Introduction
Full-length radiographic spinal imaging is indicated in several clinical scenarios such as screening for congenital abnormalities of the spine, demyelinating processes affecting the spinal cord, scoliosis diagnoses, and trauma as well as many other clinical indications. Abnormalities that arise in early embryologic osseous and neurologic development may foresee the development of spinal deformities. Spinal imaging typically begins with a series of full-length radiographs, including AP, lateral, and supine images. Advanced imaging includes CT scan and MRI imaging, as detailed in earlier sections of this chapter. The purpose of this section is to describe how to accurately perform different radiographic measurements based on plain radiographs, CT imaging, and MRI, and discuss the clinical relevance of these findings.
Anatomy
A clear and detailed understanding of normal spinal anatomy is important in understanding the possible pathologic entities in spinal deformity. There are four distinct regions of the spine: cervical, thoracic, lumbar, and sacropelvic. In the normal spine, the cervical region consists of seven vertebral segments labeled C1-C7. The thoracic spine has 12 mobile segments: T1-T12. The thoracic ribs from T1 to T10 each have a pair of ribs that are joined anteriorly at the costochondral junction or sternum. T11 and T12 usually have “floating ribs,” which are not connected anteriorly to the rib cage. The lumbar spine typically has five mobile segments: L1-L5, except in cases where there is lumbarization or sacralization of the transitional vertebra. The sacropelvic unit consists of five fused segments of the sacrum that articulate with the ilium through the SI joints bilaterally and the coccyx.
Common Measurements and Radiographic Parameters in Full-Length Spine Radiographs
Overview of Radiographic and Advanced Spinal Imaging Techniques
Standard full-length spine radiographs for adults are typically taken on 36-in. cassette standing films to assess coronal (AP radiograph) and sagittal plane alignment (lateral radiograph). The patient should be in the standing position with the hips and knees fully extended to limit compensatory realignment from hip and knee flexion. Often, depending on the type of deformity, additional imaging is performed such as supine and/or right and left bending films, which aid in assessing curve flexibility. Assessment of curve flexibility has traditionally been used to select patients who could undergo a selective fusion of only the thoracic component of a double major curve, thus sparing lumbar motion segments. Curve flexibility also allows the surgeon to evaluate structural and nonstructural deformity, and has important implications on deformity classification, which is discussed in more detail later in this chapter. Both supine traction imaging and side bending films are valid methods to assess curve flexibility; side bending films show greater flexibility in the thoracic spine with curves between 50° and 60°, whereas in the lumbar spine both films show equivalent flexibility. Additionally, other imaging to assess curve flexibility include push-prone, supine AP, fulcrum-bend (thoracic curve), traction AP, and supine hyperextension crosstable lateral radiographs. Such imaging is important, as patients who had full-length standing spine radiographs preoperatively have been shown to have a significantly lower rate of revision surgery at 5 years postoperatively.
CT scans of the spine provide an excellent visualization of cortical bone and calcifications. Recent techniques to improve the accuracy of screw placement intraoperatively include utilizing low-dose CT scans of the patient’s spine to build a virtual model to plan the screw trajectories and a 3D-printed patient-specific guide system to prepare the screw trajectories. Screw misplacement incidence can be as high as 15%–30% in spinal deformity surgery, especially with congenital, revision, or severe deformity cases. In patients with severe spinal deformity or multiple prior spinal surgeries, the bony spinal anatomy can be severely altered, which makes freehand pedicle screw placement more challenging. In these cases, 3D-printed spinal models placed in sterile bags and studied intraoperatively have been found to increase the safety of freehand pedicle screw with acceptable accuracy, and no neurological or vascular complications . These patient-specific models can also be used preoperatively to facilitate patient education regarding the specific pathology and planning the surgical procedure.
Compared with other modalities, MRI does not use ionizing radiation. MRI also provides better soft tissue contrast and allows direct visualization of the spinal cord, nerve roots, and intervertebral discs. Disorders of the spine that may warrant MRI evaluation include congenital spine and spinal cord malformations, inflammatory disorders, infectious conditions, vascular disorders, degenerative disorders, and neoplastic abnormalities.
Classification Systems
Lenke classification
The spine has a standard characteristic alignment in the coronal and sagittal planes. In the coronal plane, the spine is straight. Scoliosis is defined as the presence of one or more lateral rotatory curves of the spine measured in the coronal and sagittal planes. The most prevalent classification system used for scoliosis is the Lenke classification system. This classification system was developed in 2001 to guide the surgeon in determining appropriate fusion levels by avoiding unnecessary fusion of the nonstructural lumbar or thoracic spine as well as avoiding undercorrection of the structural secondary curves. This classification scheme improved upon the King classification system by emphasizing the sagittal plane along with the coronal plane while demonstrating good-to-excellent intraobserver and interobserver reliability. The selection of fusion levels is a heavily debated topic in spinal deformity literature. Various measurements taken from the AP radiographs are compared with predetermined numeric values that allow the identification of structural and nonstructural curves. This classification system takes into account the structural, nonstructural curves, shoulder balance, and thoracic kyphosis to determine the upper instrumented and lower instrumented vertebrae. The details of fusion-level selection are beyond the scope of this chapter but can be referred to in the original Lenke et al.’s classification or in subsequent review articles with long-term follow-up discussing the selection of upper and lower instrumented vertebrae for different Lenke curve types.
After the Cobb angles (discussed later) are determined for the various curves, the regional curves are then determined, which are defined as the proximal thoracic (PT), main thoracic (MT), and thoracolumbar/lumbar (TL/L) curves. Adult scoliosis in addition to having TL/L curves will often have a fractional lumbosacral (FL) curve below the TL/L curve, defined in the region L4-S1. Identification and treatment of the fractional curve can lead to improved patient outcomes and decreased revision rates in this complicated pathologic process. Each curve is defined by the level of its respective apex. Thoracic curves have the apex located between the T2 and T11-T12 disc, thoracolumbar curves between T12 and L1, and lumbar curves between the L1–2 disc through L4.
To begin classification, the structural or nonstructural quality of each of the three curves must be determined. The major curve will always be the MT or TL/L, whichever is the largest curve. The other two curves in a scoliotic spine are defined as minor curves, which may be defined as structural or nonstructural. Curves are considered structural if they are ≥ 25° on the standing AP radiograph and do not bend out to < 25° on the side-bending radiographs. Minor curves < 25° on the standing AP radiograph by definition will be nonstructural. However, minor curves may be deemed structural if their regional sagittal profile reveals a kyphosis ≥ + 20°. After determining the structural or nonstructural nature of each regional curve, the Lenke type can be assigned according to Fig. 1 .
