FIGURE 10.40 Dislocation with radial head fracture. A: Intermediate-weighted fat-suppressed coronal image demonstrates a radial fracture (curved arrow). The complete disorganization of the medial and lateral soft tissues is suggestive of a fracture dislocation. B: T2-weighted fat-suppressed sagittal MR image confirms the persistent anterior displacement of the humeral head (asterisk) with respect to the coronoid tip (arrow). The bone marrow edema in the coronoid (arrow) may be the result of both impaction from the humerus as well as brachialis (arrowhead) injury.
FIGURE 10.41 Dislocation with radial head and coronoid fracture. A: T2-weighted fat-suppressed coronal MR image shows disruption of the radial (curved arrow) and ulnar (arrowheads) soft tissues. Edema is noted at the sublime tubercle (arrow). B: T2-weighted fat-suppressed axial MR image confirms the diagnosis of a radial head fracture (arrow). The posterior ulnar annular ligament attachment remains intact (curved arrow), although the posterolateral capsular extension (arrowhead) and the remainder of the capsule are thickened and edematous. C: Gradient sagittal MR image shows associated coronoid tip fracture (arrow), capitellar osteochondral lesion (double arrow), and anterior (arrowhead) and posterior (curved arrow) capsular insufficiency.
In the imaging assessment of a complex elbow dislocation, fractures of the radial head and coronoid should be noted and described in detail. Revisions of original classification systems for radial head fractures have been based on the degree of displacement of the articular surface and ability to internally fixate the lesion (66). Coronoid process fractures have been classified into three types: Type I, small lip fracture; Type II, fracture involves 50% or less of the coronoid; and Type III, fracture involves more than 50% of the coronoid (66, 84). More recently, a classification system based on anatomic location of fracture fragments has been introduced into the literature (Fig. 10.42). Fractures are classified as those that involve the tip (the fracture line does not extend medially past the sublime tubercle or into the coronoid body), anteromedial fragment (the fracture line exits the medial cortex in the anterior half of the sublime tubercle and laterally extends just medial to the tip of the coronoid), and base (involving the coronoid body with greater than 50% of the height). Identifying the anteromedial fracture is a key element of this classification system. Despite the small subtle radiographic presentation, they may predispose to rapid arthritis if left unreduced (56, 72).
Near-dislocation With Coronoid Fracture
Traumatic instability of the elbow may be a result of ligamentous injury in conjunction with fractures of the coronoid process (Fig. 10.43). This can occur in an isolated fashion or with concomitant olecranon fractures and has been termed varus posteromedial rotational instability. The mechanism of injury is believed to be a fall onto the outstretched hand that creates the combination of an axial load, varus stress, and a posteromedial rotational force on the elbow. These injuries are considered “near-dislocations” because the apposing surfaces of the humerus and ulna remain partially in contact.
Near-dislocation as Posterior Monteggia Lesion
Jupiter et al. (74) described a variant of the posterior Monteggia fracture pattern that included a posterior dislocation of the radial head and a proximal ulna fracture with an anterior triangular fracture fragment at the level of the coronoid process. The mechanism of injury was usually a low-energy fall in middle-aged and older women. Radial head and lateral collateral ligament complex disruption were common. Recognizing that the anterior coronoid fragment requires stable fixation appears to be critical in achieving an optimal outcome (85).
Near-dislocation as Transolecranon Fracture Dislocation
Transolecranon fracture–dislocation involves a comminuted proximal ulna/olecranon fracture with anterior subluxation or dislocation of the radiocapitellar joint, disruption of the ulnohumeral joint, and anterior displacement of the entire forearm with maintenance of the radioulnar relationship. This differs from the posterior Monteggia fracture in that the radius and ulna are both dislocated anteriorly and remain associated (73). This injury results from a high-energy blow to the dorsal aspect of the forearm with the elbow in midflexion. This injury is typically associated with large coronoid fractures, intact collateral ligaments, and a paucity of radial head fractures.
FIGURE 10.42 Ulnar fracture classification systems. A: Lateral and (B) frontal oblique vantage points of the elbow profile the three types of coronoid fracture introduced by Morrey and Regan: I, small lip fracture; II, 50% or less involvement of the coronoid; and III, greater than 50% involvement of the coronoid. C: O’Driscoll modified this classification: fractures that involve the tip, anteromedial fragment, and base. Identifying the anteromedial fracture is a key element of this classification system.
FIGURE 10.43 Near dislocation with coronoid fracture. Intermediate-weighted fat-suppressed sagittal MR images (A) through the ulna (B) and radius show the coronoid tip fracture with (curved arrow) the humerus (arrow) perched on the radius and ulna. The anterior and posterior capsule (arrowheads) are both insufficient. This is considered a near-dislocation as the articular surfaces still maintain contact.
Varus Instability
Varus instability is attributable to disruption of the lateral collateral ligament complex. The forces across the elbow are primarily valgus as a result of the anatomic alignment of the joint with the result that this articulation is rarely subjected to varus stress (Figs. 10.44 and 10.45). It is for this reason that isolated abnormalities of the lateral collateral ligament complex are not as well described as those on the medial side of the elbow (60). If it were to occur, the mechanism of injury would be a stress or force applied to the medial side of the articulation resulting in compression on that side with opening of the lateral articulation and subsequent insufficiency of the RCL. Because the RCL attaches on and is intimately associated with the annular ligament, an abnormality discovered in one of the structures obligates a careful inspection of the other.
FIGURE 10.44 Varus instability. Gradient coronal MR image in a patient diagnosed with varus instability shows a radial collateral ligament rupture (arrowhead) and a near full-thickness rupture of the common extensor tendon (arrow). The most proximal fibers of the common extensor tendon (curved arrow) appear stripped from the lateral epicondyle.
FIGURE 10.45 Varus instability. Gradient coronal MR image in a patient diagnosed with varus instability shows a complete rupture of the radial collateral ligament (arrowhead) as well as the common extensor tendon (curved arrow).
FIGURE 10.46 Annular ligament injury. T2-weighted fat-suppressed (A) coronal and (B) axial MR images show discontinuity of the posterior attachment of the annular ligament to the ulna (curved arrow). This results in an irregular, mass-like appearance to the retracted annular ligament (arrowhead).
Varus stress applied to the elbow may occur as an acute injury, but rarely as a repetitive stress. The exception to the latter clinical situation is the patient who uses the upper extremity as a weightbearing articulation such as patients with polio with chronic crutch use. Although an isolated lateral collateral ligament injury rarely occurs, complex injuries that include a varus component are much more commonly encountered and include dislocation, subluxation, or overly aggressive surgery (such as release of the common extensor tendon or radial head resection with unintentional ligament compromise).
