FIGURE 4-1 Multiplanar reformat image shows angiographicallynormal LMCA and LAD coronary arteries.
FIGURE 4-2 Multiplanar reformat view of the Cx indicates no angiographically definable disease.
FIGURE 4-3 Multiplanar reformat view of the RCA demonstrates an eccentric 90% stenosis of the proximal segment (arrows). Note the presence of both noncalcified (dark) and calcified (bright) plaque in this lesion.
FIGURE 4-4 Invasive coronary arteriography confirms the presence of high-grade stenosis (arrows) in the proximal RCA.
FIGURE 4-5 The same RCA is shown postangioplasty/stent deployed at the site of stenosis.
Clinical Pertinence
Patients with chest pain who are at intermediate risk for coronary atherosclerosis require further risk stratification.1 The presence of a RBBB on the resting ECG precludes interpretation of ECG-only stress testing. A treadmill stress was not feasible in this patient with prior back injury. Diagnostic options include a dobutamine stress echocardiography (DSE), pharmacological stress nuclear imaging, cardiac magnetic resonance (CMR) with pharmacological stress and CT coronary angiography. A low coronary artery calcium score (CACS) and a resting heart rate (HR) of 62 bpm make the patient a good candidate for CT coronary angiography. Difficulty lying still for a prolonged period of time also makes CT coronary angiography, which can be accomplished in a manner of minutes, favorable for this patient. The other diagnostic options are relatively lengthy and require the patient to lie still for longer periods of time. The high-negative predictive value of coronary CT angiography makes it an ideal diagnostic option for this patient, in accordance with the American College of Cardiology/American Heart Association (ACC/AHA) Appropriateness Guidelines.2
CASE 2 New Left Bundle Branch Block
Clinical Scenario
A 59-year-old physician began to experience mid-sternal heaviness while completing a surgical case. He went directly to the Emergency Department where a 12-lead ECG revealed an acute anteroseptal myocardial infarction (AMI). He was taken directly to the cardiac catheterization laboratory where selective coronary arteriography demonstrated thrombotic occlusion of the LAD coronary artery. Guidewire recanalization was accomplished and followed by balloon angioplasty and placement of an intracoronary stent. Postpercutaneous coronary intervention (PCI) angiography demonstrated thrombolysis in myocardial infarction (TIMI) grade 3 flow in the LAD. The patient remained free of chest pain, but noticed a “change in his heart beat” approximately 1 week after discharge from the hospital associated with mild dyspnea on exertion. At the time of a 2-week office visit to his primary care physician, his 12-lead ECG showed a new left bundle branch block (LBBB). A 2D echocardiogram (ECHO) was technically difficult with poor visualization of cardiac structures, including the left ventricle (LV). A cardiac MRI study was ordered.
Description of Images
The coronary arteriogram of the LCA is shown in Figure 4-6. A T2-Short tau inversion recovery (STIR) image in Figure 4-7 demonstrated no residual postinfarction edema. Adenosine stress perfusion imaging (SPI) shown in Figure 4-8 revealed no reversible ischemia. Delayed enhancement imaging with Gd3+ shown in Figures 4-9 and 4-10 revealed a relatively small area of focal septal scarring involving the anatomic location of the LBB. A post-gadolinium enhancement image in the horizontal long-axis plane with TI = 600 revealed a large thrombus along the apicoseptal myocardium and is shown in Figure 4-11.
FIGURE 4-6 Left coronary artery angiography prior to intervention shows subtotal occlusion of the mid-LAD coronary artery (arrow).
FIGURE 4-7 A T2-weighted STIR image demonstrates no residual postinfarction edema.
FIGURE 4-8 A frame from the stress perfusion acquisition shows uniform first-pass perfusion in all myocardial segments during adenosine infusion consistent with absence of ischemia.
FIGURE 4-9 Late post-gadolinium enhancement imaging in the horizontal long-axis plane shows scarring in the mid-septum.
FIGURE 4-10 Late post-gadolinium enhancement imaging in a mid-short-axis plane shows scarring in the septum, where the left bundle of the intracardiac conduction system resides anatomically.