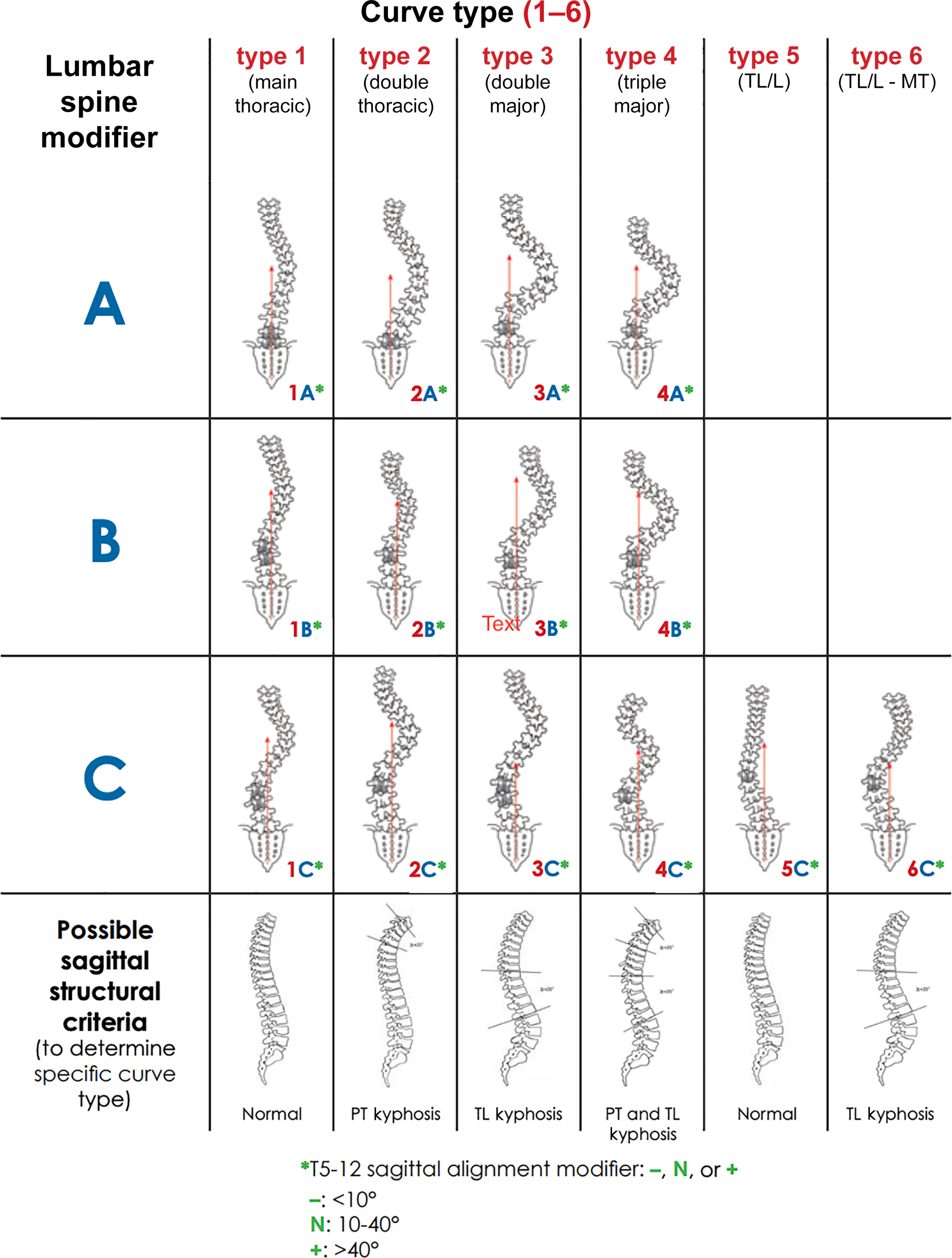
Once the Lenke curve type is assigned, the practitioner can then assign the lumbar modifier (A, B, or C). To perform this assignment, a center sacral vertical line (CSVL) is drawn vertically from the midpoint of S1. If the CSVL passes between the pedicles of the apical lumbar vertebra, the lumbar modifier A is assigned. If the CSVL falls between the medial edge of the pedicle and the lateral edge of the vertebral body, the lumbar modifier B is assigned. If the CSVL does not touch the lateral edge of the apical lumbar vertebra, the lumbar modifier C is assigned.
The thoracic sagittal modifier is added by measuring the sagittal Cobb angle between T5 and T12. If the T5-T12 sagittal Cobb is less than 10 degrees, the sagittal thoracic alignment is considered hypokyphotic and is assigned a minus modifier (−). If the sagittal Cobb is between 10 and 40 degrees, the sagittal alignment is considered normal (N). If the sagittal Cobb measurement between T5 and T12 is greater than 40 degrees, the sagittal alignment is considered hyperkyphotic and is assigned a plus modifier (+).
The Lenke system was developed to guide the practitioner in performing selective fusions, which optimizes mobile segments of the spine in patients with scoliosis. When contemplating fusion levels, radiographic parameters such as curve ratios, Cobb magnitude, apical vertebral translation and rotation, as well as relative flexibility assessments of the two curves, are used. In one series looking at selective fusion for Lenke curve types 1–2, minor global imbalance was measured on the long-term follow-up and patients demonstrated good maintenance of curve correction and global balance. However, the benefits of correcting spinal balance must be weighed against the morbidity of performing increasingly extensive surgery. Though thoracolumbar anterior fusions were associated with better radiographic outcomes, SRS-24 Questionnaire data showed no clinical difference between patients fused anteriorly vs. posteriorly.
The Lenke classification system allows for less confusion between surgeons with corresponding surgical fusion guidance and planning. Since the advent of the classification scheme, there has been a significant reduction in the variation of treatment approaches. The reliability of the Lenke curve-type classification is categorized as good-to-excellent by many studies with inter- and intraobserver reliability rates of 86.5% and 87.4%, respectively. Other studies have shown that better radiologic results were achieved through the use of the Lenke classification system for the selection of fusion levels and avoiding undercorrection of the structural secondary curves in Types 2, 3, and 6 Lenke spines.
SRS-Schwab classification
In 2012, Schwab et al. and the SRS published a hybrid classification system which utilized radiographic parameters with patient-reported outcomes to design an adult idiopathic scoliosis classification system ( Fig. 2 ). This hybrid system also includes radiographic pelvic parameters, such as pelvic incidence, lumbar lordosis, pelvic tilt, and sagittal vertical axis, to group the curves into seven curve types and three modifiers accounting for regional sagittal, lumbar degenerative, and global alignment. This newer SRS-Schwab classification system improved on the older Schwab classification system by including these spinopelvic parameters linked with pain and disability to establish treatment recommendations. The SRS-Schwab classification system uses frontal and sagittal full-length radiographs to determine coronal curve types and sagittal curve modifiers. This classification scheme is summarized in the table below. The coronal curve types are grouped into one of four types: Type T with a thoracic major curve > 30° and a lumbar curve < 30°, Type L with a lumbar or thoracolumbar major curve > 30°, Type D with a double major curve with each curve > 30°, and Type N (normal) with no coronal curve > 30°.
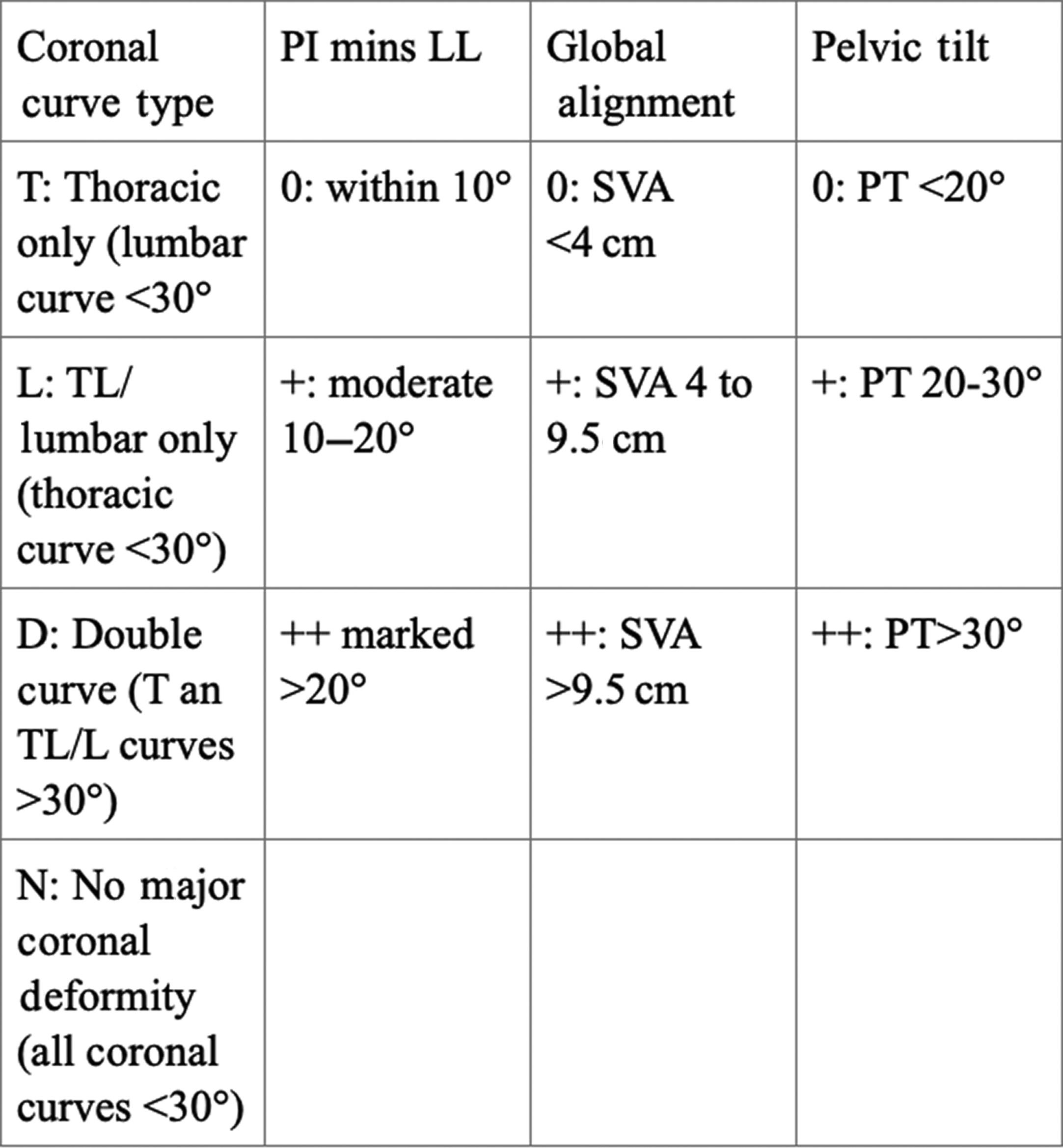
The first sagittal modifier used in this classification scheme takes into account two radiographic parameters, namely, pelvic incidence and lumbar lordosis, to calculate the difference between the two in order to achieve proper postoperative lumbar alignment. The pelvic incidence minus lumbar lordosis modifier is labeled “0” if the mismatch is < 10°, modifier “+” if it is between 10° and 20°, and modifier “++” if > 20°.
