Genitourinary System Scintigraphy
Howard A. Carpenter
Cameron C. Foster
Renal Imaging
Renal radionuclide imaging has always been an important part of the practice of nuclear medicine. US, CT, and MRI have the clear advantage of superior anatomic images, arterial and venous blood flow velocity data, renal mass density, and neoplasm imaging.
Despite advances in US, CT, and MRI, there remain many areas where scintigraphy remains the easiest, least expensive, and most accurate test. Radiopharmaceuticals are well suited for evaluating renal function including blood flow, glomerular filtration rate (GFR); effective renal plasma flow (ERPF); nephron uptake and clearance; renovascular hypertension/renal artery stenosis (RAS); acute and chronic renal failure, ischemic nephropathy; pyelonephritis, trauma or surgical complications; renal transplant function, obstruction, and acute or chronic rejection; and ureteral obstruction and vesicoureteral reflux. Radionuclide renal studies are safe, minimally invasive and expose the patients to radiation doses comparable or less than competing radiologic procedures. Functional test with radionuclides offers no risk of contrast-induced nephropathy (CIN) or nephrogenic systemic fibrosis from gadolinium contrast MRI.
Renal Function
Accurate diagnostic interpretation can be made by understanding the sequential-functional dynamic movement of the selected radiopharmaceutical from the blood to the bladder.
Renal plasma flow from cardiac output averages 600 mL/minute. Approximately 20%, or 120 mL/minute, is filtered through the glomerulus, and the remaining 80% of the renal plasma clearance occurs through tubular secretion.
Glomerular filtration is a function of the pressure gradient across the glomerulus and the glomerular permeability. The pressure gradient is responsible for driving filtration from the afferent arterioles into the renal tubules. Small charged molecules such as water, electrolytes, creatinine, urea, and technetium (Tc)-99m-DTPA (diethylenetriamine penta-acetic acid) are all filtered. The glomerular pressure gradient is decreased, on the preglomerular side, by heart failure, renal artery stenosis, and microvascular disorders. On the postglomerular side, renal collecting system/ureteral obstruction and ischemic nephropathy decrease the pressure drop, resulting in a reduction in the amount of filtrate. Intrinsic diseases such as glomerulonephritis and chronic renal failure disrupt glomerular membrane permeability, leading to scarring and sclerosis, resulting in diminished filtration and tracer movement.
Tubular secretion is an active process by which some larger molecules, and those protein bound, are removed from the peritubular capillaries and secreted into the glomerular filtered tubular urine. For a radiopharmaceutical to effectively trace tubular function, most or all of the tracer must be removed in one pass by the tubule, that is, it must be secreted into the urine and not be reabsorbed. Historically, I-131-orthoiodohippurate (I-131-OIH) was used because of its high tubular extraction efficiency and lower protein binding. I-131 has a long half-life of 8 days, emits a high energy of 364-keV photon that is suboptimal for imaging, and emits a cellular damaging beta-particle. I-131-OIH has been replaced by Tc-99m agents such as Tc-99m-MAG3 (mercaptoacetyltriglycine).
Calyces, collecting system, ureters, and bladder can be imaged by numerous radiopharmaceuticals that pass from the renal parenchyma into the collecting system. Conditions such as obstructed/nonobstructed hydronephrosis, megaureter, and urinary system leaks can be evaluated with Tc-99m-MAG3, Tc-99m-DTPA, and Tc-99m-GH (glucoheptonate). Tc-99m-sulfur colloid and Tc-99m-DTPA are directly instilled into the bladder and used to image reflux in pediatric patients.