FIGURE 10.47 Acute annular ligament injury. Proton density-weighted (A) axial MR image shows the focal discontinuity (arrow) of the posterior ulnar attachment of the annular ligament. B: More superior axial and (C) coronal MR images show an irregular mass-like appearance to the displaced annular ligament (arrows). D: Oblique coronal MR image shows the course of the lateral ulnar collateral ligament (arrowheads) tobenormal.
Few studies describe the imaging findings of injuries of the radial collateral or annular ligament. Imaging principles used to diagnose and characterize partial- and full-thickness tears of the UCL complex are also applied to the radial-sided ligaments. With regard to the annular ligament, however, lateral snapping of the elbow may arise from a lesion in this structure. Wrightman (86) described a torn annular ligament that was displaced into the radiocapitellar joint, causing a visible click in the lateral elbow. The author observed that when the elbow was extended, tightening of the capsule tended to pull the annular ligament proximally, causing the separated band to slip over the radial head. In a recent case, a similar clinical presentation was described. Findings of a torn ligamentous band of the annular ligament were confirmed intraoperatively with the band slipping over the radial head with forearm extension (51). In our practice, we have encountered several such cases with the clinical presentation of posteolateral pain in the acute setting and a snapping sensation in the more chronic setting (Figs. 10.46 and 10.47).
Anterior Instability
Anterior instability of the elbow is rare and typically seen in association with fractures of the olecranon (60, 87). The mechanism of injury has most often been described as a direct blow to the posterior aspect of the forearm in the flexed position (88). Other mechanisms include severe twisting of the forearm and a fall on the outstretched hand with hyperextension of the elbow. (88) Various factors such as laxity of the capsule and ligaments and malformation of the olecranon, trochlea, and radial head have been proposed to explain this dislocation. They are often associated with severe soft tissue disruption such as avulsion of the triceps insertion, rupture of the brachial artery, and injury to the ulnar nerve (88).
References
1. Morrey BF, An KN. Functional anatomy of the ligaments of the elbow. Clin Orthop Relat Res. 1985;201:84–90.
2. O’Driscoll SW, Jaloszynski R, Morrey BF, et al. Origin of the medial ulnar collateral ligament. J Hand Surg (Am). 1992;17: 164–168.
3. Nakanishi K, Masatomi T, Ochi T, et al. MR arthrography of elbow: evaluation of the ulnar collateral ligament of elbow. Skeletal Radiol. 1996;25:629–634.
4. Timmerman LA, Andrews JR. Histology and arthroscopic anatomy of the ulnar collateral ligament of the elbow. Am J Sports Med. 1994;22:667–673.
5. Munshi M, Pretterklieber ML, Chung CB, et al. Anterior bundle of ulnar collateral ligament: evaluation of anatomic relationships by using MR imaging, MR arthrography, and gross anatomic and histologic analysis. Radiology. 2004;231:797–803.
6. Timmerman LA, Andrews JR. Undersurface tear of the ulnar collateral ligament in baseball players. A newly recognized lesion. Am J Sports Med. 1994;22 33–36.
7. Mallisee TA, Boynton MD, Erickson SJ, et al. Normal MR imaging anatomy of the elbow. Magn Reson Imaging Clin N Am. 1997;5:451–479.
8. Beckett KS, McConnell P, Lagopoulos M, et al. Variations in the normal anatomy of the collateral ligaments of the human elbow joint. J Anat. 2000;197:507–511.
9. Mirowitz SA, London SL. Ulnar collateral ligament injury in baseball pitchers: MR imaging evaluation. Radiology. 1992;185:573–576.
10. Beltran J, Noto AM, Herman LJ, et al. Tendons: high-field-strength, surface coil MR imaging. Radiology. 1987;162:735–740.
11. Patten RM. Overuse syndromes and injuries involving the elbow: MR imaging findings. AJR Am J Roentgenol. 1995;164: 1205–1211.
12. Murphy BJ. MR imaging of the elbow. Radiology. 1992;184:525–529.
13. Carrino JA, Morrison WB, Zou KH, et al. Noncontrast MR imaging and MR arthrography of the ulnar collateral ligament of the elbow: prospective evaluation of two-dimensional pulse sequences for detection of complete tears. Skeletal Radiol. 2001;30:625–632.
14. Sonin AH, Fitzgerald SW. MR imaging of sports injuries in the adult elbow: a tailored approach. AJR Am J Roentgenol. 1996; 167:325–331.
15. Timmerman LA, Schwartz ML, Andrews JR. Preoperative evaluation of the ulnar collateral ligament by magnetic resonance imaging and computed tomography arthrography. Evaluation in 25 baseball players with surgical confirmation. Am J Sports Med. 1994;22:26–31; discussion 32.
16. Neviaser TJ. The anterior labroligamentous periosteal sleeve avulsion lesion: a cause of anterior instability of the shoulder. Arthroscopy. 1993;9:17–21.
17. Schwartz ML, al-Zahrani S, Morwessel RM, et al. Ulnar collateral ligament injury in the throwing athlete: evaluation with saline-enhanced MR arthrography. Radiology. 1995;197:297–299.
18. Carrino JA, Morrison WB, Zou KH, et al. Lateral ulnar collateral ligament of the elbow: optimization of evaluation with two-dimensional MR imaging. Radiology. 2001;218:118–125.
19. Sugimoto H, Hyodoh K, Shinozaki T. Throwing injury of the elbow: assessment with gradient three-dimensional, Fourier transform gradient-echo and short tau inversion recovery images. J Magn Reson Imaging. 1998;8:487–492.
20. Cotten A, Jacobson J, Brossmann J, et al. MR arthrography of the elbow: normal anatomy and diagnostic pitfalls. J Comput Assist Tomogr. 1997;21:516–522.
21. Cotten A, Jacobson J, Brossmann J, et al. Collateral ligaments of the elbow: conventional MR imaging and MR arthrography with coronal oblique plane and elbow flexion. Radiology. 1997; 204:806–812.
22. Cotten A, Boutin RD, Resnick D. Normal anatomy of the elbow on conventional MR imaging and MR arthrography. Semin Musculoskelet Radiol. 1998;2:133–140.
23. Jacobson JA, Propeck T, Jamadar DA, et al. US of the anterior bundle of the ulnar collateral ligament: findings in five cadaver elbows with MR arthrographic and anatomic comparison—initial observations. Radiology. 2003;227:561–566.
