Damage to the spinal cord may be caused by a wide range of pathologies and generally results in profound functional disability. A reliable diagnostic workup of the spine is very important because even relatively small lesions in this part of the central nervous system can have a profound clinical impact. MR imaging has become the method of choice for the detection and diagnosis of many spine disorders. Various innovative MR imaging methods have been developed to improve neuroimaging, including better pulse sequences and new MR contrast parameters. These new “cutting-edge” technologies have the potential to impact profoundly the ease and confidence of spinal disease interpretation and offer a more efficient diagnostic workup of patients suffering from spinal disease.
Over the past 20 years, imaging technology has revolutionized medical care, establishing radiologic evaluation as a vital part of patient management. General practitioners, and medical and surgical subspecialists, now rely heavily on imaging to establish and confirm diagnoses, and plan and monitor treatments. MR imaging is at the forefront of ever-changing and improving technology and has now become a practical and widely available tool for the diagnosis of a range of diseases. In the spine, MR imaging is the primary imaging modality for detecting disease because no other modality can provide the adequate contrast resolution necessary to differentiate the intraspinal soft tissue structures, and to reveal spinal cord or canal pathology. In addition, MR has proved to be the most sensitive tool for detecting infiltration of bone marrow . Although the development of Multidetector CT (MDCT) has made major strides, affording rapid imaging and outstanding spatial resolution, its application to the spine has been limited thus far because of limited tissue contrast and artifacts from adjacent bones and surgical material.
Standard structural MR imaging sequences, including T1- and T2-weighted spin-echo (SE) and fast spin-echo (FSE), (spoiled) gradient-echo (GRE), and contrast-enhanced images, provide most of the information required for detecting and characterizing spinal pathology and achieving a differential diagnosis. Therefore, improvements to these basic sequences, generating greater tissue contrast, better spatial resolution, and decreased motion and susceptibility artifact, likely will provide the most significant gains in MR evaluation of spinal disease. As demonstrated in the brain, advanced techniques can provide additional information that increases the sensitivity and specificity of diagnosis, and more detailed physiologic or anatomic information that can help the referring clinician in guiding management. Diffusion-weighted (DW) imaging, diffusion tensor (DT) imaging and tractography, perfusion, MR spectroscopy, and functional MR imaging (fMR imaging) sequences are now often part of a routine workup in the brain for the assessment of strokes, tumors, and inflammatory lesions. Although equally promising for the diagnostic workup of spine patients, these techniques are seldom used in spine imaging because of the technical challenges that limit image quality, including the highly magnetically inhomogeneous material surrounding the spinal canal, the small size of the spinal structures, the relatively large craniocaudal extent of the spine, cerebrospinal fluid (CSF) and blood pulsation, respiration, swallowing, and bulk motion. In addition, a substantial amount of spine patients who would require radiologic workup have to be turned away or receive inadequate imaging results because of metal artifacts adjacent to the diagnostically relevant regions.
Advances in spinal imaging depend on improvements in both MR imaging hardware and software. In the last decade, spine MR imaging has benefited the most from the introduction of phased-array coil technology and increased field strength, both of which increase the baseline signal-to-noise ratio (SNR) of a study, which is a major factor for successful spine studies that are notoriously SNR-deprived. With the recent advent of parallel imaging , multielement radio frequency (RF) coils have been improved and have enhanced the SNR of high-resolution MR imaging over a large cephalo-caudal extent. With the availability of combined head and spine arrays, the entire spine can be imaged (either stepwise or by continuously moving the patient table) without repositioning the patient and changing the coil ( Fig. 1 ). This advantage is of great relevance because often, the disease of a patient (eg, multiple sclerosis [MS] or neurofibromatosis) requires a total workup of the entire central nervous system and repositioning the patient is often tedious and associated with additional patient discomfort. In addition to the application of new contrast mechanisms and functional studies, structural imaging sequences have matured further, providing better SNR and spatial resolution within dramatically shortened imaging times. Similar to MDCT, there is a new trend toward volumetric acquisition of spine MR imaging data on the horizon, which will afford multiplanar or curved-planar reformations ( Fig. 2 ).