Pelvic tilt is an important sagittal modifier to measure because patients with greater pelvic tilt often need larger corrections to reduce the risk of postoperative failures. Patients are classified as pelvic tilt modifier “0” if the measurement is < 20°, modifier “+” if it is between 20° and 30°, and modifier “++” if the pelvic tilt is > 30°.
The last sagittal modifier is the global alignment modifier, which is based off of the sagittal vertical axis (SVA). Patients are classified as having a sagittal vertical axis modifier “0” if the measurement is < 40 mm, modifier “+” if it is 40 to 95 mm, and modifier “++” if > 95 mm.
The SRS-Schwab system focuses on spinopelvic parameters that have been shown to correlate with better health-related quality-of-life (HRQoL) scores after surgery. SRS-Schwab classification reflects severity of disease state based on multiple measures of (HRQoL); those with worse modifier grades required more extensive surgery to correct the deformity. Recent studies have also shown that the SRS-Schwab classification system can predict patient disability and guide preference for nonoperative versus operative treatment decisions based on the preoperative sagittal spinopelvic alignment. Patients with thoracolumbar and primary sagittal deformities had worse outcomes and greater disability than other curve types. These studies also found operative managed patients to have worse spinopelvic modifier grades than nonoperative patients with increasingly worse grades correlating with worsening HRQoL scores. Inter- and intraobserver reliability rates for the SRS-Schwab classification system are high and have been shown to be consistent in predicting prognosis and guiding treatment decisions for surgeons. The SRS-Schwab system has great utility in regard to treatment decisions and prognostic information.
Roussouly spinal shape classification
The Roussouly classification developed in 2003 describes four common variants in lumbar lordosis by classifying the sagittal profile and the spinopelvic alignment ( Fig. 3 ). Type 1 and 2 spinal shapes have a low sacral slope defined as < 35°. Type 1 spines are characterized as a nonharmonious back with thoracolumbar kyphosis and short hyperlordosis. Type 2 is characterized by a longer and flatter distal arch, close to a straight line. It is a harmonious flat back. Type 3 variants have a mean sacral slope between 35° and 45°. It is a harmonious regular back. Type 4 consists of spines with a high sacral slope > 45°. The distal arch has an increased angle and number of vertebrae; it is a harmonious hypercurved back.
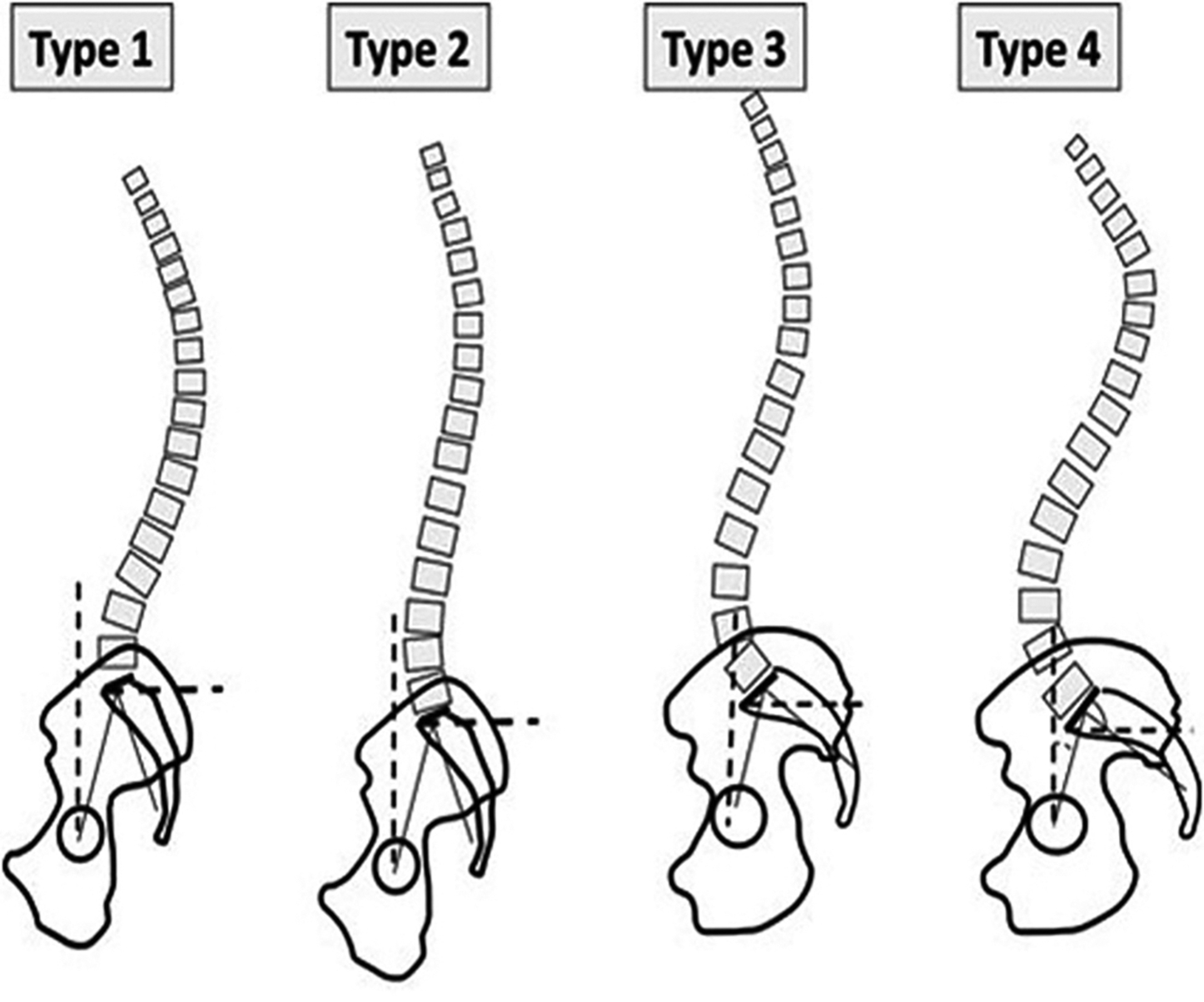
Depending on the type of classification, different degenerative changes of the spine can be predicted based on the mechanical stresses according to the geometry of the lordosis and spine balance. These different stresses include both sliding forces parallel to the endplate and pressure forces perpendicular to the endplate. An understanding of how different types of spinopelvic complexes can lead to different patterns of degenerative spine disease may help the surgeon tailor the treatment strategy for his or her patient.