24. Kuroda S, Sakamaki K. Ulnar collateral ligament tears of the elbow joint. Clin Orthop Relat Res. 1986;208:266–271.
25. Salvo JP, Rizio L 3rd, Zvijac JE, et al. Avulsion fracture of the ulnar sublime tubercle in overhead throwing athletes. Am J Sports Med. 2002;30:426–431.
26. David TS. Medial elbow pain in the throwing athlete. Orthopedics. 2003;26:94–103; quiz 104–105.
27. Field LD, Savoie FH. Common elbow injuries in sport. Sports Med. 1998;26:193–205.
28. Hyman J, Breazeale NM, Altchek DW. Valgus instability of the elbow in athletes. Clin Sports Med. 2001;20:25–45, viii.
29. Safran MR. Elbow injuries in athletes. A review. Clin Orthop Relat Res. 1995;310:257–277.
30. Frostick SP, Mohammad M, Ritchie DA. Sport injuries of the elbow. Br J Sports Med. 1999;33:301–311.
31. Eygendaal D, Heijboer MP, Obermann WR, et al. Medial instability of the elbow: findings on valgus load radiography and MRI in 16 athletes. Acta Orthop Scand. 2000;71:480–483.
32. Safran MR, Graham SM. Distal biceps tendon ruptures: incidence, demographics, and the effect of smoking. Clin Orthop Relat Res. 2002;404:275–283.
33. Hang YS, Lippert FG 3rd, Spolek GA, et al. Biomechanical study of the pitching elbow. Int Orthop. 1979;3:217–223.
34. Miller CD, Savoie FH 3rd. Valgus extension injuries of the elbow in the throwing athlete. J Am Acad Orthop Surg. 1994;2:261–269.
35. Werner SL, Fleisig GS, Dillman CJ, et al. Biomechanics of the elbow during baseball pitching. J Orthop Sports Phys Ther. 1993;17:274–278.
36. Conway JE, Jobe FW, Glousman RE, et al. Medial instability of the elbow in throwing athletes. Treatment by repair or reconstruction of the ulnar collateral ligament. J Bone Joint Surg Am. 1992;74:67–83.
37. Chen FS, Rokito AS, Jobe FW. Medial elbow problems in the overhead-throwing athlete. J Am Acad Orthop Surg. 2001;9: 99–113.
38. Wilson FD, Andrews JR, Blackburn TA, et al. Valgus extension overload in the pitching elbow. Am J Sports Med. 1983;11: 83–88.
39. Mulligan SA, Schwartz ML, Broussard MF, et al. Heterotopic calcification and tears of the ulnar collateral ligament: radiographic and MR imaging findings. AJR Am J Roentgenol. 2000;175:1099–1102.
40. Morrey BF, Tanaka S, An KN. Valgus stability of the elbow. A definition of primary and secondary constraints. Clin Orthop Relat Res. 1991;265:187–195.
41. Miller TT. Imaging of elbow disorders. Orthop Clin North Am. 1999;30:21–36.
42. Rijke AM. MR imaging versus stress radiography in the evaluation of chronic ankle instability. Radiology. 1995;196:580–581.
43. Glousman RE. Ulnar nerve problems in the athlete’s elbow. Clin Sports Med. 1990;9:365–377.
44. Kooima CL, Anderson K, Craig JV, et al. Evidence of subclinical medial collateral ligament injury and posteromedial impingement in professional baseball players. Am J Sports Med. 2004; 32:1602–1606.
45. Nuber GW, Diment MT. Olecranon stress fractures in throwers. A report of two cases and a review of the literature. Clin Orthop Relat Res. 1992;278:58–61.
46. Rettig AC, Wurth TR, Mieling P. Nonunion of olecranon stress fractures in adolescent baseball pitchers: a case series of 5 athletes. Am J Sports Med. 2006;34:653–656.
47. Ahmad CS, Park MC, Elattrache NS. Elbow medial ulnar collateral ligament insufficiency alters posteromedial olecranon contact. Am J Sports Med. 2004;32:1607–1612.
48. Yamamoto Y, Ishibashi Y, Tsuda E, et al. Osteochondral autograft transplantation for osteochondritis dissecans of the elbow in juvenile baseball players: minimum 2-year follow-up. Am J Sports Med. 2006;34:714–720.
49. Cain EL Jr, Dugas JR, Wolf RS, et al. Elbow injuries in throwing athletes: a current concepts review. Am J Sports Med. 2003;31:621–635.
50. Dunning CE, Zarzour ZD, Patterson SD, et al. Ligamentous stabilizers against posterolateral rotatory instability of the elbow. J Bone Joint Surg Am. 2001;83:1823–1828.
51. Huang GS, Lee CH, Lee HS, et al. MRI, arthroscopy, and histologic observations of an annular ligament causing painful snapping of the elbow joint. AJR Am J Roentgenol. 2005;185: 397–399.
52. Fowler KA, Chung CB. Normal MR imaging anatomy of the elbow. Magn Reson Imaging Clin N Am. 2004;12:191–206.
53. Potter HG, Weiland AJ, Schatz JA, et al. Posterolateral rotatory instability of the elbow: usefulness of MR imaging in diagnosis. Radiology. 1997;204:185–189.
54. Terada N, Yamada H, Toyama Y. The appearance of the lateral ulnar collateral ligament on magnetic resonance imaging. J Shoulder Elbow Surg. 2004;13:214–216.
55. Grafe MW, McAdams TR, Beaulieu CF, et al. Magnetic resonance imaging in diagnosis of chronic posterolateral rotatory instability of the elbow. Am J Orthop. 2003;32:501–503; discussion 504.
56. O’Driscoll SW, Bell DF, Morrey BF. Posterolateral rotatory instability of the elbow. J Bone Joint Surg Am. 1991;73:440–446.
57. O’Driscoll SW, Morrey BF, Korinek S, et al. Elbow subluxation and dislocation. A spectrum of instability. Clin Orthop Relat Res. 1992;280:186–197.
58. O’Driscoll SW. Elbow instability. Hand Clin. 1994;10:405–415.
59. O’Driscoll SW. Elbow instability. Acta Orthop Belg. 1999;65: 404–415.
60. O’Driscoll SW. Classification and evaluation of recurrent instability of the elbow. Clin Orthop Relat Res. 2000;370:34–43.
61. O’Driscoll SW, Jupiter JB, King GJ, et al. The unstable elbow. Instr Course Lect. 2001;50:89–102.
62. O’Driscoll SW, Spinner RJ, McKee MD, et al. Tardy posterolateral rotatory instability of the elbow due to cubitus varus. J Bone Joint Surg Am. 2001;83:1358–1369.
