Diffusion-weighted imaging (DWI) has become an important tool in pediatric neuroradiology, helping in the evaluation of the encephalopathic and seizing neonate, and adding conspicuity, specificity, and prognostic value to the conventional magnetic resonance (MR) imaging data. DWI also facilitates understanding the pathophysiology and natural time course of ischemic and nonischemic disorders. When interpreted concurrently with the conventional MR imaging and other advanced MR imaging techniques, such as spectroscopy and arterial spin labeling, DWI can give clues leading to an accurate diagnosis and provide important information about pathophysiology and prognosis of the diseases, as well as guide adequate therapeutic modalities.
Diffusion-weighted imaging (DWI) has become an important tool in pediatric neuroradiology, helping in the evaluation of the encephalopathic and seizing neonate, and adding conspicuity, specificity, and prognostic value to the conventional magnetic resonance (MR) imaging data. DWI also facilitates understanding the pathophysiology and natural time course of ischemic and nonischemic disorders.
As myelination is incomplete in neonates, the detection of edema and the estimate of edema extent on T2-weighted imaging (T2-WI) is difficult. In addition, Fluid-attenuated inversion recovery (FLAIR) is insensitive to acute edema in the newborn brain, due to lack of myelination. DWI with corresponding apparent diffusion coefficient (ADC) maps provide the ability to differentiate edema associated with increased extracellular space and edema associated with metabolic compromise or evolving necrosis. The quantitative nature of ADC maps also provides the potential to detect regions of increased or decreased diffusivity if performed at b values where normal data are available.
Although transport of the acutely ill neonate to the MR imaging scanner can be challenging, it should be performed as soon as clinically possible to search for the presence, pattern, severity, and extent of injury. MR-compatible incubators with built-in coils have provided a means to reduce transport time and minimize transport risk. Incorporation of DWI into the standard MR imaging protocol adds only minutes, yet provides the potential for early detection of the presence and type of perinatal brain injury. This information is valuable to the clinical team as they attempt to determine if acute brain injury is the cause of the clinical symptoms and what type of injury has occurred. Although in most centers providing therapeutic hypothermia MR imaging is typically not performed until the neonate is stable on cooling to maximize therapeutic effectiveness, MR imaging as soon as stable for transport is still preferred. In these cases MR imaging with DWI provides valuable information about the presence of contraindications for therapeutic hypothermia, such as significant hemorrhage or venous thrombosis.
At pediatric centers axial DWI images are typically acquired with maximum b values ranging from 700 to 1000 s/mm 2 . The authors prefer a b value of at least 1000 to 1500 s/mm 2 because of the improved contrast to noise for regions with decreased ADC. Higher b values (∼3000 s/mm 2 ) may be useful for improved conspicuity of regions with decreased ADC, but myelinating regions have also become more conspicuous, so detection of very subtle injury requires knowledge of normal appearance. Moreover, as signal to noise decreases with increasing b value, these higher b values are most helpful on 3T systems with 32 channel coils.
As with all studies in which DWI is performed, ADC maps must be obtained and used in conjunction with DWI for optimal interpretation. This guideline holds particularly true for the immature brain, where there are normally large variations in ADC values caused by large regional differences in tissue water content. The ADC map is a direct reflection of the rate of water diffusion and is therefore not complicated by T2 contrast. Regions of low T2 due to early myelination can mask otherwise bright regions of decreased ADC on DWI, and regions of high T2 due to extracellular edema can appear bright on DWI, falsely appearing like regions of decreased ADC. Therefore both DWI and ADC maps must be used when evaluating the newborn brain.
Arterial hypoxic-ischemic injury
Despite the developments in modern medicine, perinatal brain injury remains a common cause of morbidity and mortality. In particular, neonatal hypoxic-ischemic injury (HII) is one of the most important causes of neurologic disabilities, accounting for 15% to 28% of children with cerebral palsy.