The Roussouly classification allows the surgeon to form a correlation in shape and positioning, and form and function, between the pelvis and the spine. Patients with a low-grade PI need a restoration of Type 1 or 2 spine type, without increasing the lordosis. With increasing pelvic incidence, additional lumbar lordosis has to be augmented in angle and length, to reduce the posterior tilt of the pelvis. Patients who both matched Roussouly sagittal spinal type and improved in SRS-Schwab modifiers had superior patient-reported outcomes at 1 year. Utilizing both classification systems in surgical decision-making can optimize postop patient outcomes.
Spondylolisthesis classification
Spondylolisthesis is defined as the anterior, posterior, or lateral translation of one vertebral body relative to an adjacent level. When a slipped vertebra is identified, further assessment is warranted to determine the stability between two adjacent levels. To measure the subluxation, the distance technique can be used to measure the degree of subluxation in millimeters. The most prevalent grading system for spondylolisthesis is the Meyerding classification, which divides the superior endplate of the caudal vertebra into four quarters ( Fig. 4 ).
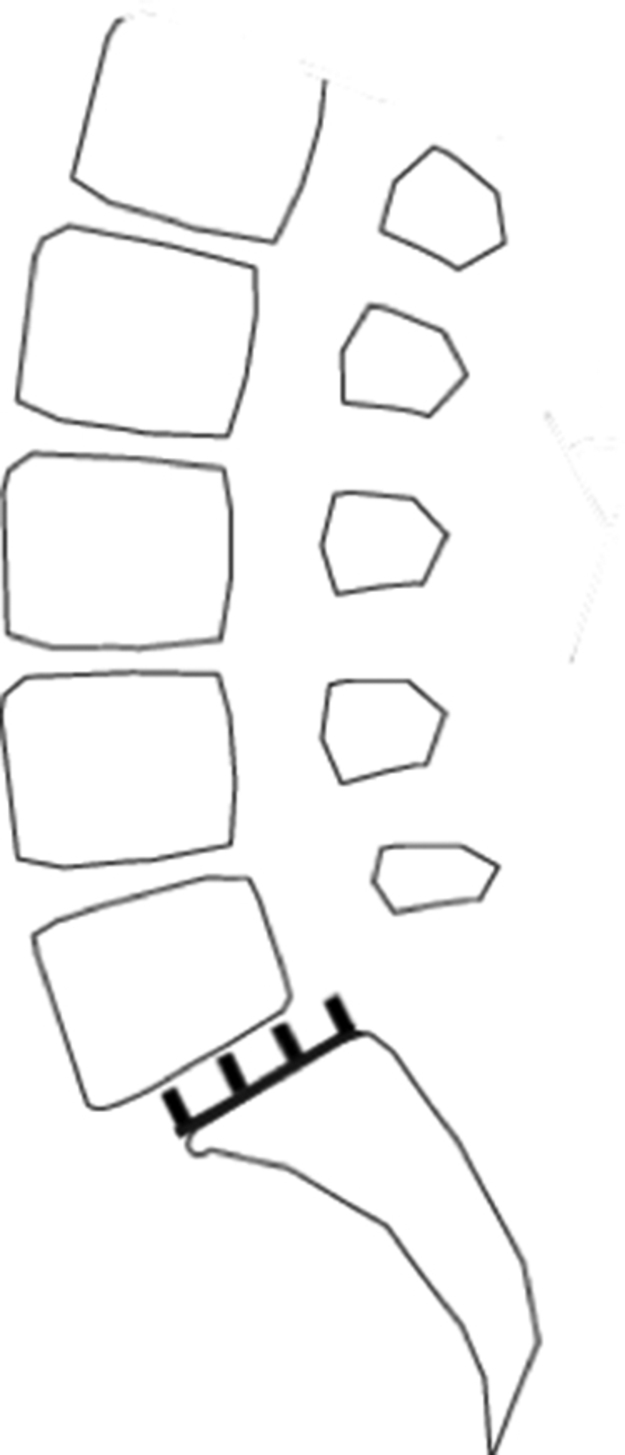
Vertebral rotation classification
Measurement of vertebral rotation is of key significance in the prognosis and treatment of scoliotic curves. This variable has been shown to be related to curve progression, thus being clinically applicable for both preoperative and postoperative assessment. Furthermore, knowledge of vertebral rotation can decrease the risk of pedicle screw misplacement, which may lead