63. O’Driscoll SW. Stress radiographs are important in diagnosing valgus instability of the elbow. J Bone Joint Surg Am. 2002;84:686; author reply 686–687.
64. Bredella MA, Tirman PF, Fritz RC, et al. MR imaging findings of lateral ulnar collateral ligament abnormalities in patients with lateral epicondylitis. AJR Am J Roentgenol. 1999;173: 1379–1382.
65. Rodgers WB, Kharrazi FD, Waters PM, et al. The use of osseous suture anchors in the treatment of severe, complicated elbow dislocations. Am J Orthop. 1996;25:794–798.
66. Phillips CS, Segalman KA. Diagnosis and treatment of post-traumatic medial and lateral elbow ligament incompetence. Hand Clin. 2002;18:149–159.
67. Ring D, Jupiter JB. Fracture–dislocation of the elbow. J Bone Joint Surg Am. 1998;80:566–580.
68. Josefsson PO, Gentz CF, Johnell O, et al. Dislocations of the elbow and intraarticular fractures. Clin Orthop Relat Res. 1989;246:126–130.
69. Broberg MA, Morrey BF. Results of treatment of fracture–dislocations of the elbow. Clin Orthop Relat Res. 1987;216:109–119.
70. Ring D, Jupiter JB. Fracture–dislocation of the elbow. Hand Clin. 2002;18:55–63.
71. Biga N, Thomine JM. Transolecranal dislocations of the elbow [in French]. Rev Chir Orthop Reparatrice Appar Mot. 1974;60: 557–567.
72. Tashjian RZ, Katarincic JA. Complex elbow instability. J Am Acad Orthop Surg. 2006;14:278–286.
73. Ring D, Jupiter JB, Sanders RW, et al. Transolecranon fracture–dislocation of the elbow. J Orthop Trauma. 1997;11:545–550.
74. Jupiter JB, Leibovic SJ, Ribbans W, et al. The posterior Monteggia lesion. J Orthop Trauma. 1991;5:395–402.
75. Hotchkiss RN. Displaced fractures of the radial head: internal fixation or excision? J Am Acad Orthop Surg. 1997;5:1–10.
76. Mason ML. Some observations on fractures of the head of the radius with a review of one hundred cases. Br J Surg. 1954;42:123–132.
77. Broberg MA, Morrey BF. Results of delayed excision of the radial head after fracture. J Bone Joint Surg Am. 1986;68:669–674.
78. Herbertsson P, Josefsson PO, Hasserius R, et al. Uncomplicated Mason type-II and III fractures of the radial head and neck in adults. A long-term follow-up study. J Bone Joint Surg Am. 2004;86:569–574.
79. Gupta GG, Lucas G, Hahn DL. Biomechanical and computer analysis of radial head prostheses. J Shoulder Elbow Surg. 1997;6:37–48.
80. Pribyl CR, Kester MA, Cook SD, et al. The effect of the radial head and prosthetic radial head replacement on resisting valgus stress at the elbow. Orthopedics. 1986;9:723–726.
81. Josefsson PO, Gentz CF, Johnell O, et al. Dislocations of the elbow and intraarticular fractures. Clin Orthop Relat Res. 1989;246:126–130.
82. Ring D, Jupiter JB, Zilberfarb J. Posterior dislocation of the elbow with fractures of the radial head and coronoid. J Bone Joint Surg Am. 2002;84:547–551.
83. Pugh DM, Wild LM, Schemitsch EH, et al. Standard surgical protocol to treat elbow dislocations with radial head and coronoid fractures. J Bone Joint Surg Am. 2004;86:1122–1130.
84. Regan W, Morrey B. Fractures of the coronoid process of the ulna. J Bone Joint Surg Am. 1989;71:1348–1354.
85. Ring D, Jupiter JB, Simpson NS. Monteggia fractures in adults. J Bone Joint Surg Am. 1998;80:1733–1744.
86. Wrightman J. Clicking elbow from a torn annular ligament: report of a case. J Bone Joint Surg Br. 1963;45:380–381.
87. Torchia ME, DiGiovine NM. Anterior dislocation of the elbow in an arm wrestler. J Shoulder Elbow Surg. 1998;7: 539–541.
88. Saouti R, Albassir A, Berger JP, et al. Anterior elbow dislocation with recurrent instability. Acta Orthop Belg. 2003;69:197–200.
The classification of tendon injuries about the elbow can be organized by location, acuity, and degree of injury. Tendon injury related to a single isolated event is uncommon, although exceptions to this rule do occur. More commonly, tendinous injuries in this location relate to chronic repetitive microtrauma. MRI is particularly well suited, with its excellent soft tissue contrast, to diagnose tendon pathology. This is done primarily by close inspection of signal intensity and morphology of the tendons.
The many muscles and associated tendons of the elbow lend themselves to division into four anatomic regions: posterior, anterior, medial, and lateral. The posterior group includes the triceps and anconeus. The muscles of the anterior group are the biceps brachii and brachialis. The muscles in the medial group are the pronator teres, the palmaris longus, and the flexors of the hand and wrist emanating primarily from the common flexor tendon. The muscles in the lateral group include the supinator, brachioradialis, and extensor muscles of the hand and wrist, the latter arising primarily from the common extensor tendon. Specific anatomic considerations, tendon pathology commonly encountered in the elbow as well as MRI diagnostic criteria are addressed.
Muscle and Tendon Anatomy
Organization of the complex muscular anatomy about the elbow lends itself to division into anterior, posterior, medial, and lateral groups. Although this method allows delineation of the specific muscles and their respective tendons about the elbow, it is important to emphasize that the common flexor and common extensor tendons are involved in the vast majority of musculotendinous pathology about the elbow, thus obviating the need for localizing pathology to a single muscle. To this end, the oblique coronal imaging plane provides optimal visualization of both the common flexor and common extensor tendons, underscoring the importance of obtaining high signal-to-noise ratio fluid-sensitive images in the coronal plane.
The lateral muscle group can be thought of consisting of three components: a superficial group, the common extensors, and the supinator. The superficial group includes the brachioradialis and extensor carpi radialis longus (ECRL). Together with the extensor carpi radialis brevis, the superficial group forms a bulky muscular mass termed the mobile wad, which surrounds much of the anterolateral aspect of the elbow (Fig. 11.1). The brachioradialis forms the most anterior portion of the lateral muscle group, arises from the supracondylar ridge of the humerus, and inserts near the radial styloid process. The extensor carpi radialis longus originates from the supracondylar ridge just distal to the brachioradialis and inserts distally at the base of the second metacarpal.