Radiopharmaceuticals
Radiopharmaceutical renal imaging can be grouped into four main functions: blood flow, glomerular filtration and ERPF, renal tubular function, and excretory system function. The radiopharmaceutical doses should be adjusted for body surface area for pediatric patients, using available nomograms or by employing Webster’s rule:

Tc-99m-DTPA (10 to 20 mCi, 370 to 740 MBq) is a small molecule that is completely filtered at the glomerulus and is most commonly used for GFR calculations and reflux imaging when instilled into the bladder. It is not significantly protein bound when properly prepared (approximately 1%), and there is no
tubular secretion or reabsorption. These characteristics result in a lower extraction fraction than Tc-99m-MAG3, which is secreted into the tubules. This lower extraction fraction and relative lack of protein binding result in a lower target-to-background ratio and subsequent lower-resolution images. In a patient with already low levels of renal function, this effect is exaggerated.
tubular secretion or reabsorption. These characteristics result in a lower extraction fraction than Tc-99m-MAG3, which is secreted into the tubules. This lower extraction fraction and relative lack of protein binding result in a lower target-to-background ratio and subsequent lower-resolution images. In a patient with already low levels of renal function, this effect is exaggerated.
Tc-99m-DMSA (dimercaptosuccinic acid) (1 to 5 mCi, 37 to 185 MBq) is an excellent renal cortical imaging agent, although the mechanism of uptake and clearance is not well understood. Approximately 40% of the injected dose eventually binds to the sulfhydryl groups in the proximal renal tubules. With minimal collecting system activity, high-resolution imaging can be performed 2 to 3 hours postinjection with a pinhole collimator as well as SPECT and SPECT/CT. DMSA is used to evaluate renal viability, pseudotumors such as columns of Bertin, cortical defects, and scaring from reflux and acute pyelonephritis.
Tc-99m-MAG3 (5 to 10 mCi, 185 to 370 MBq) is the most commonly used radiopharmaceutical for renal imaging. MAG3 is essentially 100% secreted within the tubules and has high extraction efficiency. MAG3 is highly protein bound, which results in improved count statistics and excellent target-to-background ratio while using a lower dose. MAG3 is commonly used to assess blood flow, parenchymal function, and excretion. MAG3 can also be seen in the liver and the gallbladder, especially in patients with decreased renal function.
Tc-99m-GH (10 to 20 mCi, 370 to 740 MBq) is a radiolabeled carbohydrate that has unique pharmacokinetics, although it is rarely used. Approximately 80% of the injected dose is cleared into the urine through glomerular filtration, whereas the remainder is bound in the renal tubules, making this agent useful for simultaneous renal cortical imaging and functional assessment.
Image Acquisition
Historically, the strength of radiopharmaceutical renal imaging was not high-resolution anatomic imaging, but rather functional and dynamic imaging of the genitourinary (GU) system. Recent advances in gamma camera technology, SPECT, and SPECT/CT imaging now bring more information to the clinical scenario and subsequently the diagnosis. Imaging acquisition matrices of (64 × 64) or (128 × 128) and the computer or camera zoom should be chosen such that the resultant images include all desired structures and the pixel size is approximately one half the resolving capacity of the system at the distance of the organ of interest from the camera. Recent computer technology and the commercial processing packages allow for the acquisition and rapid processing of large volumes of dynamic functional data and curve generation that is essential in the evaluation of the renogram study, the assessment of collecting system obstruction, renal transplant function, renovascular hypertension, and prostate-specific membrane antigen studies in prostate cancer patients (ProstaScint®).
The Consensus Reports on both diuretic renography and the scintigraphic evaluation of renovascular hypertension should be read by those unfamiliar with these studies, as their advice on study performance and interpretation, avoiding artifacts, performance, and interpretation pitfalls is sound, and because of their excellent explanations of patient preparation, pathology, and physiology.
Imaging Acquisition Techniques. In all renal studies, it is critical that the patient remain still during acquisition. As always, pediatric patients can present a challenge. Patient and parent reassurance, skilled technologists, and the occasional use of immobilization devices are usually sufficient to obtain a high-quality study. Occasionally, either sedation or the need for image reregistration is necessary. The supine position is used routinely. The more physiologic upright sitting position or semi-upright positions may be used, especially when ureteral drainage is evaluated or where renal pelvic activity might interfere with the study (e.g., evaluation for renovascular hypertension or the assessment of collecting system obstruction). The main drawback to nonsupine positions is in mobile kidneys that may move during the study and could adversely affect differential function determinations. Renal transplants and some renal variants (e.g., horseshoe kidney) and the urinary bladder are best imaged anteriorly. When dynamic imaging studies are performed, the injection site should be imaged, because even a small amount of infiltrated dose can affect excretion and uptake quantitative analysis.