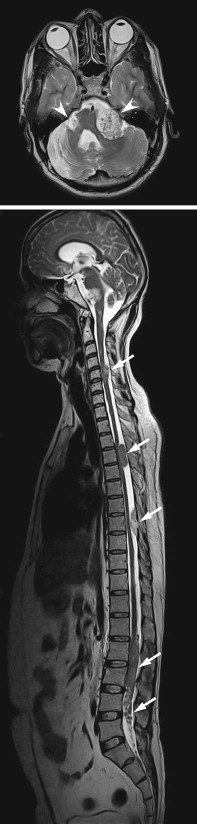
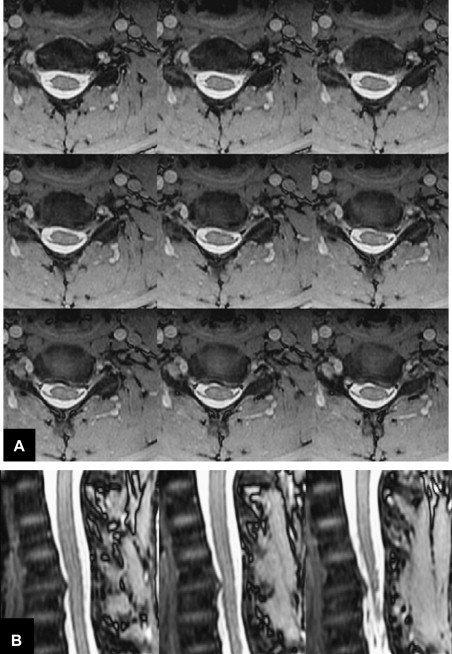
Although “cutting-edge” technology often implies that the technology being developed is not available readily to every user or is still under investigation, most of the sequences discussed are, in fact, currently or soon to be available from most MR imaging vendors and could be implemented in typical radiology practices. The techniques discussed can be supported at a field strength of 1.5 T, but their usefulness at 3 T makes them more popular in clinical settings, so they could be incorporated into routine protocols. The authors describe these advanced sequences in the context of common clinical scenarios, and address how such sequences may help answer clinical questions with greater accuracy and precision.
Degenerative disease and chronic instability
One of the most common indications for spine imaging is the evaluation of back pain and radicular symptoms caused by degenerative disc disease and facet arthropathy . The established role of MR imaging in this clinical setting is to identify the causes of the nerve root compression (including disc protrusions, osteophytes, and synovial cysts) and to assess the severity of spinal stenosis, and to exclude other conditions, such as infection and neoplasm, which may not be suspected clinically .
The high sensitivity of MR imaging studies is important because additional invasive testing, such as nerve root and facet blocks or discography, may be required in cases where standard MR imaging sequences fail to reveal a specific source of pain. Improved SNR with imaging at 3 T ( Fig. 3 ), and decreased CSF pulsation artifact and improved spatial and contrast resolution using the new pulse sequences described below are likely to improve sensitivity for identifying subtle abnormalities. Such advances will provide the greatest advantage in the cervical spine, where small disc spaces and the relative paucity of epidural fat make delineation of disc protrusions more challenging than in the thoracic and lumbar spine.