Global impairment in the exchange of respiratory gases occurring in the perinatal period causes deficit in oxygen supply, leading to neural tissue dysfunction and consequent hypoxic-ischemic encephalopathy in term and near-term neonates. Hypoxemia and mainly hypoperfusion are the two major pathogenic mechanisms. Because the neonatal brain is quite resistant to hypoxia in a setting of normal cerebral perfusion because of its ability to obtain energy from glucose and other substrates, most hypoxic ischemic brain injury likely results from a combination of both cerebral hypoxia and hypoperfusion. Although hypoxia and hypoperfusion are thought to be primary factors, there are additional vulnerability factors such as inflammation, oxidative stress, and excitotoxicity that are thought to play a role in determining the extent and severity of brain injury, with different regions of brain having different susceptibility to injury at different maturational stages. For example, developing oligodendrocytes were found to be more susceptible to oxidative stress toxicity than mature oligodendrocytes, due to a depletion of intracellular glutathione in the oligodendrocyte progenitor cells and preoligodendrocytes. These oligodendrocyte progenitor cells are also more vulnerable to glutamate excitotoxicity, because glutamate receptors are transiently expressed in this cell population but are downregulated in mature oligodendrocytes. Inflammatory response may be critically important in the genesis of brain injury in the term as well as preterm newborn, even though in this population it may play more of a modulating role. Previous studies have suggested that chorioamnionites might be considered an independent risk factor for brain damage in term as well as preterm infants.
Newborn HII may be associated with reperfusion before mitochondria are irreversibly damaged, preserving their ability to produce adenosine triphosphate (ATP). As a result, HII can trigger different pathways of cell death, both ATP-independent and ATP-dependent. First, if an HI event is severe and prolonged enough to cause critical energy reduction then failure of the ATP-dependent Na + /K + pump will occur, followed by NA + , Cl − , and water influx, cell swelling, and consequent immediate cell death by necrosis. Second, if an HI event is less severe with blood flow and oxygen restored rapidly enough that transient energy recovery occurs, necrotic cell death is delayed. Finally, if an HI event is even less severe with blood flow and oxygen restored rapidly enough that transient energy recovery occurs and necrosis is averted, delayed cell death may still occur but by apoptotic or autophagic mechanisms. Apoptosis occurs without significant inflammatory response, in contrast to cell necrosis in which strong inflammatory reaction usually occurs. Autophagy is another pathway for the cell affected by asphyxia by mediating either successful repair or cell suicide. After injury the cell may catabolize existing cytoplasmic components to maintain energy production, so that if reperfusion occurs the cell may survive. On the other hand, the cells can die if the apoptotic process cannot be interrupted. Mixed patterns of cell death can also occur. A cell that has initiated apoptotic program may not have the necessary energy to finish the apoptotic process or may be irrevocably injured by prolonged overactivation of autophagy, and when this happens the process may progress toward necrosis.
Immediate necrosis is associated with immediate ADC decrease and delayed necrosis is associated with delayed ADC decrease. The ADC signatures of apoptotic and autophagic mechanisms have not been well described, but are unlikely to cause large ADC decreases. Therefore, the magnitude of ADC decrease is likely affected by delays in initiation of necrotic cell death as well as by the proportion of necrotic, apoptotic, and normal cells. It must be noted that a normal DWI study does not exclude imminent necrotic cell death or ongoing apoptosis/autophagosis.
Most cases of HI encephalopathy are positive on DWI within the first day of life if therapeutic hypothermia has not been initiated. However, in milder cases or with therapeutic hypothermia, delays of at least 24 hours can occur before ADC decreases are evident or, if apoptosis dominates, ADC decreases may never occur. Therefore regions of decreased ADC may appear only after a few days and increase in volume over time as the injury evolves. Consequently, DWI performed in the first day of injury may be a poor predictor of the final extent of injury. A second MR image obtained between days 5 and 8 is beneficial in evaluating progression of injury. ADC pseudonormalization may occur around 7 to 10 days and could underestimate the extent; however, at this time abnormalities on conventional MR imaging sequences are typically amply evident.
Global Arterial Ischemic Injury in Term Neonates
Arterial ischemic injury can be global or focal. Global injuries are often associated with encephalopathy, and have two major patterns that can coexist: a profound or central pattern and a peripheral or partial pattern.