Adequate hydration is mandatory to avoid delayed excretion and washout of the radiopharmaceutical, which may simulate obstruction. Also, false-positive renovascular hypertension studies can result. Many departments perform IV hydration and supplemental oral hydration routinely on all children and many adults because of the wide variability in the state of hydration upon presentation for the procedure.
Quantitative Analysis and Interpretation
Effective Renal Plasma Flow (ERPF) and Glomerular Filtration Rate (GFR). Plasma-based and camera-based methods of measuring ERPF and GFR are available. Plasma-based studies are deemed more accurate; however, they require great attention to detail from expertly trained technologists. Camera-based study calculations require measurements of the radiopharmaceutical syringe before injection and counts over the kidneys after injection. Commercially available software can be used to simplify corrections for patient and acquisition variables.
ERPF is most commonly measured using Tc-99m-MAG3. Excellent results are achieved for plasma-based measurements once corrected for differences in extraction fraction. A single blood sample protocol is used, obtained at 45 minutes postinjection. This time point represents full radiopharmaceutical distribution in the body and blood pool, and any subsequent reduction in activity should only reflect renal clearance. Tc-99m-DTPA samples are drawn at 60 and 180 minutes after injection secondary to the slower clearance of tubular agents. Camera-based ERPF calculations are not as accurate as plasma studies; however, they are highly reproducible and deemed reliable for clinical use.
GFR plasma-based studies utilize Tc-99m-DTPA and are well suited for a precise GFR measurement. However, measurements by this technique do slightly underestimate GFR, secondary to the protein binding, and are thus less accurate. Normally, GFR is in the range of 100 mL/minute for adults. Pediatric GFR values should reach adult levels by 2 years of age, and all values should be corrected for body surface area. In patients with markedly diminished GFR (<25 mL/minute), the results of this method are less accurate. Camera-based GFR studies capture the dynamic flow phase postinjection, followed by static images for 20 minutes. Postprocessing includes background subtraction of counts and correction for attenuation effects.
Blood flow, uptake, and clearance form the basis of most functional renal imaging. When used with or without other pharmaceuticals, numerous conditions are well evaluated including hydronephrosis/obstruction, acute and chronic renal failure, renal transplant, ischemic nephropathy (acute tubular necrosis [ATN]), RAS, trauma, and surgical complications.
Renal perfusion imaging is performed using a bolus intravenously injection of the radiopharmaceutical and the subsequent acquisition of images at a rate of 1 to 3 seconds per frame for 60 seconds. This is followed by imaging at 60 seconds per frame for 25 to 30 minutes, to capture the peak perfusion and subsequent washout of the radiopharmaceutical from the parenchyma. Cinematic perfusion and uptake and washout images are provided electronically. Static sequential images can be substituted as needed.
A plot of the time-activity curves (TAC) of both kidneys, obtained in the posterior projection of the supine patient, allows accurate relative comparison of the blood flow and function of both kidneys. A separate plot of the blood flow phase and the uptake-clearance phase are usually provided. In a normal study, the aorta is visualized 2 to 5 seconds before the kidneys. A steep uptake phase, lasting 1 to 2 minutes, is followed by a distinct peak during cortical perfusion, which normally occurs between 3 and 5 minutes. Clearance of the radiotracer from the cortex/parenchyma follows, there is normally less than 50% of peak activity by 7 to 10 minutes. Collecting system and bladder filling and clearance occur immediately following the cortical/parenchymal phase. Overlapping collecting system counts will interfere with measurements of the excretory phase of imaging. Whole kidney ROIs (range-of-interest) are drawn before collecting system activity, or renal cortical ROIs are used when needed.