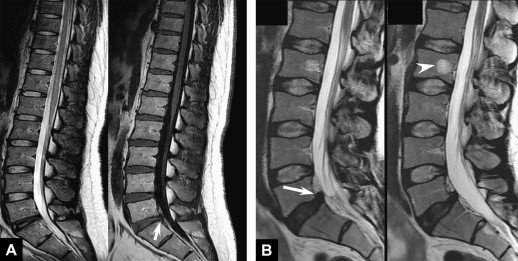
In addition to identifying a possible source of back pain and radicular symptoms, referring clinicians also look to MR imaging findings to guide surgical intervention. Detailed information regarding spinal canal stenosis, neural foraminal narrowing, and compression of nerve roots and the spinal cord can tip the balance in favor of surgical, rather than conservative, treatment. The identification of an extruded or sequestered disc fragment is vital information for the surgeon before intervention. MR imaging findings must be interpreted together with clinical information because symptomatic lesions cannot always be differentiated from asymptomatic lesions purely on the basis of imaging . However, accurate delineation of the contents of the spinal canal and neural foramina may improve the diagnostic yield of MR imaging. Lesions that are associated with nerve root compression are more likely to be symptomatic, and therefore improved visualization of individual nerve roots and surrounding CSF, and more accurate assessment of the caliber of the spinal canal and neural foramina, are likely to improve the correlation of MR findings with treatment outcomes and help guide appropriate therapy .
For practical purposes, a short standard protocol, including sagittal T1- and T2-weighted sequences and axial GRE or T2, is desirable for screening the large number of patients referred for back pain. Improving the speed of these acquisitions with parallel imaging is helpful not only to improve patient throughput but also to minimize discomfort for patients who suffer back pain and may have difficulty lying in a fixed position for long periods of time. In short, parallel imaging uses multiple small coils that are part of a phased-array coil, each of which has a different signal reception characteristic, to provide complementary image encoding through coil sensitivity, in addition to regular gradient encoding .
Axial GRE is used typically to assess degenerative disease in the cervical spine. Unlike with SE and FSE sequences (also known as turbo spin-echo), disc material (which is hyperintense on GRE) and osteophytes (which are hypointense) usually can be differentiated with GRE, regardless of flip angle . In addition, the small size of the cervical disc spaces requires the use of contiguous slices, which is less feasible with FSE or SE. However, GRE often suffers from limited gray matter/white matter contrast and contrast to visualize nerve roots and foraminal stenoses better. Recently, a GRE method with multiple bipolar GRE formations has been introduced that combines the signal from the individual echoes. Here, early echoes provide increased SNR, and later echoes boost contrast ( Fig. 4 ). This sequence type is known as Multiple Echo Recombined Gradient Echo (MERGE) or Multi Echo Data Image Combination (MEDIC) and can be performed as either a 2-D or a 3-D sequence. For the cervical spine, typical scan parameters are as follows: 650 ms/27 ms repetition time/echo time, 4 mm section thickness, 16 cm field of view, 512 matrix, 2 × parallel imaging acceleration. Three-dimensional imaging confers an extra advantage in the cervical spine, enabling sagittal oblique reformats to be generated that can demonstrate the obliquely oriented cervical neural foramina en face.
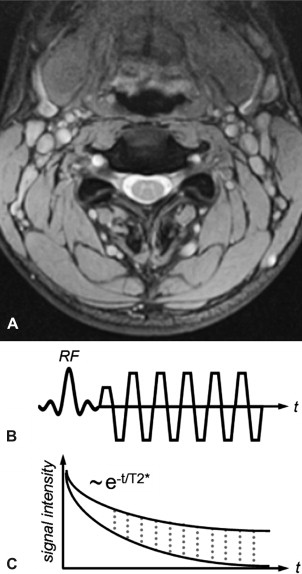
Balanced steady-state free precession (bSSFP) sequences also have an inherently high contrast between tissue and fluid. Moreover, compared with unbalanced steady-state free precession (SSFP) sequences (ie, the net gradient area within one repetition time (TR) is not zero), bSSFP provides high baseline SNR. Thus, it provides an efficient alternative sequence for better detection of herniations, sequestrations, or nerve root compression. Conversely, the gray matter/white matter contrast of conventional bSSFP is relatively poor. Modifications to the sequence, specifically ramping the flip angles up and down to increase contrast and SNR, have been suggested under the name Coherent Oscillatory State acquisition for the Manipulation of Image Contrast (COSMIC) (see Fig. 2 ).