to spinal cord injury. The different classification methods for vertebral rotation include ones described by Cobb, Nash and Moe, Perdriolle, Stokes, Aaro-Dahlborn, and Ho et al. ( Fig. 5 ). These classification schemes are discussed at length later in this chapter.
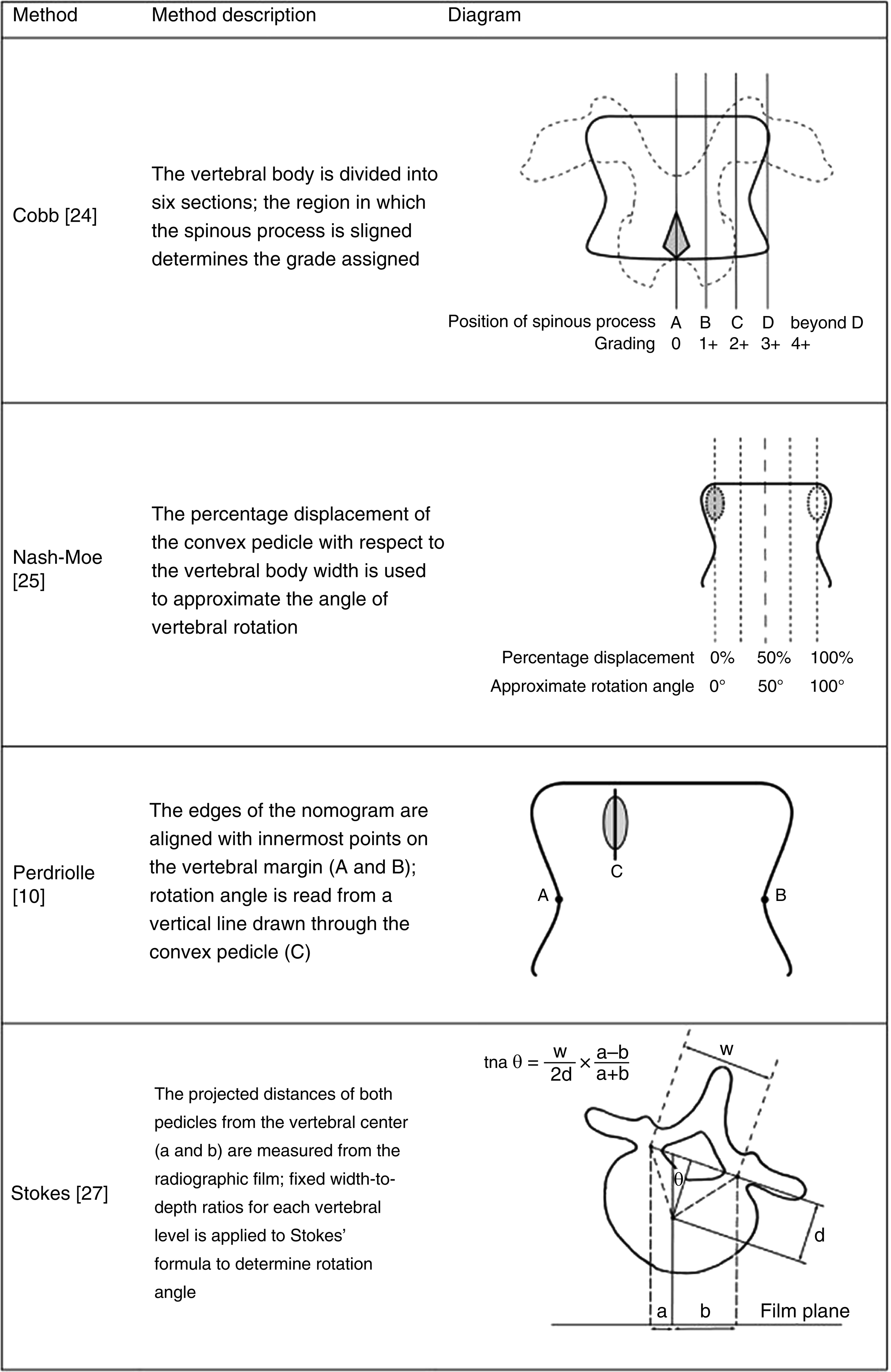
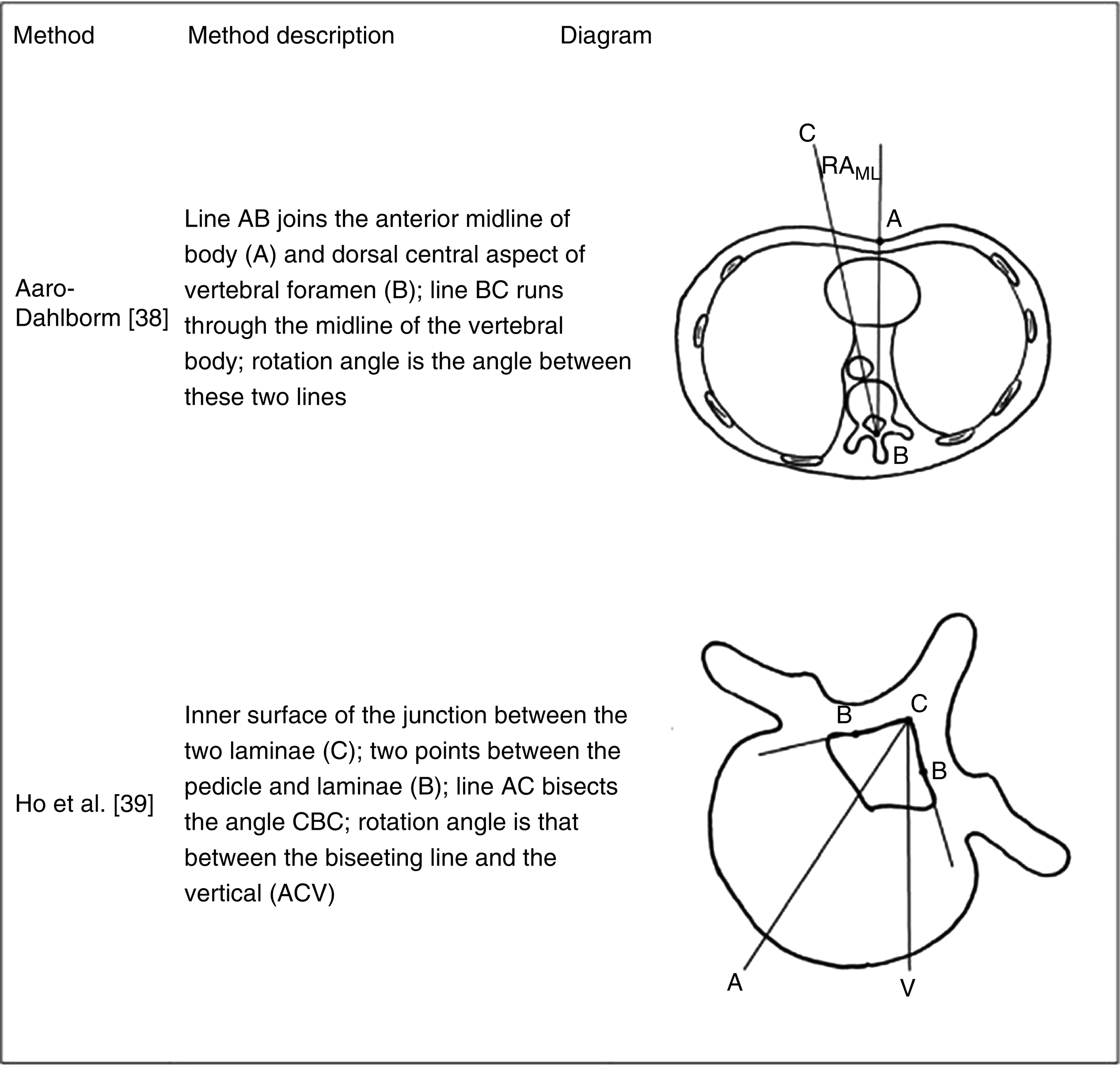
In the following sections, various measurements that can be obtained from the full-length spinal radiographs and advanced imaging will be discussed in detail.
Coronal Alignment and Radiographic Measurements
Cobb angle
The Cobb angle is the most widely used measurement to quantify the magnitude of spinal deformities measured on radiographs. To measure the Cobb angle, the end vertebrae of the curve deformity are chosen for each curve segment that exists. End vertebrae are the most tilted vertebrae at the cephalad and caudal ends of a curve. Lines are then drawn along the endplates, and the angle between the intersection of the two lines is then measured ( Fig. 6 ). In cases where the lines will not intersect on the film or monitor, two lines at right angles to the previous lines can be drawn and the angle between these new lines measured ( Fig. 6 ). Some caution should be used during Cobb angle measurement, which has approximately a 5–10 degree intraobserver and interobserver variation. It is also important to keep rotation in mind, as minor rotation of patients between examinations can significantly change measurements. The severity of thoracic and lumbar Cobb angles is correlated with an increased progression of spinal deformity. Bracing is typically initiated for curves greater than 25 degrees, while surgical intervention is typically pursued for curves measuring more than 45 degrees.