Various background corrected parameters are measured and reported, most commonly the time to peak cortical activity (normally 3 to 5 minutes). T1/2 washout time, which represents the time to clear 50% of the peak cortical activity (normally 7 to 10 minutes). Percent clearance at 20 minutes (normally >70%), 20-minute activity/peak activity ratio (20/peak) (normally in the range of 0.30), and the 20-minute/3-minute activity ratio (20/3) (normally in the range of 0.30). These values quantify the renal function and will increase as renal function deteriorates, making them useful in serial exams. Differential function of the two kidneys is reported using the whole kidney ROI, before collecting system visualization. Normally each kidney contributes 45% to 55% of total renal function. A kidney contributing less than 40% is considered abnormal.
The following Tc-99m renal agents are used for this common study.
Because the clearance of MAG3 (300 to 400 mL/minute) (extraction efficiency = 60%) is so much greater than that of DTPA (80 to 140 mL/minute) (extraction efficiency = 20%), MAG3 is the agent of choice for imaging kidneys in moderate to severe renal failure, immature kidneys, and in transplant kidneys where renal function is often in flux (Fig. 59.1).
MAG3 in a normally functioning kidney will demonstrate the following ranges:
Time to peak: 3 to 5 minutes.
T1/2 = 7 to 10 minutes.
Collecting system: 5 minutes.
Bladder: 10 to 15 minutes.
In practice, because glomerular function and tubular function generally parallel each other, MAG3 has replaced DTPA in many clinics, unless glomerular function analysis is specifically requested or comparison with legacy DTPA exams is needed.
Clinical Applications
Anatomic Variants. Nuclear renal imaging is of use in evaluating anatomic variants when other imaging modalities have not fully defined the abnormality. The ability to assess function makes scintigraphy even more valuable. A dromedary hump, a fetal lobulation, or a renal column of Bertin may appear as a mass on an US, CT, or MRI. DMSA or GH images (pinhole and/or SPECT) will demonstrate the questionable tissue to be normally functioning and rule out a pathologic lesion. Nuclear imaging is particularly useful in congenital abnormalities (Fig. 59.2) such as horseshoe kidney, lump or cake kidney, and crossed-fused ectopia and solitary kidney when other modalities have not located or explained the functional nature of the abnormality.
Mass Lesions. Although radionuclide studies are not generally used to detect solid intrarenal masses, they may be of value in investigating masses detected on US, CT, or MRI DMSA or GH can distinguish functional from nonfunctional renal tissue and are useful in pseudotumors such as columns of Bertin, fetal lobulations, or dromedary humps, all of which show normal functional renal tissue (Fig. 59.3). In the neonate, multicystic dysplastic kidney and hydronephrosis both present as fluid-filled masses that can be differentiated by the presence or the absence of radiotracer in the fluid collection(s). With MAG3 or DTPA imaging, urine collections such as hydronephrosis or urinoma increase tracer concentration with time. Photopenic lesions may represent cysts, abscess, neoplasm, hematoma, or infarcts.
Cortical Imaging. Although both Tc-99m-DMSA and Tc-99m-GH can be used for cortical imaging, DMSA is the cortical agent of choice. When the usual dose of DMSA is used, this results in excellent target-to-background ratio and minimal interference of excreted radiopharmaceutical with analysis of the kidney cortex. Acute pyelonephritis in a pediatric patient can occur from reflux of infected urine and is often evaluated using renal scintigraphy. Cortical defects may represent infection, single or multiple ill-defined lesions, scaring, localized with sharp margins or a neoplasm. Follow-up studies of acute pyelonephritis will show resolution or significant improvement in 6 months (Fig. 59.4).