SE and FSE sequences provide good anatomic detail in spine imaging and are favored for the evaluation of spinal canal diameter and for the detection of spinal cord abnormalities, with less susceptibility artifact from bone and improved contrast between gray and white matter structures within the cord, compared with GRE. Given the abundance of epidural fat within the lumbar spine, and the relatively large disc spaces, FSE sequences are favored over GRE in the lumbar spine to assess for focal disc protrusions and nerve root compression. However, the CSF adjacent to the cord, together with cord motion, often causes ghosting artifacts in conventional Cartesian imaging, and is particularly problematic in FSE T2-weighted images. Such artifacts create a substantial challenge for the radiologist in identifying small structures, such as nerve roots, within the CSF space. Radial sampling, in particular when combined with PROPELLER-type acquisitions, is less sensitive to these types of distortions and improves the diagnostic quality of such sequences ( Fig. 5 ). Recently, this method has been combined with fast-recovery (FR)-FSE sequences . The benefit of FR-FSE over conventional FSE is the dramatically reduced TR, at similar, or even improved, T2 contrast between tissue and fluids . With FR-FSE, assuming that the transverse signal of tissue (short T2) has decayed away at the end of the FSE train, a negative 90° (echo reset) pulse orients spins with long T2 (eg, fluid) from the transverse plane back along the longitudinal direction, leading to a much faster recovery of long T2 components to the equilibrium signal and thus, better contrast between long and short T2 species. FR-FSE has demonstrated great usefulness also for 3-D acquisitions because the TR, and thus the imaging time, can be reduced substantially .
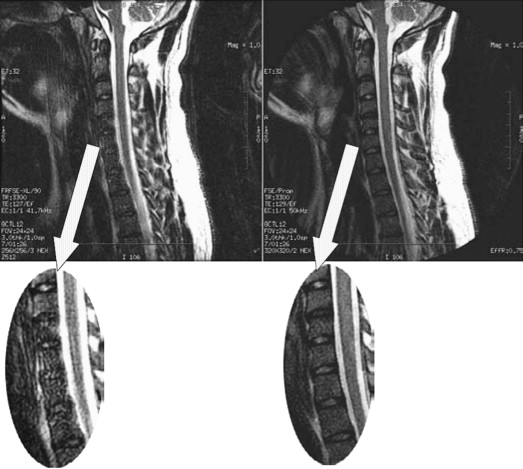
Additional sequences may be helpful in the face of persistent unexplained symptoms, or for more complex or specific questions about anatomy or the influence of patient position on alignment and stenoses. Contrast-enhanced T1-weighted images with fat saturation can reveal facet joint pathology, spondylolysis, spinal degenerative/inflammatory changes, and changes within the paraspinal muscles, which are not always evident on conventional imaging . Some studies also suggest that MR performed with axial load may add sensitivity and specificity to the evaluation of spinal stenosis and nerve root compression . Similarly, dynamic imaging, with patients positioned to reproduce symptoms, may improve the ability to identify significant lesions. Often, abnormalities are apparent only during weight bearing in the upright position, or by flexion or extension of the spine. Although weight bearing can be studied in certain interventional magnets that have enough aperture to allow the patients to sit in an upright position, flexion and extension or lateral flexion can be also accomplished in conventional magnets by using specific positional devices that allow different degrees of flexion/extension ( Fig. 6 ). Certainly, great care has to be exercised when applying these maneuvers, and the selection of patients who might qualify for these kinds of tests should be made only with the referring orthopedic surgeon or neurologist.
