Diffusion-weighted imaging and fractional anisotropy may be more sensitive than other conventional magnetic resonance imaging techniques to detect, characterize, and map the extent of spinal cord lesions. Fiber tracking offers the possibility of visualizing the integrity of white matter tracts surrounding some lesions, and this information may help in formulating a differential diagnosis and in planning biopsies or resection. Fractional anisotropy measurements may also play a role in predicting the outcome of patients who have spinal cord lesions. In this article, we address several conditions in which diffusion-weighted imaging and fiber tracking is known to be useful and speculate on others in which we believe these techniques will be useful in the near future.
Magnetic resonance (MR) imaging plays a major role in the diagnosis and follow-up of spinal cord lesions. The main objectives of spinal cord imaging are to detect and characterize lesions, to assess the feasibility of surgical resection, and to diagnose recurrences and complications of therapy. Conventional MR imaging using T1- and T2-weighted sequences (in spin or gradient echo) lacks sensitivity in detecting and characterizing cord lesions, such as multiple sclerosis or acute spinal cord infarction. In addition, in patients who have cord tumors, conventional sequences may not be able to clearly identify the transition between the tumor and the surrounding edema. In the brain, diffusion-weighted (DW) imaging is an established and reliable method that helps to detect and characterize such lesions, and diffusion tensor (DT) imaging is becoming an important technique to identify white matter tracts and the effects of different lesions on them. DW imaging and DT imaging are usually performed using echo planar sequences, which are sensitive to noise, motion, and susceptibility artifacts. These two caveats make it difficult to detect and characterize spinal cord lesions, particularly with DW imaging. In addition, the resolution of most currently clinically used DW imaging sequences is not optimal to image structures as small as the spinal cord and its internal features. Better characterization of white matter lesions (and therefore many cord lesions) may be achieved using DT imaging, an MR technique that evaluates the movement of extracellular water molecules within white matter fibers and enables the reconstruction of three-dimensional images of white matter tracts using specialized fiber tracking (FT) algorithms .
Recently, several investigators have assessed the feasibility of performing spinal cord DT imaging studies . DT imaging sequences with computation of fractional anisotropy (FA) are more sensitive than spin echo T2-weighted images in detecting intrinsic abnormalities in acute or chronic spinal cord compression . In lesions that produce involvement of white matter fibers, it has also been reported that DT imaging with FT may help to define abnormal areas that are undetected on routine T2-weighted imaging . In our experience, FA and FT maps derived from DT imaging computations may help neurosurgeons to better delineate spinal cord tumors and may contribute important information before tumor resection.
In this article, we review the different methods available to obtain DT imaging and FT in the spinal cord and their clinical applications. We discuss novel and dedicated spine FT programs and speculate about the future of DT imaging and FT in spinal cord imaging.
Diffusion tensor imaging and fiber tracking methods
Image acquisition
DT imaging may be reliably performed on 1.5 T MR imaging systems with actively shielded magnetic field gradients, but strong gradients are needed (≥30 mT/m) for optimal imaging. To decrease magnetic susceptibility artifacts intrinsic to echo planar DT imaging sequences, parallel imaging with the shortest echo time is desirable. The spinal cord is a small organ with less extracellular water than the brain. Consequently, small b values are required to prevent signal attenuation on DW imaging. SENSE or GRAPPA echo planar imaging or multishot fast spin echo sequences obtained with a b value of approximately 500 s/mm 2 are most advantageous because they reduce magnetic susceptibility artifacts and result in shorter acquisition times . Several DT imaging gradient directions are needed, and theoretically more directions result in a better signal-to-noise ratio and better diffusion ellipsoids. Investigators have tested 6, 12, 25, and 55 directions and have concluded that the best compromise between acquisition time and image quality is achieved with 25 directions ( http://www.research.att.com/∼njas/electrons/ ). Data are then converted to the sagittal plane (resulting a more complete visualization of the spinal cord than in the axial plane) using a simple matrix rotation. Sagittal DT imaging sequences are most sensitive to water diffusivity along the main spinal cord axis. To decrease cerebrospinal fluid partial flow motion, cardiac gating may be used, especially for thoracic cord imaging, but it is not mandatory if saturation pulses are placed in the region of the heart.
Based on these observations, we performed our studies using sagittal, single–shot, spin echo, echo-planar parallel GRAPPA DT imaging with an acceleration factor of 2 and 25 noncollinear gradient directions with two b values ( b = 0 and 500 s/mm 2 ) (field of view: 180 × 180 mm; image matrix: 128 × 128; 12 slices with a thickness of 3 mm, nominal voxel size: 1.4 × 1.4 × 3 mm, and TR/TE = 4600/83 ms). Acquisition time is slightly over 3 minutes per study, during which time the patients are asked to hold still and to try to avoid swallowing.
Other acquisition schemes exist (field of view: 200 mm; axial 2 mm slice thickness, and b value ∼ 800 s/mm 2 ), but to our knowledge they fail to generate adequate FT images needed to visualize the white matter tracts in the spinal cord (Metens and Balleriaud, personal communication, 2005).