The common extensor group is composed of four muscles: the extensor carpi radialis brevis (ECRB), extensor digitorum, extensor digiti minimi, and extensor carpi ulnaris (Fig. 11.2). The common extensors originate from the lateral epicondyle through the common extensor tendon and are found along the posterolateral aspect of the elbow blending with the more substantial superficial muscle group. The most lateral component of the common extensor tendon is the ECRB, which lies immediately deep to the ECRL. The radiohumeral bursa is an adventitial bursa deep to the ECRB, which may distend in cases of epicondylitis, there by separating the lateral collateral ligament (LCL) and ECRB (1).
The common extensor tendon is seen as a hypointense band arising from the lateral epicondyle on MRI (Fig. 11.3). The ECRB and LCL complex are closely applied to each other and may not be resolved as discrete structures. Some authors suggest that the anatomic origin of the ECRB has contributions from the common extensor tendon, LCL complex, overlying fascia, and intramuscular septum (2, 3). The tendinous origins of the extensor carpi radialis longus and brevis can be distinguished from one another with the ECRL slightly superficial to the ECRB with intervening intermediate signal between the two tendons (Fig. 11.4). The ECRB is the initial site of signal abnormalities in cases of tennis elbow (1). Given the close proximity of the ECRB and lateral ulnar collateral ligament (LUCL), it is not surprising that severe lateral epicondylitis is associated with secondary abnormalities of the LUCL on MRI (4).
The supinator, the deepest of the lateral muscle group, arises from both the lateral epicondyle and the supinator crest of the ulna, inserting distally on the radial shaft, enveloping much of the proximal radius along its course. The muscle typically has thin superficial and bulky deep components (Fig. 11.5). The posterior interosseous nerve innervates the supinator and travels between the deep and superficial portions of the muscle.
The medial muscle group includes the pronator teres and four superficial flexors: the flexor carpi radialis (FCR), palmaris longus (PL), flexor carpi ulnaris (FCU), and flexor digitorum superficialis (FDS) (Figs. 11.6 and 11.7). The pronator teres forms most of the anteromedial muscle mass at the level of the elbow. The bulk of the pronator teres arises from a large humeral head proximal to the medial epicondyle. A smaller ulnar head of pronator teres arises from the medial aspect of the coronoid process. The pronator teres proceeds distally to insert on the lateral aspect of the radial shaft.
FIGURE 11.1 Lateral muscle and tendon anatomy. The superficial lateral muscle group consists of the brachioradialis and extensor carpi radialis longus. Together with the extensor carpi radialis, the superficial group forms the muscle mass referred to as the mobile wad. The common extensor group is composed of the extensor carpi radialis brevis, extensor digitorum, extensor digiti minimi, and extensor carpi ulnaris.
FIGURE 11.2 Common extensor tendon anatomy. The common extensor group originates from the lateral epicondyle through the common extensor tendon. The extensor carpi radialis brevis also has a significant muscular attachment to the humerus that blends with the more superficial extensor carpi radialis longus.
The common flexor tendon arises from the medial epicondyle and includes the FCR, PL, FCU, FDS (humeroulnar head), and a portion of the pronator teres (Fig. 11.8). The common flexor muscles at the level of the elbow form a confluent muscular mass at the posteromedial aspect of the joint. On oblique coronal MR images, the common flexor tendon appears as a hypointense band that gradually blends with proximal fibers of the ulnar collateral ligament arising from the undersurface of the medial epicondyle (Fig. 11.9). The palmaris longus, which blends with the posteromedial border of pronator teres, may be absent in up to 13% of cases (5). The FDS arises from two heads, a humeroulnar head arising from the common flexor tendon and a radial head arising from the anterior radius. A fibrous arch is formed where the two heads of the FDS coalesce, beneath which passes the anterior interosseous branch of the median nerve. The FCU also arises from two heads near the cubital tunnel. The first head is the most medial portion of the common flexor tendon and the second head from the medial aspect of the olecranon. A fibrous arch between the two heads forms the roof of the cubital tunnel and has been variously termed the arcuate ligament, Osborne’s band, and the cubital tunnel retinaculum.
The deep flexors include the flexor digitorum profundus and the flexor pollicis longus (FPL). Thisgroup originates from the proximal ulna, just distal to the cubital tunnel, thus forming a small muscular mass as the posteromedial aspect of the ulna. Gantzer’s muscle, an accessory slip of the FPL, may be encountered in 45% of individuals and rarely causes impingement of the median nerve (6, 7).
FIGURE 11.3 Common extensor tendon. A: T1-weighted coronal MR image and B: corresponding specimen photograph show the proximal attachment of the common extensor tendon (straight arrow) arising from the undersurface of the lateral epicondyle. The radial collateral ligament (curved arrow) is closely apposed to its undersurface.
FIGURE 11.4 Components of the common extensor tendon. A: T1-weighted sagittal MR image and B: corresponding specimen photograph show the relationship of the extensor carpi radialis longus (arrowheads) to the components of the common extensor tendon: extensor carpi radialis brevis (straight arrow), extensor digitorum (thin arrow), extensor digiti minimi (angled arrow), and extensor carpi ulnaris (curved arrow). The narrow lowsignal–intensity bands that extend distally to the forearm from the separate muscular contributions represent intermuscular septa that serve as aponeuroses for the individual muscles.
FIGURE 11.5 Supinator muscle. A: T1-weighted axial MR image and B: corresponding specimen photograph demonstrate its thin superficial (arrowheads) and bulky deep (arrows) components with the interposed posterior interosseous nerve (thin arrow).
FIGURE 11.6 Medial muscle and tendon anatomy. The medial muscle group includes the pronator teres and four superficial flexors.
The anterior muscle group includes the two primary flexors of the elbow, the biceps brachii and brachialis. The brachialis arises from the distal humerus and inserts at the ulnar tuberosity. The biceps brachii muscle arises from long and short heads and terminates in a single insertion at the radial tuberosity. The short head arises from the coracoid process and the long head from the supraglenoid tubercle of the scapula. The distal biceps tendon is formed from the two muscle bellies, which unite approximately 7 cm proximal to the elbow. Like the Achilles tendon, the distal biceps tendon has no tendon sheath. The distal portion of the biceps tendon may be at risk for attritional changes and tearing in a fashion similar to the supraspinatus tendon of the shoulder in cases of impingement (8). A relative hypovascular zone has been described in the distal tendon subject to mechanical impingement between the radial tuberosity and ulna (9). The bicipital aponeurosis, or lacertus fibrosis, arises from the distal biceps tendon and passes medially to blend with the fascia covering the flexor–pronator mass of the medial aspect of the elbow (Figs. 11.10 and 11.11). This fibrous band may prevent proximal retraction of the complete biceps rupture, there by masking the typical clinical findings of a balled-up muscular mass.