The central or profound pattern is usually related to a sentinel event, such as placental abruption, severe maternal hypotension, twin-twin transfusion, or cardiac failure, causing global profound hypoxia and ischemia for a short period of time and without time to establish collateral blood flow, resulting in damage to the most metabolically active zones. The affected regions on DWI correspond to zones of active myelination or high concentration of excitatory amino acids and corresponding receptors, therefore abnormalities can be observed in the ventrolateral thalamus, corticospinal tract in posterior limb internal capsule with or without involvement of the perirolandic cortex, other cortical areas, white matter, hippocampi, midbrain, dorsal brainstem, and superior vermis. Absent or less collateral blood flow in the basal ganglia, thalamus, and hippocampus make these regions more susceptible to injury when compared with cerebral cortex ( Fig. 1 ) .
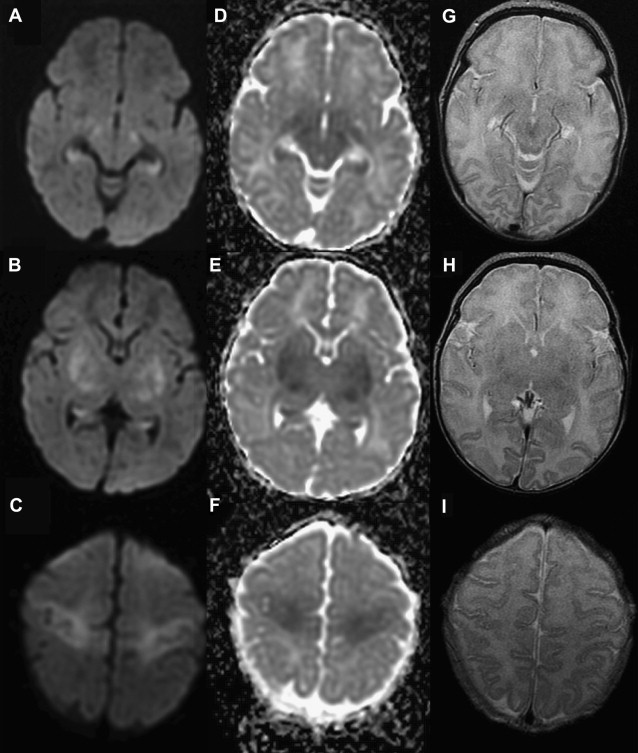
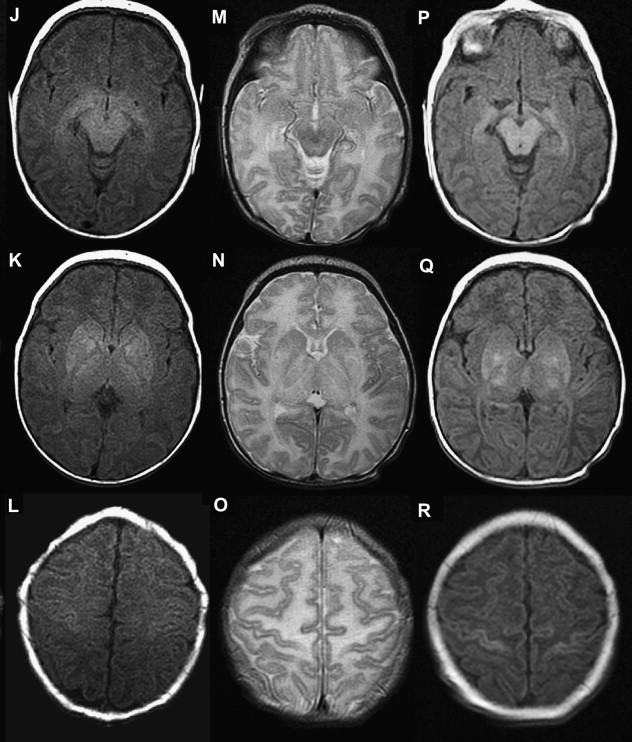
T1 shortening can be observed in areas of injury approximately 3 days after injury. However, subtle abnormalities may be difficult to detect because these areas correspond to the myelination zones with normal expected T1 shortening. T2 prolongation can also be seen in these areas with blurring of deep gray nuclei and cortical margins, but these findings are subtle and difficult to detect if one is unfamiliar with neonatal images. Abnormalities on DWI are seen earlier and are more conspicuous. Reduced diffusion may be evidenced on the first day and evolve over time because of delayed cell death, as stated earlier. Pseudonormalization on ADC maps occurs around 7 to 10 days, making diffusion imaging insensitive to recent injury after this time. Vermeulen and colleagues, studying 46 infants with neonatal HII, showed that low ADC values in the posterior limb of the internal capsule between 0 and 4 days of life were highly predictive of poor motor outcomes at 2 years of age. An increase in the lactate/choline ratio on MR spectroscopy on the first 72 hours after birth is also correlated with bad outcome. However, the authors’ group has seen several cases with no lactate in the first 24 to 48 hours that did not survive. In some of these cases arterial spin labeling images showed increased blood flow, which leads the authors to suspect that rebound hyperperfusion may correct the anaerobic metabolism but may not prevent delayed necrosis.
