are listed in Table 2.2 and are still applicable generally.
|
|
|
|
|
|
|
|
|
|
There is no doubt that breast cancer is an important health problem, considering the fact that in 2018 almost 700,000 women died from this disease, despite the presence of a treatment. Screening for breast cancer is built on the paradigm that breast cancer–specific survival increases because earlier treatment is more effective. It should be realized that this is not necessarily true. When breast cancer is in general already metastasized from its onset, then earlier treatment of the disease locally will not make a difference. Moreover, when tumor stage at detection neither affects survival nor the selection/aggressiveness of therapy, the screening paradigm will be broken. However, currently, this is far from reality.
Randomized controlled trials performed with mammography in the second half of the 20th century proved that the screening paradigm was valid. Average tumor size in most studies decreased, and with it the breast cancer–specific mortality decreased. In meta-analysis of these studies, the invitation to screen leads to a mortality reduction of approximately 20%. This says little about the actual effect of screening, but it also takes the acceptability of the screening test for the population into account. Case-control studies in which women actually screened are compared with those who are not screened report a much higher reduction of mortality due to mammographic screening on the order of 40%. Current international recommendations uniformly recommend screening in women between the ages of 50 and 70. Variations occur due to differences in the age groups invited (range roughly 40–75) and the frequency at which the screening test is offered (annually or once every 2–3 years). These variations are based on the expected efficiency of the screening test in the population and the value attributed to early cancer detection relative to the negative values attributed to the downsides of screening.
Apart from having to undergo the screening test, screening comes with two typical disadvantages. The first is overdiagnosis, which refers to the detection of cancers that would never become symptomatic in the woman’s lifetime. This defines the upper age limit to which screening is useful, because after that age earlier detection of breast cancer is unlikely to contribute to better survival (i.e., the fraction of overdiagnosis goes up with increasing age).
The second disadvantage is the detection of false positives. This refers to a situation in which the screening test points to a possible abnormality that might or might not be breast cancer but that turns out to be benign after further workup. In essence, “false positive” is a misnomer, as screening is aimed to separate women who are most likely cancer-free from those who may or may not have breast cancer. For the latter group, the investigation is simply not finished but requires additional imaging tests or even image-guided biopsy before a final verdict can be given (see Fig. 2.6 ). Nonetheless, such supplemental studies have been shown to temporarily increase the stress women experience and should thus be limited as much as possible. False-positive findings occur more frequently when the screening test is repeated more frequently, and thus they are affected by the screening frequency. However, by comparing the new screening examination to prior studies, the number of false positives can be strongly reduced (depending on the country and the recall policy, between 2% and 10%).
The biggest shortcoming of mammographic screening is not in its findings, however; rather, it is the underdiagnosis of relevant cancers (i.e., the lack of findings in women who actually do have significant breast cancer). This is evident in the fact that even in women screened, a significant fraction of cancers presents with symptoms in between two screening rounds (i.e., interval cancers), and also from the fact that a significant fraction of cancers detected through screening are over 2 cm in size and/or already have lymph node metastasis at the time of detection. Consequently, there is a strong need to improve breast cancer screening.
In the last decade, much effort has been put into evaluation of tomosynthesis as an alternative screening test. Several large case-control studies showed increased cancer detection with tomosynthesis over mammography, usually on the order of 1 to 2 in 1000 screens. In the United States, where recall rates are traditionally high, the use of tomosynthesis leads to an increase in specificity. However, in Europe, where recall rates are traditionally much lower, the effect is reversed, and the increased detection of cancer with DBT also slightly reduces the specificity. Overall, the cancers detected with DBT are similar to those observed on mammography, both in size and nodal status. However, follow-up on several of these studies now seems to show an overall reduction of interval cancers, with reported values ranging from 0% to 40%. Thus DBT seems to be somewhat better than mammography for screening purposes. However, this comes at a price that is mainly problematic in population-based screening programs. Reading tomosynthesis takes on average about twice the time that is required for assessing a mammogram and therefore would require a strong increase in the number of evaluating radiologists in such programs. This is not so much an issue in the United States, where screening is mainly hospital based; therefore DBT has been largely embraced as the new screening modality in the United States, whereas it is not commonly used within Europe for screening.