FIGURE 4-11 A post-gadolinium image acquired in the horizontal long-axis plane with TI of 600 milliseconds revealed a large thrombus in the apex of the LV (arrowhead).
Clinical Pertinence
The development of a LBBB after acute coronary syndrome carries an adverse prognosis and is usually accompanied by evidence of LV dysfunction and symptoms of congestive heart failure (CHF).3 LBBB causes septal dyssynchrony (SDS) which results in abnormal intraventricular flow with a decrease in forward cardiac output, increasing the likelihood of stasis and thrombus formation in the LV cavity. Accurate identification of these potential sequelae of a LBBB is crucial to implementing appropriate therapy for LV dysfunction and thromboembolism in a timely manner.4 Although the size of infarction was small and not responsible for severe impairment of LV systolic contractility, the anatomic location of the infarction was clearly responsible for the LBBB and the attendant hemodynamic and thrombotic complications experienced by this patient.
The absence of postinfarction edema on T2-STIR imaging suggests that the LV conduction impairment is not likely to improve in the post–infarction period. The absence of reversible ischemia on cardiac MRI adenosine SPI suggests that ongoing ischemia is not the cause of the new LBBB, avoiding the need for repeat coronary arteriography.
Although development of postinfarction LBBB is associated with an increased likelihood of complete heart block, the cardiac MRI delayed enhancement images showed that the localized area of infarction did not involve other components of the electrical conduction system, making the postinfarction development of complete heart block less likely, obviating the need for repetitive ECG ambulatory monitoring studies.
Lastly, the fortuitous finding of LV thrombus formation on the specialized cardiac MRI imaging sequences, allowed treatment with systemic anticoagulation. Such treatment that is not typically required after MI in this instance could likely have prevented a cerebrovascular accident (CVA), a not uncommon but potentially devastating post-MI complication. Even if the 2D ECHO had been an adequate study, it would not have been able to provide all of the clinically pertinent information elucidated by the cardiac MRI study. The unique ability of cardiac MRI to provide information about postinfarction edema, post-PCI ischemia and exact anatomic location, and extent of infarction in less than 1 hour with no X-ray radiation exposure or iodinated contrast makes it an ideal imaging modality for post-acute coronary syndrome (ACS) patients.
CASE 3 False-Positive Nuclear Stress Perfusion Study
Clinical Scenario
A 64-year-old African-American obese woman (BMI = 46.5 kg/m2) presented to her primary care physician with a 1 month history of chest pain, sometimes related to exertion. She also reported dyspnea on exertion (DOE). Her brassiere size was 46 EEE. Anticipating a high likelihood of breast attenuation artifact, a 2-day high-dose nuclear stress perfusion study was ordered and it demonstrated a nonreversible inferior perfusion defect suggesting inferior infarction. Her gated-SPECT study shows normal LV systolic contractility and segmental wall motion with a global LV ejection fraction (EF) of 64%. Invasive coronary arteriography was recommended by her cardiology consultant, but not pursued because the patient’s weight exceeded the catheterization table limit. A CT coronary angiogram was considered, but not pursued because of increased BMI. A cardiac MRI with adenosine stress perfusion was ordered to corroborate the presence of inferior infarction scar and delineate the extent of any reversible ischemia.
Description of Images
Figure 4-12 is an image of the patient’s torso. Figure 4-13 shows the nonreversible inferior perfusion defect. Figure 4-14 confirms normal left ventricular systolic contractility. Delayed hyperenhancement (DHE) imaging with gadolinium shows normal myocardial viability with no evidence of myocardial fibrosis or infarction in Figures 4-15, 4-16, and 4-17. Figure 4-15 is the mid-level 2-chamber (2 CH) short-axis DHE view, Figure 4-16 is the 2-CH long-axis DHE view and Figure 4-17 is the 4-CH DHE view.
FIGURE 4-12 Left lateral (left panel) and anterior (right panel) views of the patient’:s torso indicate large breasts and increased abdominal girth, both sources of potential attenuation artifact on nuclear scintigraphic imaging.