When extremely severe and prolonged hypoxia and ischemia occurs, diffuse damage to the brain with diffuse brain swelling and involvement of basal ganglia and thalami may take place, sparing only medulla oblongata and cerebellum. In this situation, where there is extensive supratentorial involvement, visual analyses of the DWI images can be challenging to the radiologist unfamiliar with normal studies, although the cerebellum, which is usually spared from the ischemia in term neonates, can be used as a reference.
When the hypoxia and/or hypoperfusion is less profound but lasts hours or days, continuously or intermittently, blood flow is redistributed to regions with lower energy demands, sparing the highly metabolically active zones involved in the profound pattern. The resulting DWI pattern of injury is a partial or peripheral pattern of injury with variable regions of cortex and subcortical white matter involved, but with cortex at the sulcal depths often preferentially involved. T1 shortening can also be observed in the depth of sulci on T1-WI beginning around day 3 and cortical increased T2 signal or blurring of cortical margins on T2-WI detected beginning sometime between the acute and subacute phase. DWI permits an earlier detection of injury, particularly in the first few days subsequent to injury ( Fig. 2 ) .
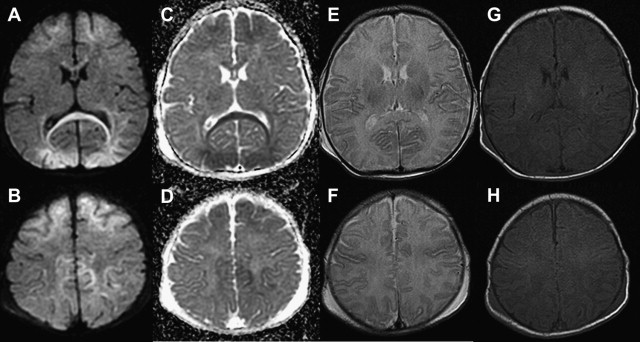
White matter injury can also occur in the absence of gray matter injury in term neonates. Li and colleagues described term neonates with mild encephalopathy and without congenital heart disease, with focal white matter injury associated with decreased diffusion on the third day of life suggesting perinatal injury. The investigators hypothesized that the lesions could be attributed to heterogeneous oligodendrocyte progenitor cell maturation, as white matter lesions were commonly recognized in “early” term neonates rather than low birth weight neonates. However, in the author’s experience similar lesions can also be seen in full-term infants in a pattern that suggests venous injury.
Encephalopathy of Prematurity
Advances in neonatal intensive care have increased the survival rates of preterm infants, including the ones with low birth weight. However, premature infants have a high incidence of cerebral palsy, cognitive impairment, visual loss, and epilepsy. Although these disabilities were first described as secondary to white matter injury, recent imaging studies have shown abnormalities consistent with both white matter injury and apparent neuronal/axonal disease. Thus, the term “encephalopathy of prematurity” has been found to be more accurate to describe the complexity of findings in the injured premature brain.
The pathophysiological basis of white matter injury on preterm newborns is not completely understood, although hypoperfusion and maternal infection or inflammation has been implicated in its development. In addition, vulnerability of specific cell populations, such as late oligodendrocyte progenitor cells and subplate neurons, and the complexity of developmental events make the immature white matter susceptible to damage.