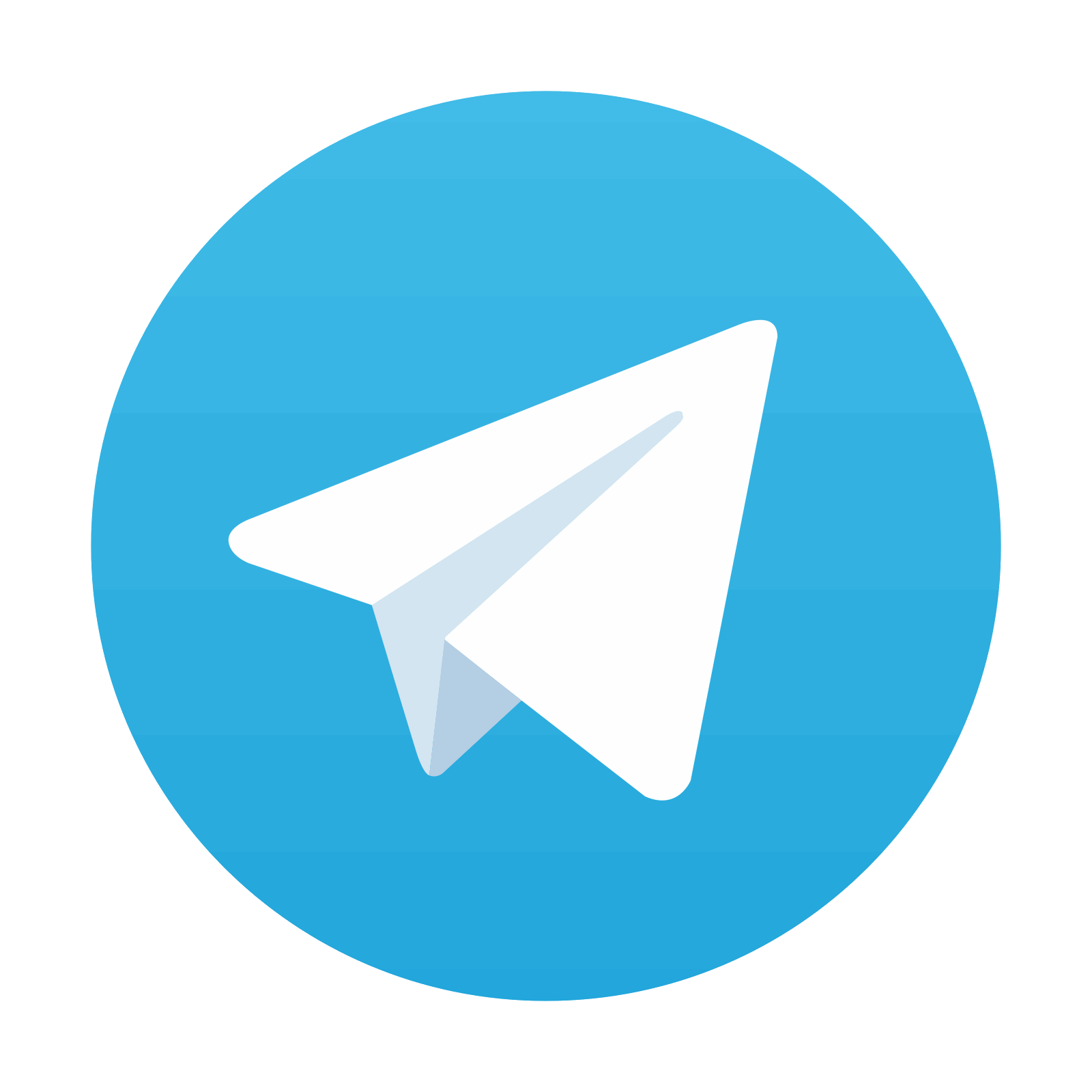
Stay updated, free articles. Join our Telegram channel

Full access? Get Clinical Tree
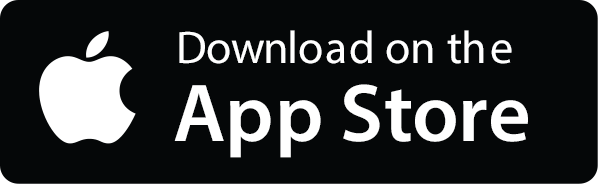
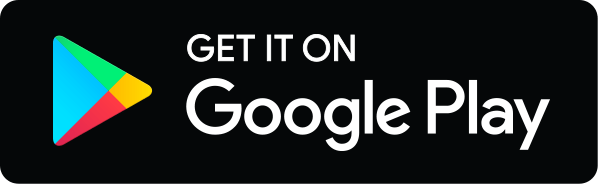