Axial images generally provide the best visualization of the biceps tendon as it inserts on the radial tuberosity. Sagittal images of biceps may help confirm suspect pathology. Fluid distension of the bicipitoradial bursa can be easily seen on all three standard imaging planes, although axial images best illustrate the close relation of the bursa and biceps tendon.
The anconeus and triceps form the posterior muscle group. The triceps arises from three heads: the lateral head from the posterolateral proximal humerus, the long head from the infraglenoid tubercle of the scapula, and the medial head from the posterior distal humerus. These form a common tendon that inserts at the olecranon, which normally may have a striated appearance (10). Rarely, a portion of the medial triceps may insert on the medial epicondyle and compress the ulnar nerve (11). The medial head of the triceps may subluxate, causing a snapping sensation similar to that of ulnar nerve subluxation (12). The anconeus arises from the posterior margin of the lateral epicondyle and courses medially to insert on the lateral margin of the olecranon. The role of the anconeus is somewhat controversial, although it is thought to help stabilize the elbow. The triceps insertion is clearly seen on sagittal and axial MR images (Fig. 11.12). The anconeus is easily seen on axial images, serving as a useful landmark for the lateral aspect of the elbow.
The anconeus epitrochlearis is an anomalous muscle found to occur in 11% of anatomic specimens that may cause cubital tunnel syndrome (13). In addition, there have been reports of symptomatic muscle edema in this accessory muscle as an additional cause of medial elbow pain (14). The anconeus epitrochlearis arises from the medial humeral condyle, passes superficial to the ulnar nerve, and inserts on the olecranon (Fig. 11.13).
FIGURE 11.7 Medial muscle anatomy. A: T1-weighted axial MR image and B: corresponding specimen photograph profile components of the medial muscle group: flexor carpi ulnaris (arrowheads), flexor digitorum superficialis (arrow), flexor carpi radialis (curved arrow), and pronator teres (angled arrow). There is no palmaris longus muscle as is noted in 13% of the population.
FIGURE 11.8 Common flexor tendon anatomy. The common flexor tendon arises from the medial epicondyle and includes contributions from the flexor carpi radialis, palmaris longus, flexor carpi ulnaris, humeroulnar head of the flexor digitorum superficialis, and a portion of the pronator teres.
FIGURE 11.9 Common flexor tendon. A: T1-weighted coronal MR image and B: corresponding specimen photograph show the proximal attachment of the common flexor tendon (straight arrow) arising from the undersurface of the medial epicondyle. The ulnar collateral ligament (curved arrow) is closely apposed to its undersurface.
Tendon Lesion Classification and Imaging Characterization
Like elsewhere in the body, the tendons about the elbow should be smooth, linear structures of low signal intensity. The distinction between tendinosis and tendon tear with MRI can be challenging. Abnormal morphology (attenuation or thickening) can be seen in tendinosis or tear. It is the presence of increased signal intensity within the substance of a tendon, that which parallels simple fluid on a fluid-sensitive or long TE sequence, that heralds the presence of a tear and allows the distinction between tendinosis and tendon tear. Increased signal intensity within a tendon that does not brighten to the level of fluid on fluid-sensitive sequences, rather, is classified as tendinosis (Fig. 11.14). With regard to the short TE sequences, increased signal intensity can be identified in both tendinosis and tear. An alternative explanation for increased intratendon signal intensity with short echo times is the “magic angle” phenomenon encountered when tendons are placed at an angle of 45 to 65 degrees to the static magnetic field. In the fully extended elbow, however, the angle of the tendons just beyond their origins from the humeral epicondyles is much less than 45 degrees, lessening the effect of this phenomenon significantly (15).
FIGURE 11.10 Lacertus fibrosis. The lacertus fibrosus, also called the bicipital aponeurosis, arises from the distal biceps tendon and passes medially to blend with the fascial covering of the flexor–pronator group.
FIGURE 11.11 Lacertus fibrosis. T1-weighted axial MR image shows the biceps tendon (curved arrow) within the antecubital fossa en route to its ultimate attachment on the radial tuberosity. The lacertus fibrosis (arrowheads) is seen extending from the tendon to the flexor–pronator muscle group.
Pathologic lesions within tendons should be carefully characterized with regard to degree of abnormality as well as localization. Like in the shoulder tendons, elbow tendon tears are described as partial or complete. A complete tear is diagnosed by a focal area of discontinuity (Fig. 11.15). Partial tears are further described by their location within the tendon. They are described as articular side, intrasubstance, or superficial in nature (Fig. 11.16). It is the preference of the author to further characterize the degree of tendon tear as low grade (less than 50% of the width of the tendon), moderate grade (50% of the width of the tendon), or high grade (greater than 50% of the width of the tendon). This system uses the internal standard of the patient’s own tendon width to help approximate the degree of abnormality identified by imaging. In addition, all lesions should be localized with regard to location within the tendon, from attachment site to musculotendinous junction.
FIGURE 11.12 Triceps. A: T1-weighted sagittal MR image and B: corresponding specimen photograph shows the combined tendon (arrow) and muscular (curved arrow) attachments of the triceps to the olecranon process.
FIGURE 11.13 Anconeus epitrochlearis. T1-weighted axial MR image demonstrates an accessory muscle, the anconeus epitrochlearis (arrow), posterior to the medial epicondyle and ulnar nerve (arrowhead). The anconeus (curved arrow) muscle is noted at the radial side of the joint. The close approximation and mass effect of the muscle on the subjacent nerve explain the association of this condition with ulnar neuritis and the cubital tunnel syndrome.
Epicondylitis and Overuse Syndromes
The clinical entity of epicondylitis is the most common source of elbow pain in the general population with lateral epicondylitis occurring seven to 20 times more frequently than its medial counterpart with an incidence of four to seven per 1000 patients seen in general practice (16–18). Although the term “epicondylitis” suggests an inflammatory process, the current consensus based on clinical and surgical evidence suggests that the underlying pathology within the tendon is that of microtears resulting in mucoid degeneration and neovascularization (1, 19, 20). Nirschl and Pettrone (3) described a characteristic microscopic appearance of “angiofibroblastic hyperplasia” of the involved tissue. The normal parallel orientation of collagen fibers is disrupted by an invasion of fibroblasts and vascular granulation-like tissue without an acute or chronic inflammatory component. In the series of surgically treated elbows reported by Nirschl and Pettrone, 97% (n = 88) demonstrated varying amounts of this gross pathologic tissue at the origin of the extensor carpi radialis brevis tendon; 35% also demonstrated gross tendon rupture. In addition to the chronic fibrous and vascular granulation tissue noted by Nirschl and Pettrone, Goldie (21) revealed the presence of free nerve endings within the tissue at the site of lateral epicondylitis, possibly explaining the symptomatic nature of this process. For the purposes of imaging diagnosis, it is the preference of the author to characterize the imaging appearance of the tendon as normal, tendinotic, or torn. Either the presence of tear or tendinosis could be consistent with the clinical entity of epicondylitis.