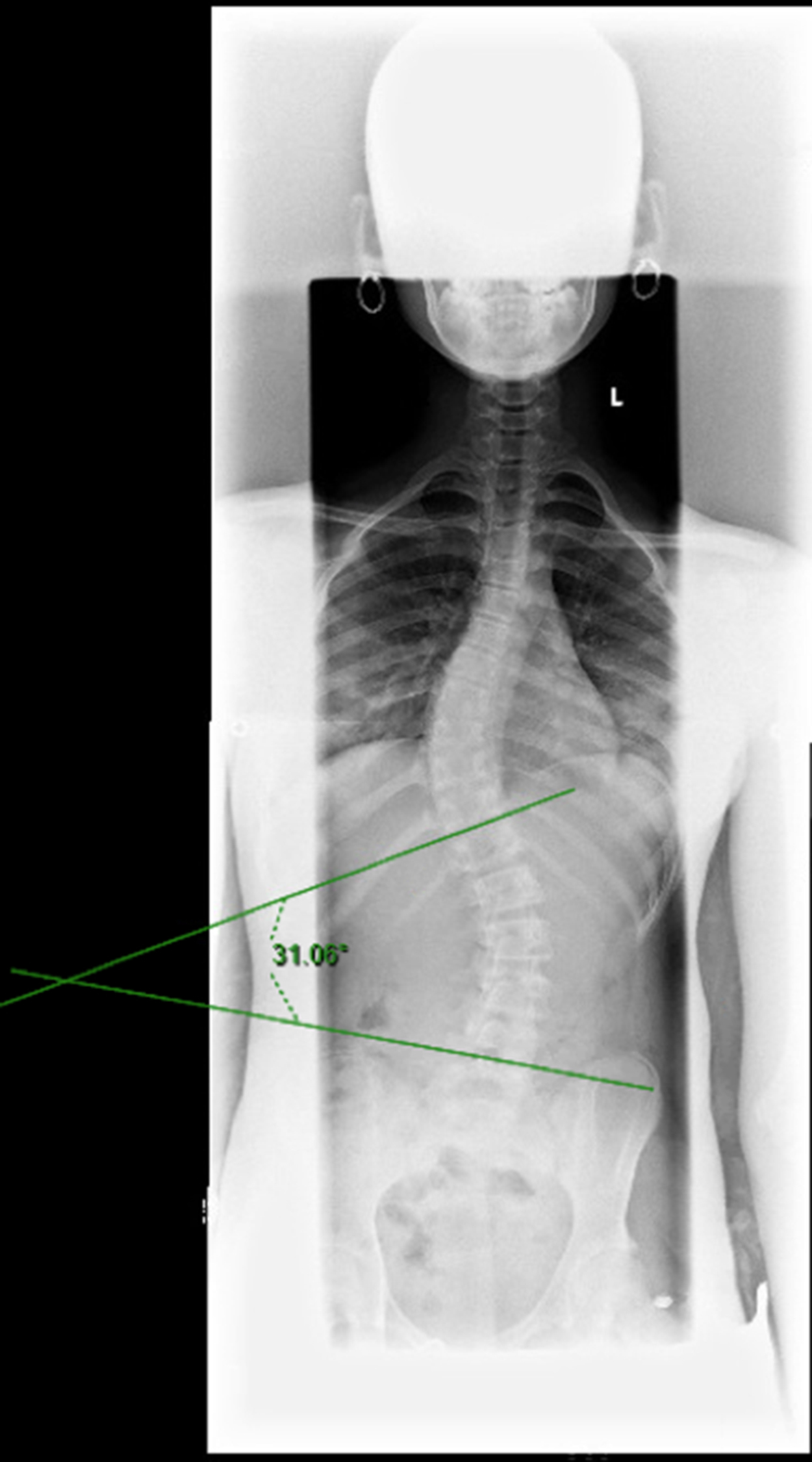
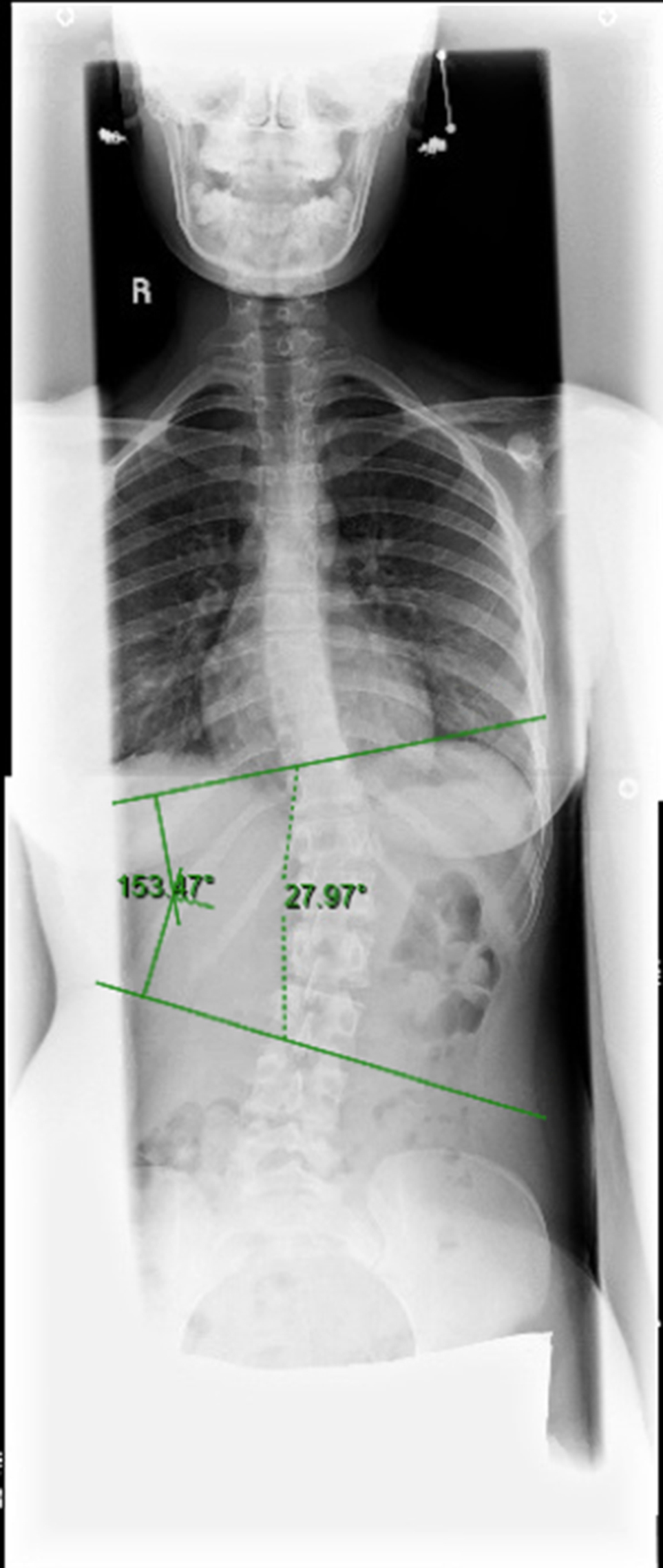
Instrumented vertebra tilt angle
The upper instrumented vertebra (UIV) tilt angle is measured by drawing a line along the cephalad endplate of the UIV. A second line is drawn perpendicular to the vertical edge of the radiograph and intersects Line A at the edge of the UIV. When the left edge of the vertebral body is up, the tilt angle is defined as positive. This measurement was found to be an important postoperative radiological parameter that has a significant correlation with postoperative neck and medial shoulder imbalance and measures of T1 tilt and cervical axis measurement. The lower instrumented vertebra (LIV) is measured similarly, using a line drawn along the caudal endplate of the LIV and a line perpendicular to the vertical edge of the radiograph. Smaller UIVDiff (difference between postoperative UIV tilt and preoperative optimal UIV tilt) angles are associated with better lateral shoulder balance.
T1 tilt angle
The T1 tilt angle is an angle formed by a line drawn between a horizontal line and the superior endplate of T1 or along the zenith of both first ribs if the T1 endplate is not well visualized ( Fig. 7 ). This measurement has been found to have a moderate positive correlation with SVA (dens) and can be used as a good predictor of overall sagittal balance. When the T1 tilt was higher than 25°, all patients in the series by Knott et al. had at least 10 cm of positive sagittal imbalance and patients with negative sagittal balance had mostly low T1 tilt values, usually below 13° of angulation.