Image analysis
Image analysis is performed on a voxel-by-voxel basis using our dedicated software (DPTools; http://www.fmritools.org ). Before performing the tensor estimation, an unwarping algorithm is applied to the DT imaging dataset to correct for distortions related to eddy currents induced by the large diffusion sensitizing gradients. This algorithm relies on a three-parameter distortion model including scale, shear, and linear translation in the phase-encoding direction . Optimal parameters are assessed independently for each slice relative to the corresponding T2-weighted image by maximization of an entropy-related similarity measure called “mutual information” . This algorithm has proven to be fast and reliable and is used in many brain applications. We adapted it to the spinal cord to decrease the distortions induced by the echo planar sequence. DT imaging acquisitions can be further processed without distortion corrections, but FA and FT maps may show artifacts in the edges of the field of view. After distortion correction, the diffusion tensor and subsequently the Eigen system (with Eigen values λ1, λ 2, λ 3 ) is calculated on a voxel-by-voxel basis as described in the literature . Thus, the DT imaging tensor field ( Fig. 1 ), the apparent diffusion coefficient (ADC) mean = (λ1 + λ 2 + λ 3 )/3 = λ, and the
FA = 3 2 ⋅ ( λ 1 − λ ) 2 + ( λ 2 − λ ) 2 + ( λ 3 − λ ) 2 λ 1 2 + λ 2 2 + λ 3 2
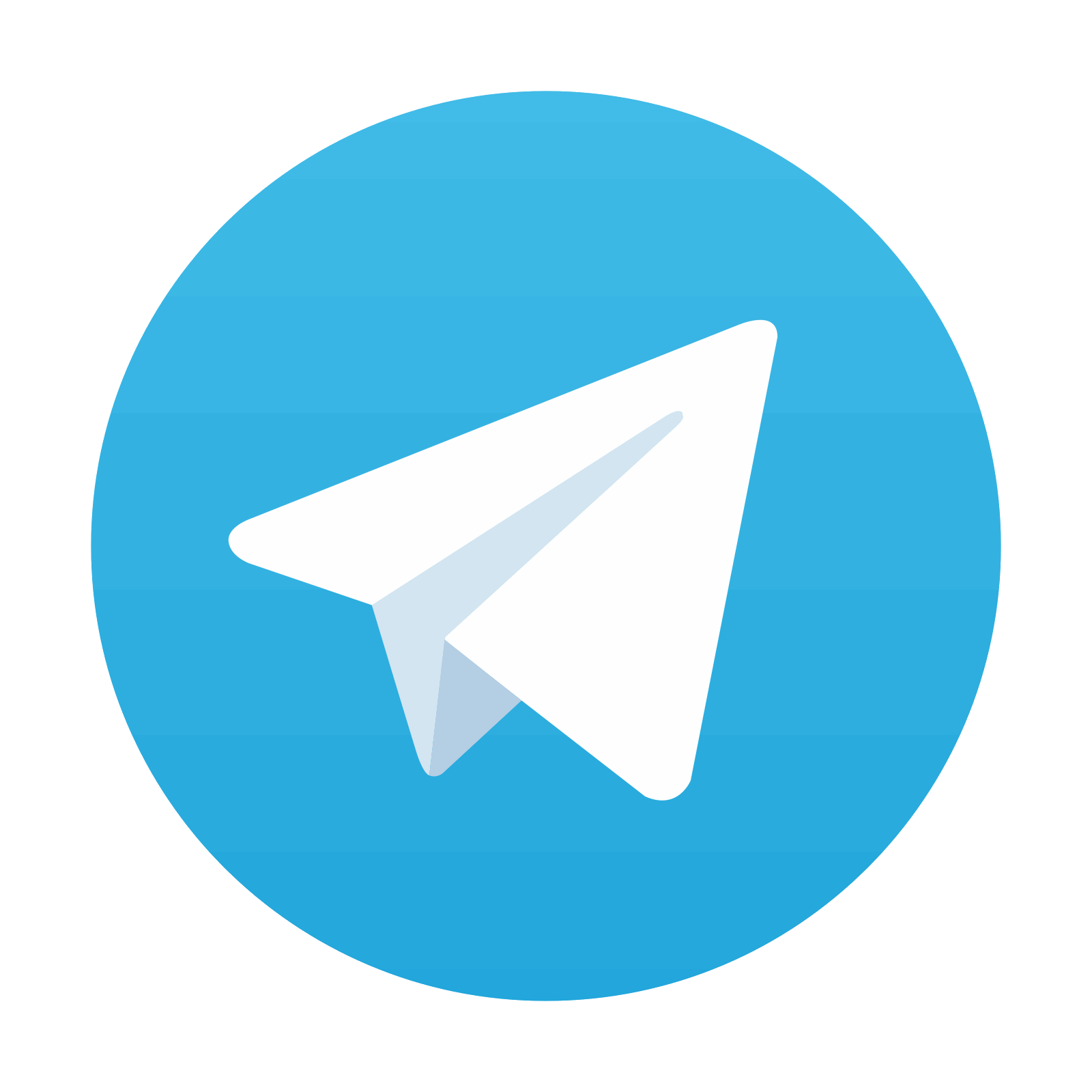
Stay updated, free articles. Join our Telegram channel

Full access? Get Clinical Tree
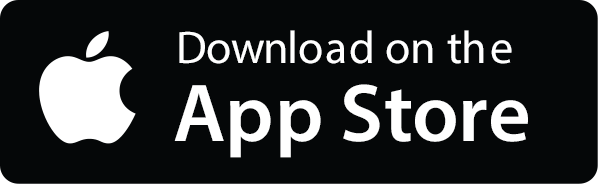
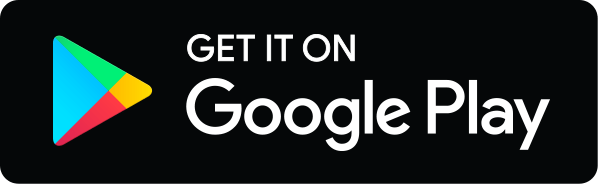