FIGURE 11.14 Signal intensity changes in tendinosis versus tendon tear. A: In the setting of tendinosis, signal changes encountered on T1-weighted images should not brighten to the level of simple fluid on long TE sequences (proton density- or T2-weighted), B: whereas with tendon tears, these changes brighten to the level of fluid on long TE sequences.
FIGURE 11.15 Full-thickness tendon tears. Full-thickness tears of the tendon are characterized by complete discontinuity of tendon fibers.
Lateral Epicondylitis
Clinical Diagnosis. As previously mentioned, lateral epicondylitis is a common source of elbow pain, first described over 100 years ago in a tennis player. This entity represents a pathologic condition of the common extensor muscles at their origin on the lateral epicondyle and is characterized by pain in that area on physical examination. The area of maximal tenderness lies 2 to 5 mm distal and anterior to the midpoint of the lateral epicondyle (19). Resisted wrist and finger extension with the elbow in full extension can intensify pain, whereas range of motion and sensation are generally normal. In some cases, wrist extensor weakness secondary to pain may be detected by the astute clinician. The clinical diagnosis is generally straightforward but can be confused with other causes for lateral-sided elbow pain. These causes include many of the previous theories posed to explain the pathophysiology of lateral epicondylitis: inflammation of an extra-articular radial humeral bursa, inflamed synovial fringe, fibrosis of the annular ligament, radial or posterior interosseous nerve entrapment, radiocapitellar chondromalacia, and cervical radiculopathy (19, 22–25). Clearly, MRI with its excellent soft tissue contrast and multiplanar imaging capabilities can be helpful in distinguishing between the aforementioned sources of lateral elbow pain.
FIGURE 11.16 Characterization of partial-thickness tendon tears. Partial-thickness tears of the tendon are characterized as A: articular-, B: intrasubstance-, or C: superficial-sided.
Mechanism. As previously noted, current theories indicate that lateral epicondylitis appears to begin as a microtear, usually in the origin of the extensor carpi radialis brevis, with formation of subsequent fibrosis and granulation tissue as a consequence of chronic repetitive microtrauma (3, 26). Activities that require forceful and repetitive forearm dorsiflexion, radial deviation, and supination stress the wrist extensors. Occupational activities that lead to epicondylitis include meat cutting, plumbing, painting, raking, and weaving. Recreational activities implicated in lateral epicondylitis include racquet sports such as squash, racquetball, and tennis, hence the moniker “tennis elbow.” This biomechanical overload has been demonstrated by electromyographic (EMG) studies that analyzed the activity of the muscles that stabilize the elbow while playing tennis. These studies showed the greatest activity during ground strokes to be within the musculotendinous unit stabilizing the wrist, in decreasing order: the ECRB followed by the ECRL and the extensor digitorum communis. These data support the theory that the ECRB, by overuse, is the musculotendinous unit most prone to be affected in this condition (27).
Further exploration into the specific components of overuse that result in the clinical expression of lateral epicondylitis suggest that increased age of the patient as well as increased time performing the offending activity play a role in the development of symptoms (19). Studies analyzing the two-handed versus single-handed backhand in tennis investigated the theory that players using the double-handed backhand rarely developed lateral epicondylitis because the helping hand both absorbs energy lessening the stress on the dominant hand as well as changes the mechanics of the swing. Giangarra et al. (28) found that extensor EMG activity was not decreased in the case of the double- versus single-handed backhand. Moreover, it was discovered that the activity in the helping and dominant hands was not significantly different from each other or from the single-handed technique. These results suggest that technique may be one of the most important factors in the development of symptomatic structural changes in the setting of overuse of the common extensor tendon and its components.
Imaging Diagnosis. As previously noted, the MRI diagnosis of pathology in the common extensor tendon focuses primarily on signal intensity and morphology changes to distinguish between tendinosis and tear. The common extensor tendon is best visualized in the oblique coronal imaging plane, arising from the undersurface of the lateral epicondyle, closely apposed to portions of the radial collateral ligament complex. It is imperative that a sequence with optimal fluid sensitivity and signal-to-noise resolution is included in the imaging protocol of the elbow. In a meta-analysis of the MR findings of humeral epicondylitis, four of seven studies included in the analysis used either a T2-weighted fat-suppressed imaging sequence or an inversion recovery sequence in the imaging protocol (17). As noted in the “Technical Considerations” chapter, some authors do use gradient recalled echo imaging sequences for evaluation of ten-dons and ligaments about the elbow (1). It is the bias of the authors to use fat suppression in conjunction with T2-weighted fast spin-echo imaging to improve conspicuity of signal changes in an effort to facilitate the distinction of tendinosis versus tears of tendons.
The MRI diagnosis of the clinical entity of lateral epicondylitis includes abnormal morphology and signal intensity of the common extensor tendon. Several imaging findings have been associated with the clinical entity of lateral epicondylitis. Not all studies localize tendon abnormalities to the specific components of the common extensor tendon; however, those that do attempt to localize it show pathology primarily in the ECRB (1, 15, 29–32). The spectrum of pathology encountered on MRI studies in this clinical entity includes abnormal separation of the radial collateral ligament and the ECRB with granulation tissue (Fig. 11.17). Potter et al. (1) showed this finding in 10 of 20 patients with lateral epicondylitis Savnik et al. (32) in 25 of 30 patients. In a recent study of 11 patients with the clinical diagnosis of refractory lateral epicondylitis, MRI demonstrated findings that suggested granulation tissue within the ECRB (n = 6). Enucleation of the granulation tissue provided symptomatic relief in all six symptomatic patients, underlining the importance of MRI as a decision-making tool in the treatment of this entity (33). The vast majority of patients with lateral epicondylitis show abnormal morphology of the ECRB in the form of abnormal thickening with abnormal signal intensity on the short TE sequences establishing the underlying diagnosis of tendinosis (Fig. 11.18). It must be emphasized that the imaging findings of tendinosis must be correlated with the clinical presentation before the diagnosis of lateral epicondylitis is made. In many studies, asymptomatic volunteers comprising a control group were discovered to have abnormal morphology and signal intensity of the tendon (1, 15, 32). In the absence of clinical symptoms, imaging findings likely represent subclinical tendinosis or early tendon degeneration.