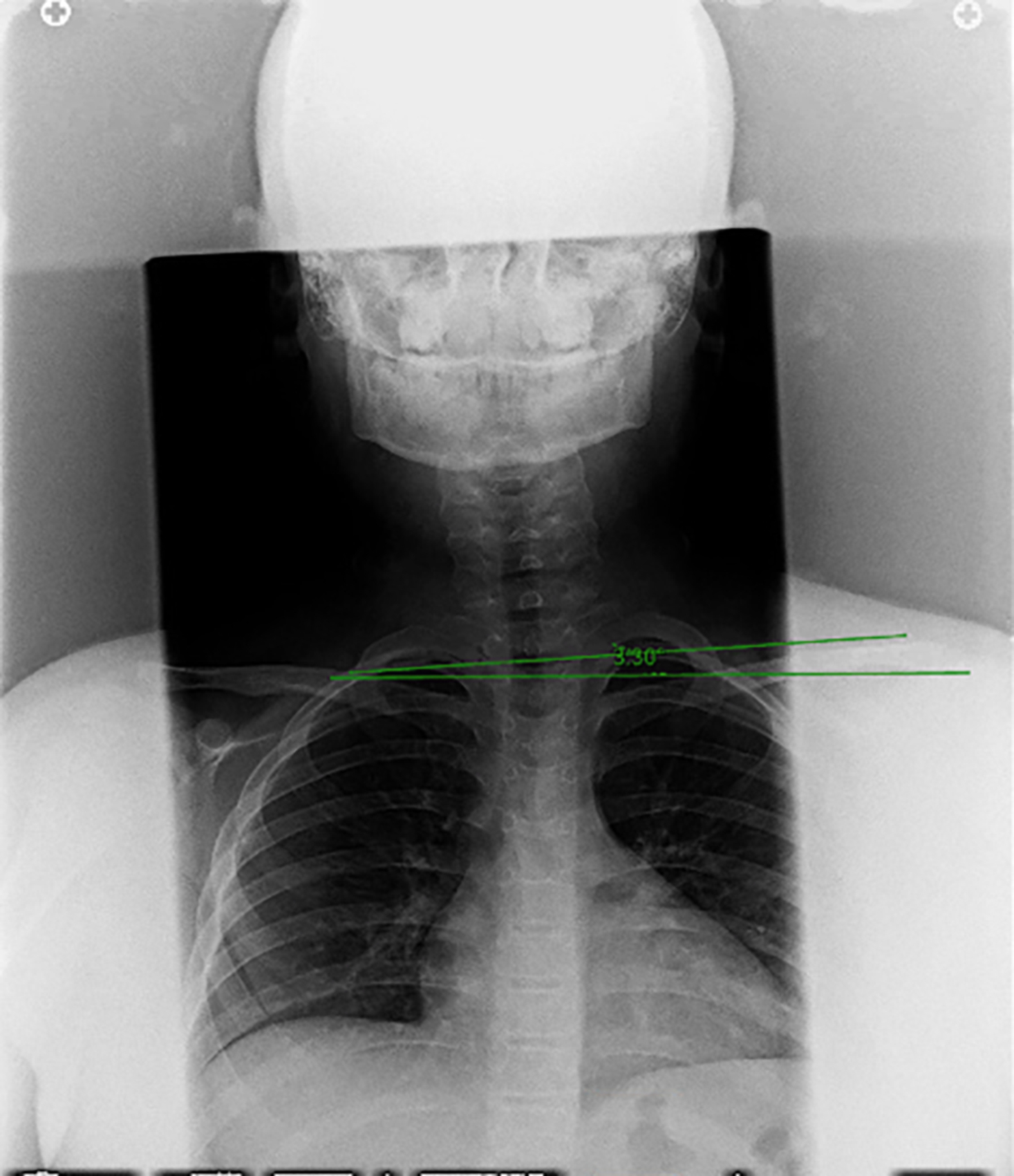
Clavicle angle
The clavicle angle is an angle drawn between a horizontal reference line and clavicle horizontal reference line (CHRL), which is drawn perpendicular to the lateral edge of the radiograph and touches the most cephalad portion of the elevated clavicle and a line which touches the most cephalad aspect of both the right and left clavicles (clavicle reference line) ( Fig. 8 ). Angles in which the left shoulder up are positive and angles with the right shoulder up are negative. This directionality is consistent with that of the T1 tilt angle described earlier. Clavicle tilt has been shown in some studies to have an association with postoperative shoulder imbalance.
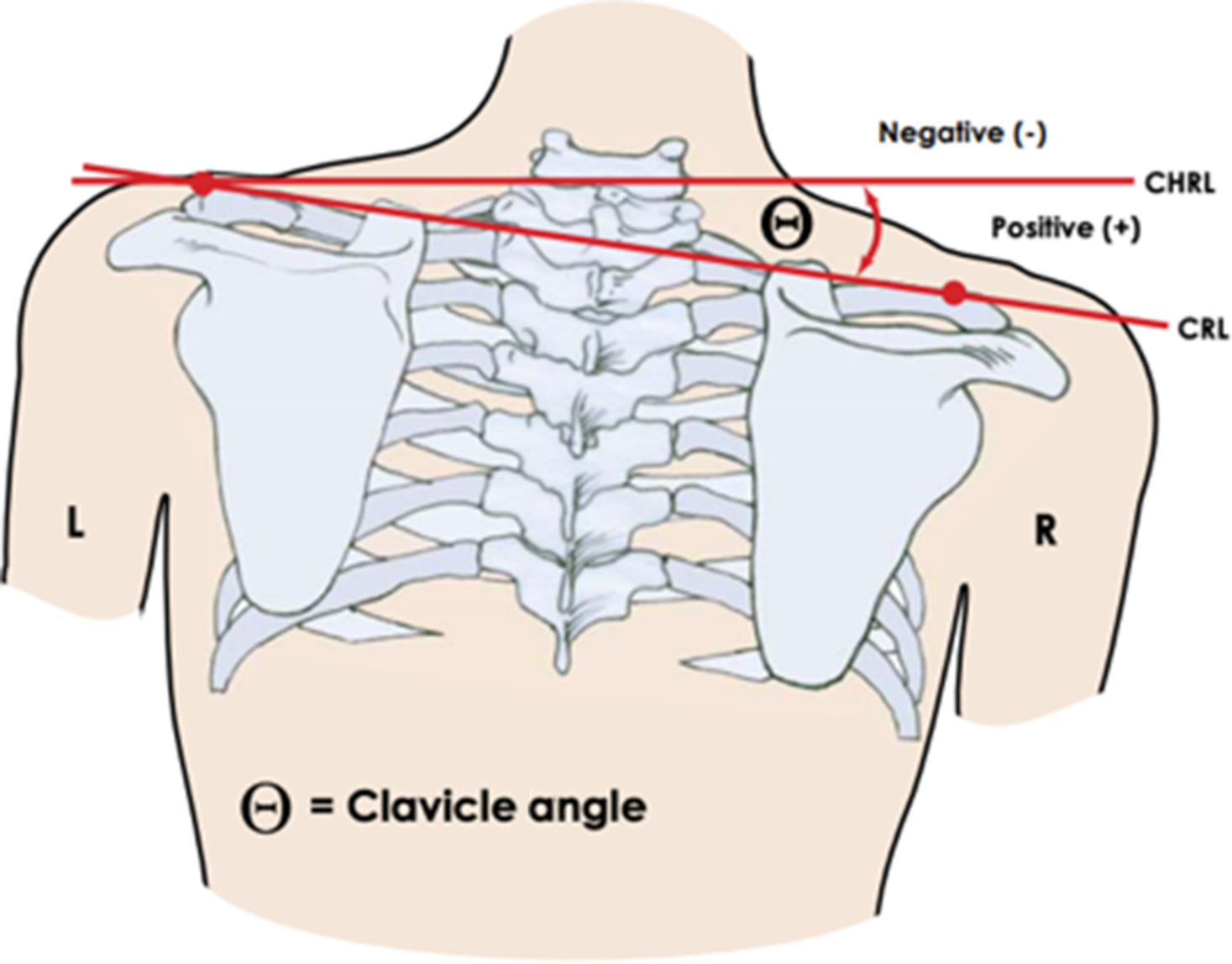
Radiographic shoulder height
Radiographic shoulder height is defined as the distance measured in millimeters between the superior horizontal reference line (SHRL) and the inferior horizontal reference line (IHRL) ( Fig. 9 ). The SHRL passes through the intersection of the soft tissue shadow of the shoulder and a line drawn vertically up from the acromial clavicular joint of the cephalad shoulder. The IHRL is a similar line drawn over the caudal acromial clavicular joint. The distance the linear distance between these two reference lines is measured in millimeters and defined as positive if the left shoulder is up and negative if the right shoulder is up. Again, by convention, left up is always positive and right up is always negative.