White matter injury has both focal and diffuse components. Focal areas of microscopic necrosis involve pre-oligodendrocytes, axons, and perhaps late-migrating interneurons. This condition can evolve to cystic cavities in deep cerebral white matter around the ventricles. The diffuse component comprises mainly the pre-oligodendrocytes, leading to impaired myelination and consequent axonal deficiency. Along with the white matter injury, volume loss of the cerebral cortex, thalamus, basal ganglia, brainstem, and cerebellum is also observed, likely secondary to injury to subplate neurons, and retrograde and anterograde (transsynaptic) effects.
Signal abnormalities become evident first on DWI, being perceptible in the first week after birth. Both focal lesions and diffuse white matter decreases in diffusion have been reported. In one study, reduced diffusion progressed to increased diffusion in about the second to the fourth week of life and finally disappeared around the fourth month. Corresponding findings on conventional MR imaging are hyperintensity on T1-WI and hypointensity on T2-WI in the periventricular white matter. Susceptibility imaging may or may not show blooming indication that not all lesions are associated with hemorrhage.
The area of abnormal signal intensity seen on DWI is usually larger than the area of damage demonstrated on subsequent conventional MR imaging; however, Roelants-van Rijn and colleagues demonstrated histopathological white matter injury beyond the area of DWI signal abnormality, suggesting that both DWI and conventional MR imaging underestimate the real extent of damage. On follow-up MR imaging examination gliosis, white matter volume loss, callosal thinning, irregularity of ventricular walls, and ventricular dilatation can be recognized. MR imaging analyses can also demonstrate a decreased volume of neuronal structures as early as term-equivalent age, as well as later in childhood, adolescence, and adulthood.
Another common type of brain injury in premature infants is germinal matrix hemorrhage. Damage to the germinal matrix along the ventricular wall typically has its onset within 5 hours of birth. Hemorrhage is thought to be secondary to vascular pressure changes within the fragile germinal matrix capillaries. It can also be seen within the external granular layer of the cerebellum, which represents the cerebellar germinal zone. Cranial ultrasonography can be used to assess these lesions; however, gradient echo T2-WI or susceptibility-weighted images have higher sensitivity, and improve detection of cerebellar hemorrhage and periventricular venous hemorrhagic infarctions ( Fig. 3 ).
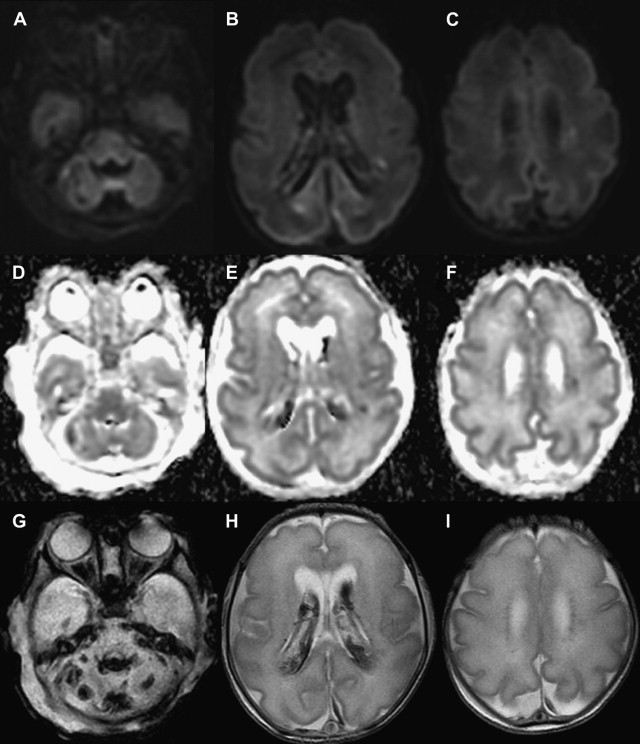
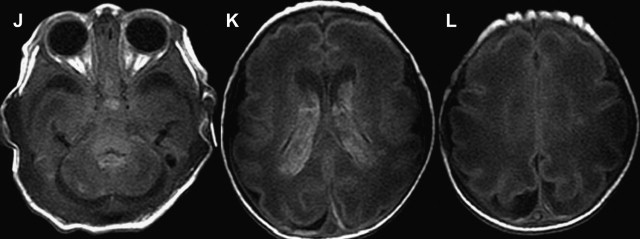
Arterial Ischemic Stroke
Arterial strokes are more common in term neonates and have an incidence of approximately 1 in 4000 deliveries. Focal arterial occlusion occurs in an otherwise normal brain, unlike global HII where the entire brain is exposed to hypoxia and ischemia. The origin of the arterial occlusion in unknown, but potential causes includes emboli, thrombosis, or transient spasm.