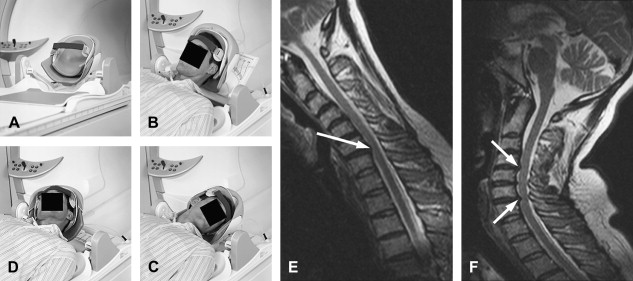
MR imaging may provide important prognostic information regarding the potential for recovery following decompressive surgery in patients with long-standing myelopathic symptoms. High signal within the compressed cord on conventional T2-weighted sequences is nonspecific, representing a combination of myelopathic changes and surrounding edema. DW imaging may provide greater specificity than conventional sequences regarding which changes in the cord are irreversible. DW imaging is an MR technique that is sensitive to the random motion of water molecules in tissues over microscopic distances, and it can generate a map of the average apparent diffusion coefficient (ADC). Reduced ADC is seen in acute cerebral stroke and aids early detection of infarcts, improving accuracy over conventional MR imaging. DW imaging also has become an invaluable technique for assessing other intracranial diseases, with applications in infection, tumors, and cysts. In one of the authors’ studies , they found that spondylotic myelopathy presented with reduced ADC values, whereas the surrounding cord demonstrated elevated diffusivity. Presumably, the former is caused by either cord compression or vascular compromise, whereas the latter is caused by surrounding edema.
Postoperative evaluation
The challenge presented to radiologists by the postoperative spine is twofold:
- 1.
Metallic surgical implants may produce artifacts that obscure anatomic detail.
- 2.
Postsurgical reactive inflammatory changes can be difficult to distinguish from residual or recurrent disease or postsurgical complications such as abscess or seroma.
Metal in the area being imaged is problematic for both CT and MR. The concern for MR is the significant susceptibility distortions caused by the metal adjacent to the tissue. Although the metal itself cannot be imaged, these susceptibility changes in the proximity of the metal (eg, pedicle screws, metal fixation rods, metal cages, or endplates of artificial discs, and so forth) can lead to geometric distortions and signal loss or pile-up. Signal loss and geometric distortions can be reduced by smaller voxel sizes or thinner slices and excessive RF refocusing. Recently, new variants of 3-D FSE sequences (FSE-XETA, T2-SPACE, VISTA) have been introduced that differ from conventional FSE sequences by their excessively long FSE readout ( Table 1 ). Here, a readout train comprises up to 200 echoes obtained at a minimum echo spacing, and allows image formation very rapidly, altogether diminishing artifacts ( Fig. 7 ). A specific hallmark of these sequences is the flip angle modulation during the FSE readout. Here, the focus is to carry along magnetization as long as possible to avoid blurring and to provide optimal signal at the effective echo time (TE), ie, when the center k-space lines are acquired ( Fig. 8 ). Using that regime, the specific absorption rate can also be diminished substantially. The volume acquisition follows a new trend that has been carried over from MDCT. By acquiring high-resolution 3-D volumes and subsequently generating multiplanar reformats or even curved-planar reformats, imaging could be made much more efficient. However, it remains to be shown whether these reformats are of sufficient quality and detail to replace additional, conventional cuts. By additional modification to the pulse sequence, different contrast and better gray/white differentiation can be achieved ( Fig. 9 ).
Vendor | |||
---|---|---|---|
Pulse sequence | GE | Philips | Siemens |
Multiecho gradient echo acquisition | MERGE | — | MEDIC |
Volumetric FSE | FSE-XETA | VISTA | SPACE |
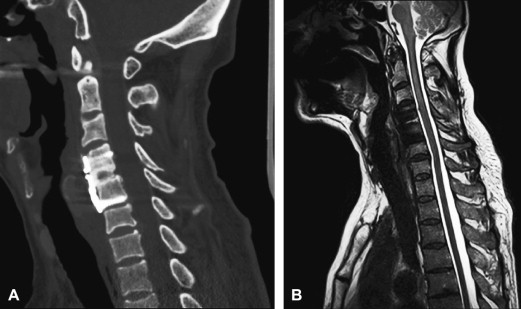
Gadolinium-enhanced imaging is vital after surgery. Enhancement identifies areas of inflammatory postsurgical change, and recurrence of disease. Following diskectomy, recurrent disc protrusions are identified by their lack of contrast enhancement in comparison to uniformly enhancing scar tissue. After tumor resection, non-neoplastic enhancement can develop quickly because of postsurgical inflammation and neovascularity, and has been described even within the first 24 hours of brain surgery . Early imaging following tumor resection maximizes the radiologist’s ability to distinguish a residual enhancing tumor from postsurgical changes, and helps establish a postoperative baseline for the patient. In on-going tumor surveillance, it is important to have high-quality, fat-saturated enhanced images to be able to identify areas of subtle new nodular enhancement representing tumor on the background of postsurgical scarring. Homogeneous fat saturation is critical when obtaining contrast-enhanced T1-weighted images, to avoid obscuring enhancement with signal from epidural fat and fatty vertebral body marrow.