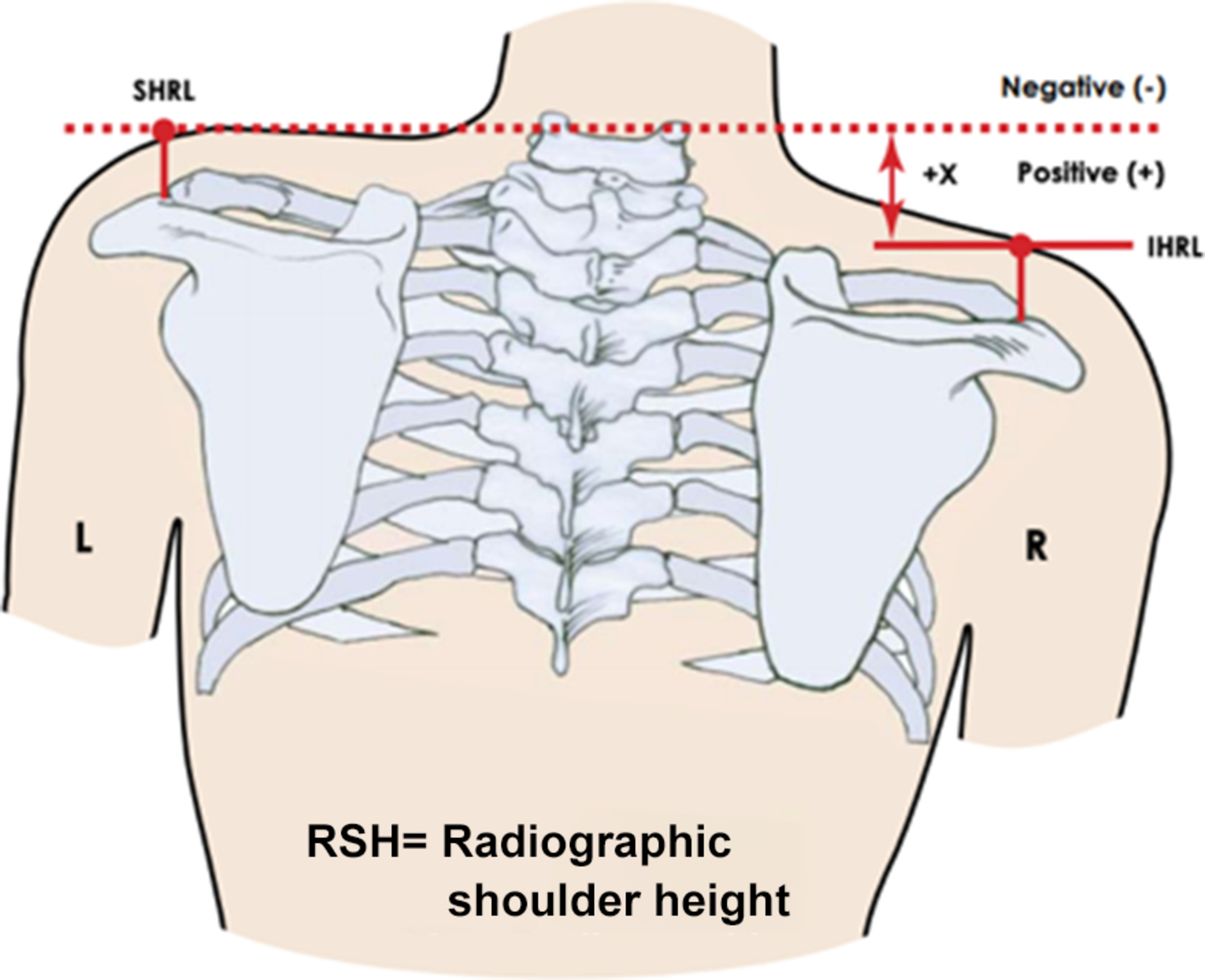
Thoracic trunk shift
Thoracic trunk shift is measured on standing PA or AP full-length spine films. Once the apical thoracic vertebra is identified, a horizontal reference line is drawn through the center of the vertebra and extending to the right and left rib cages. The midpoint of the reference line is marked and a perpendicular line is dropped as a vertical trunk reference line (VTRL). Trunk shift is then calculated by measuring the linear distance in millimeters between the VTRL and the CSVL ( Fig. 10 ). A trunk shift to the right of the CSVL is a positive value, and to the left of the CSVL a negative value. Trunk shift is a spinal imbalance in which the thorax is not centered over the pelvis. LIV selection and ratio of MT to TL/L curve are highly correlated with the onset of postoperative trunk shift in Lenke Type 1C curves.
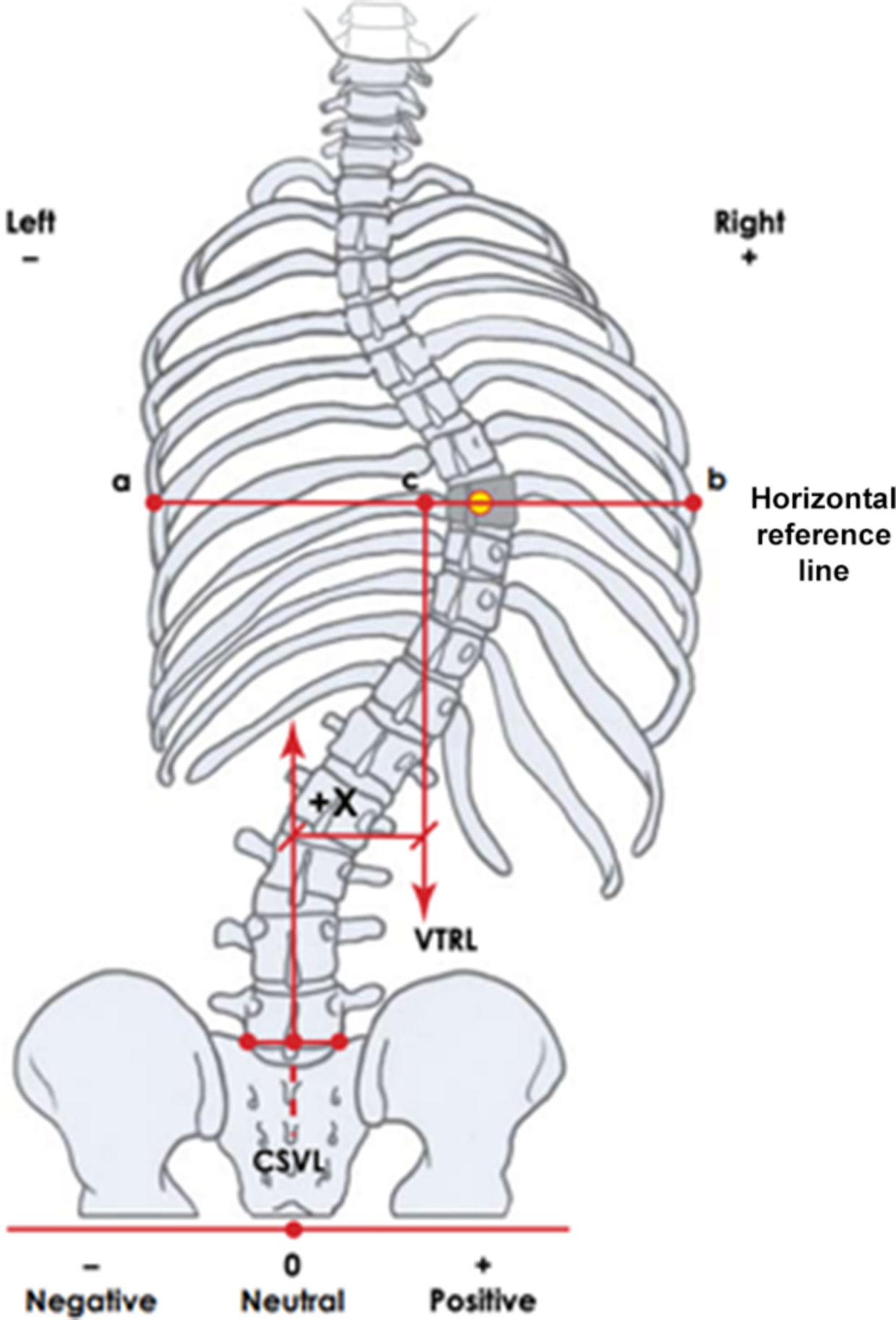
Pelvic obliquity
Pelvic obliquity can be measured using several different reference points and a horizontal reference line. The tips or sulcus of the sacral ala can be used to create the pelvic coronal reference line (PCRL) for the pelvic orientation in the coronal plane. An angle between this line and a horizontal reference line is then measured ( Fig. 11 ). Alternatively, the top of the ilium may be used to create the PCRL. Leg length discrepancy (LLD) is measured using PA standing radiographs without blocks under the patient’s feet and with the knees extended. A femoral horizontal reference line (FHRL) will be created by making a horizontal line that is tangent to the top of the highest femoral head or at the level of the lesser trochanter. The difference between the height of this line and the height of the lower femoral head is then measured as the LLD. If the left hip is up, the value is positive (+). If the right hip is up, then the value will be negative (−). Poorer clinical outcomes have been associated with sagittal (C7-SVA, PT, LL, sagittal LIVDA) and/or coronal imbalance (C7-CSVL, shoulder-tilt, and pelvic obliquity).