Ultrasound is not commonly used as a first-line screening technique but rather as a supplement to mammographic screening. Although the sensitivity of ultrasound for breast cancer is likely higher than that of mammography, it is much harder to document the entire breast with ultrasound. A structured assessment of both breasts is reported to take approximately 21 minutes and requires a skilled operator. Because only abnormalities are structurally documented, it is also much harder to compare new ultrasound acquisitions to prior images, which adds to the already high false positive rates. Still, in women with dense breasts, ultrasound is commonly used as a supplemental screening technique. Reported supplemental cancer detection rates after negative mammography range between 1 and 7 in 1000 screens. In direct comparison to tomosynthesis, the yield is approximately double. The use of ultrasound as a supplemental screening technique was reported to reduce the interval cancer rate by 50%. However, reported false-positive rates are up to 25%, which seriously hampers the implementation. In practice, the recall rate is usually lowered by only including clearly solid suspicious lesions and leaving out all probably benign lesions. Consequently, the increase in cancer detection is much lower than in the prospective studies. For example, Lee and colleagues reported no increase in cancer detection in women screened with mammography and ultrasound over mammography alone (5.4/1000 vs. 5.5/1000) but did report a strong increase in false-positive findings leading to biopsy (52/1000 vs. 22/1000). This implies that the use of ultrasound for screening is somewhat questionable even when it is undoubtedly true that ultrasound can show cancers that are not visible on mammography.
Breast MRI has been tested as a supplemental screening modality since the start of this century, and its role has been ever expanding. MRI is primarily used in populations with a high likelihood of developing breast cancer, in which conventional screening with mammography and DBT is particularly poor. Based on a number of large comparative multicenter studies, which showed that breast MRI roughly doubles the sensitivity of mammography, in 2007 the American Cancer Society issued guidelines to define who to screen with breast MRI. They concluded that combined mammography and MRI screening was indicated in women with hereditary breast cancer, including those with BRCA1, BRCA2, PTEN, and STK11 mutations that all lead to a lifetime risk of more than 50% to develop breast cancer. Moreover, screening in these women should start at the age of 25, as they are prone to breast cancer development much earlier than the general population. This list was later supplemented with other genetic mutations that also lead to increased risk for the development of breast cancer, such as CDH1, PALB2, and ATM. Apart from these groups of women with hereditary gene mutations, also women with a personal history of chest irradiation during puberty (usually for lymphoma/leukemia) were deemed eligible for MRI screening, as their risk is more or less similar. Reported positive predictive values of breast MRI in a screening setting range between 20% and 40%; these are generally comparable to those from mammography and much better than those reported for ultrasound. Still, it should be realized that this leads to somewhat higher absolute numbers of false-positive findings, as the number of cancers detected is higher.
Although the American Cancer Society also recommended to screen women with a lifetime risk of between 20% and 50% based on family history with supplemental MRI screening, this recommendation was followed less often due to concerns about cost-effectiveness. However, this was recently shown to be both effective and cost-effective ( Fig. 2.7 ). In the last decade, research on the value of breast MRI for screening focused mostly on women in such intermediate risk groups and even in women at average risk. It was shown that breast MRI has far higher sensitivity than mammography in women with a personal history of breast cancer, women with lobular carcinoma in situ, atypical ductal hyperplasia, and other epithelial atypias on biopsy. Also, MRI outperforms mammography in women with extremely or heterogeneously dense breasts on mammography, and even in women at average risk in general. In a landmark randomized controlled trial in women with extremely dense breasts (Dense Tissue and Early Breast Neoplasm Screening [DENSE]), supplemental cancer detection after a negative mammogram was 16.5 in 1000. It was shown that breast MRI reduces the rate of interval cancers by approximately 85% and causes a major stage shift that leads to all cancers detected in follow-up screening rounds to be stage 0 or 1 and all node negative.