Often, because of the harsh magnetic environment in and around the spine, or because of the presence of surgical material, frequency-selective, fat-suppression techniques or spectrally-selective excitation pulses are suboptimal ( Fig. 10 ). Therefore, short-tau inversion recovery (STIR) techniques are used frequently, despite their obvious SNR penalty and the potential for altered contrast in the presence of contrast material. Recently, a variant of Dixon imaging has been introduced that has proved to be very robust ( Fig. 11 ) and provides increased SNR because of the combination of measurements. Especially for scans with number of excitations (NEX) greater than one, it appears to make sense to acquire data at slightly different echo times and to combine the data with the aforementioned iterative Dixon technique. One challenge for the Dixon method is too-rapid field fluctuations, causing the underlying phase maps needed for fat-water separations to fail.
DW imaging may be helpful in early postoperative follow-up as well. After surgery, patients may develop nonspecific fluid collections in the paraspinal soft tissues. Often, these represent seromas that will resolve gradually over time, with conservative management. Such benign collections do not demonstrate restricted diffusion, whereas frank pus within an abscess typically shows high signal on DW imaging, with reduced ADC . DW imaging may be helpful also to distinguish ischemic injury to the paraspinal muscles from reactive enhancement caused by retraction during surgery .
Postoperative evaluation
The challenge presented to radiologists by the postoperative spine is twofold:
- 1.
Metallic surgical implants may produce artifacts that obscure anatomic detail.
- 2.
Postsurgical reactive inflammatory changes can be difficult to distinguish from residual or recurrent disease or postsurgical complications such as abscess or seroma.
Metal in the area being imaged is problematic for both CT and MR. The concern for MR is the significant susceptibility distortions caused by the metal adjacent to the tissue. Although the metal itself cannot be imaged, these susceptibility changes in the proximity of the metal (eg, pedicle screws, metal fixation rods, metal cages, or endplates of artificial discs, and so forth) can lead to geometric distortions and signal loss or pile-up. Signal loss and geometric distortions can be reduced by smaller voxel sizes or thinner slices and excessive RF refocusing. Recently, new variants of 3-D FSE sequences (FSE-XETA, T2-SPACE, VISTA) have been introduced that differ from conventional FSE sequences by their excessively long FSE readout ( Table 1 ). Here, a readout train comprises up to 200 echoes obtained at a minimum echo spacing, and allows image formation very rapidly, altogether diminishing artifacts ( Fig. 7 ). A specific hallmark of these sequences is the flip angle modulation during the FSE readout. Here, the focus is to carry along magnetization as long as possible to avoid blurring and to provide optimal signal at the effective echo time (TE), ie, when the center k-space lines are acquired ( Fig. 8 ). Using that regime, the specific absorption rate can also be diminished substantially. The volume acquisition follows a new trend that has been carried over from MDCT. By acquiring high-resolution 3-D volumes and subsequently generating multiplanar reformats or even curved-planar reformats, imaging could be made much more efficient. However, it remains to be shown whether these reformats are of sufficient quality and detail to replace additional, conventional cuts. By additional modification to the pulse sequence, different contrast and better gray/white differentiation can be achieved ( Fig. 9 ).