FIGURE 11.17 Common extensor tendon pathology and granulation tissue. A: T2-weighted fat-suppressed coronal MR image through the elbow shows a partial articular-sided tear (arrow) manifested by high signal intensity within the common extensor tendon. Intermediate signal intensity material representing granulation tissue (curved arrow) is present between the radial collateral ligament and the common extensor tendon. B: Proton density-weighted fat-suppressed sagittal MR image through the common extensor tendon shows the intermediate-signal–intensity granulation tissue (curved arrow).
FIGURE 11.18 Common extensor tendinosis. A: T1-weighted and B: T2-weighted fat-suppressed coronal MR images show thickened morphology of the common extensor tendon (arrow) with no abnormal increased signal intensity on the T2-weighted image. Subtle osseous irregularity (curved arrow) is noted at the humeral attachment of the common extensor tendon.
Partial- or full-thickness tears of the ECRB superimposed on tendinosis are commonly encountered in patients with lateral epicondylitis. In the setting of partial-thickness tendon tears, attenuation of the tendon can be seen (1). Less common findings that have been reported in the setting of lateral epicondylitis include peritendon edema as well as focal bone marrow edema at the tendon attachment site to the humerus interpreted as avulsive injury (Figs. 11.19–11.21) (1, 15). It has been reported that 22% to 25% of patients with lateral epicondylitis may have calcification within the soft tissues about the lateral epicondyle (Fig. 11.22) (3, 19). This finding has not been commonly reported in MRI studies of the elbow in the setting of lateral epicondylitis. It appears that the presence of calcification has no prognostic implications and may disappear after treatment.
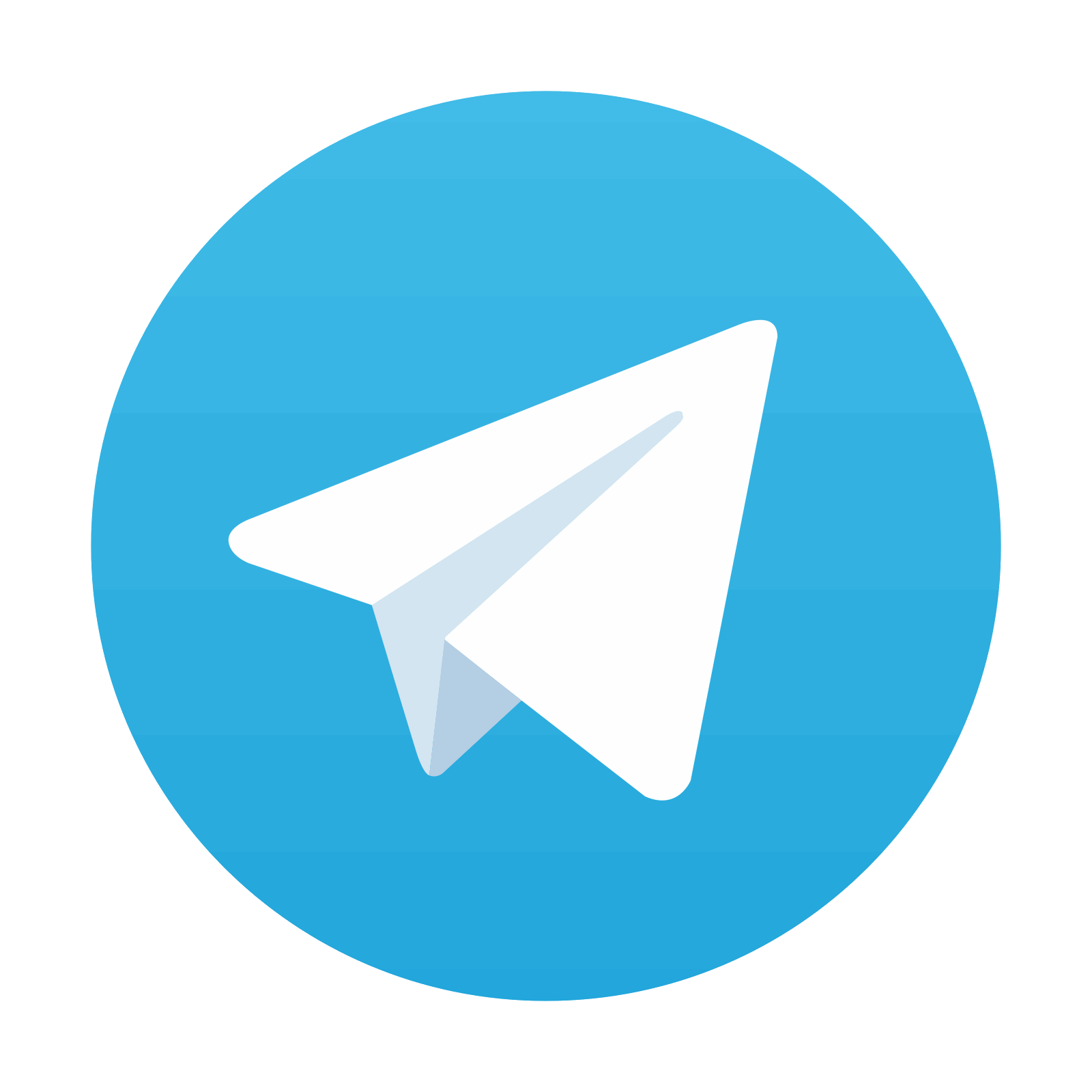
Stay updated, free articles. Join our Telegram channel

Full access? Get Clinical Tree
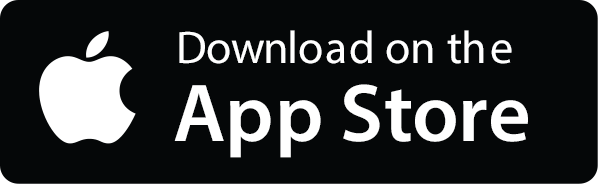
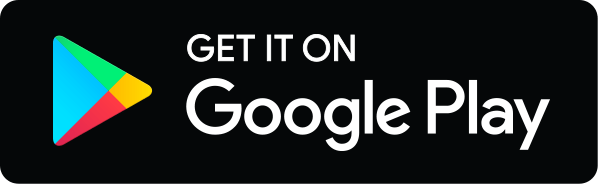