FIGURE 4-13 Single photon emission computed tomography (SPECT) images rendered after typical nuclear postprocessing indicate a non-reversible inferior perfusion defect in both reconstructed sections and polar plots.
FIGURE 4-14 Normal ventricular systolic function is indicated by thickening of all segments in this end-systolic frame of a short-axis cine cardiac magnetic resonance acquisition.
FIGURE 4-15 Late post-gadolinium enhancement imaging in a mid-short-axis plane does not indicate infarct scar consistent with viable myocardium.
FIGURE 4-16 Late post-gadolinium enhancement imaging in the vertical long-axis plane shows no infarct scar consistent with viable myocardium.
FIGURE 4-17 Late post-gadolinium enhancement imaging in the horizontal long-axis plane indicates nonscarred, viable myocardium.
Clinical Pertinence
Obese patients and women with large breasts have an increased likelihood of having a false-positive nuclear stress because of breast and diaphragm artifact, even with attenuation correction.5 Coronary CT angiography and invasive coronary arteriography are diagnostic options, but have limited application in the morbidly obese patient. Cardiac MR image quality is not degraded by obesity or overlying breast tissue. Cardiac MRI stress testing with adenosine or dobutamine warrants consideration as the preferred diagnostic procedure in obese patients and women with large breasts.
CASE 4 False-Negative Nuclear Stress Perfusion Study
Clinical Scenario
A 58-year-old Caucasian man presented to his internist with symptoms of exertional chest pain, which began 2 weeks earlier. His Framingham score indicated intermediate risk, prompting further testing. A nuclear stress perfusion study was ordered. The patient completed 9 minutes of the Bruce protocol, reaching 100% of predicted HR, and developed 1.5 mm of horizontal ST segment depression. At peak exercise he had minimal chest discomfort. The nuclear stress and rest perfusion images were normal. His exertional chest pain persisted, prompting cardiology consultation. A coronary CT angiogram was ordered.
Description of Images
The normal nuclear stress study is shown in Figure 4-18. Figure 4-19 is a coronary CT angiographic multiplanar reformat view of the LAD coronary artery in the left anterior oblique (LAO) projection demonstrating significant stenosis of the proximal vessel. A magnified multiplanar curved reformat view of the proximal LAD in the LAO cranial projection is depicted in Figure 4-20, confirming severe stenosis and demonstrating associated mural calcification along the medial wall of the LAD at its origin. Straight lumen and cross-sectional views are shown in Figure 4-21 demonstrating greater than 90% cross-sectional atherosclerotic obstruction of the proximal LAD lumen. Figure 4-22 depicts the invasive coronary arteriographic images of the proximal LAD before and after PCI.
FIGURE 4-18 Myocardial perfusion by nuclear scintigraphic imaging (spatial resolution of 1 cm) does not demonstrate myocardial ischemia.
FIGURE 4-19 Coronary CT angiographic multiplanar reformat view of the LAD coronary artery in the LAO projection demonstrating significant stenosis of the proximal vessel (arrow).
FIGURE 4-20 A magnified multiplanar curved reformat view of the proximal LAD in the LAO cranial projection is depicted, confirming severe stenosis and demonstrating associated mural calcification of the LAD ostium.
FIGURE 4-21 Straight lumen and cross-sectional views are shown demonstrating greater than 90% cross-sectional stenosis due to atherosclerotic plaque of the proximal LAD.