Early detection of neonatal ischemic stroke is challenging because these neonates typically appear healthy at birth and have normal Apgar scores. Moreover, clinical symptoms can be subtle, and typically present as symptomatic seizure around 48 hours after birth when cortical edema is already evident.
White matter and cortical hyperintensity on T2-WI result in the “missing cortex sign” in the first postnatal week. The cortex becomes hypointense during the second week while hyperintense signal persists in the white matter until 2 to 3 weeks. Reduced diffusion is observed on DWI when symptoms are evident, and is more marked during the first 4 days. ADC pseudonormalization takes places from the seventh day. Frequently the area of reduced diffusion tends to be larger than the area of signal abnormality seen on follow-up conventional MR imaging. This mismatch is likely due to early reperfusion when compared with adult stroke or later hypertrophy of adjacent cortical regions ( Fig. 4 ).
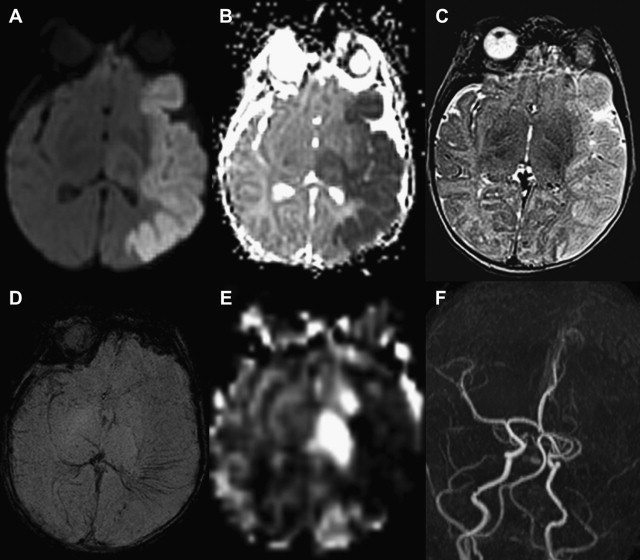
Sinovenous thrombosis
An important cause of neurologic morbidity in the neonatal period is the occurrence of sinovenous thrombosis. This condition is generally identified in neonates with history of maternal infection or corioamnionitis, and less regularly in the presence of congenital heart disease or prothrombotic disorders. Most of the infants present with seizures between 36 hours and 28 days of life, although apnea, hypotonia, and lethargy can also occur.
MR imaging may show diffuse parenchymal edema, intraventricular, subarachnoid, or intracerebral hemorrhage (can affect thalamus and caudate), ischemic injury with decreased diffusion on DWI, or signal abnormality in deep or superficial venous system. Intraluminal clot may be seen in the deep venous system but is rarely identified in the superficial system. MR venography can infrequently demonstrate decreased flow-related enhancement or abrupt change in caliber due to a superficial sinovenous thrombosis. However, changes in caliber of the superior sagittal sinus are most often caused by sutural diastasis at the lambdoid suture, a common sequela of delivery. The difficulty in finding an occlusion could be related to rapid dissolution of the clot or transient damage to the venous system resulting from mechanical forces during delivery. An important number of neonates that undergo neuroimaging studies present with small parturitional hemorrhage along the tentorium, which should not be misinterpreted as venous thrombosis.
DWI can exhibit reduced or increased diffusion, representing cytotoxic or vasogenic edema, respectively. Vasogenic edema occurs secondarily to minor blood-brain barrier breakdown in the setting of venous congestion, and usually resolves on follow-up examinations. Venous ischemic injuries show reduced diffusion in a venous drainage territory involving subcortical white matter that on follow-up, similar to arterial ischemic strokes, may undergo atrophy or almost completely resolve, likely depending on the severity and duration of the injury ( Fig. 5 ).