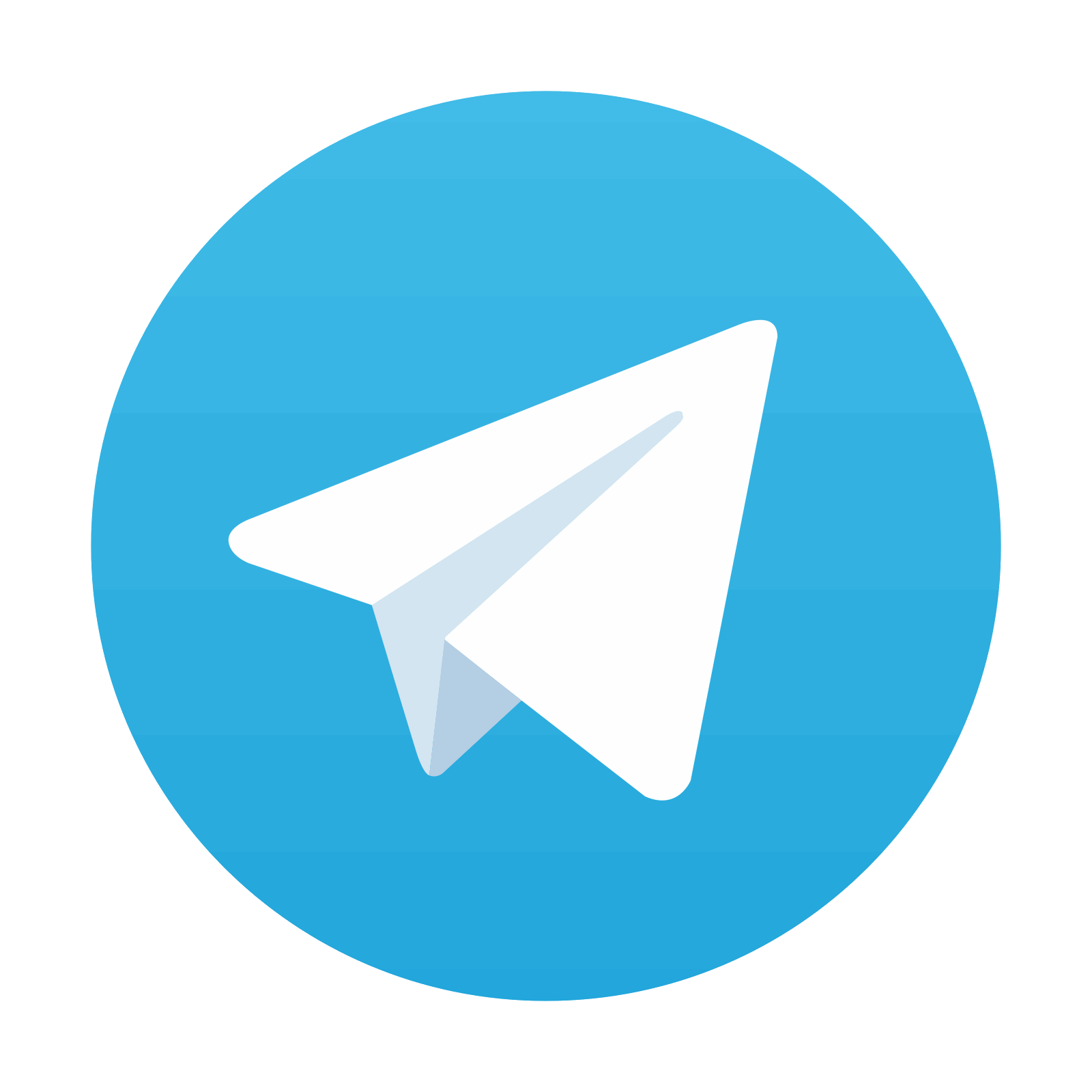
Stay updated, free articles. Join our Telegram channel

Full access? Get Clinical Tree
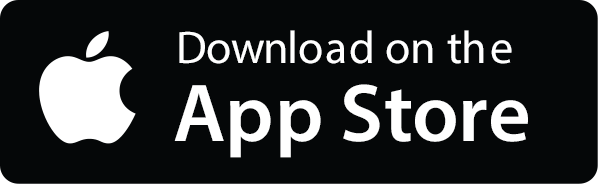
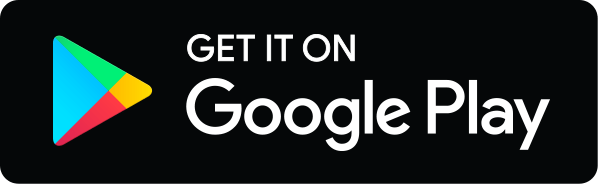