Fig. 4.1
Time sequence of radiobiological process after ionization radiation
4.1.2 Direct and Indirect Actions of Radiation
Biological effects after exposure of radiation are divided into two categories, direct action and indirect action of radiation by the very early process of the energy deposition (Fig. 4.2). DNA molecules can receive energy directly from the secondary electrons produced by the incident radiation, resulting in their ionization, and are damaged by cleavage of the chemical bonds. This direct action of radiation accounts for approximately 1/3 of all biological effects after radiation. DNA molecules can also be damaged by active group of molecules (free radicals) produced by ionization of the surrounding water molecules. This is an indirect action of radiation and accounts for the remaining approximately 2/3 of the biological effects. The efficiency of free-radical production is expressed as a G-value, which is defined as the number of free-radical molecules produced per 100 eV of absorbed energy. The values are obtained as the final result of chain reactions. This process mainly consists of the chemical reactions of water molecules with the body constituents and can be affected by the surrounding various free-radical scavengers. These active species deprive a biomacromolecule (R) of a hydrogen atom (dehydrogenation) to make a radical R; the radical R binds with a hydroxyl radical (OH·) or reacts with another radical biomolecule to compose a new molecule. Consequently, the active species cause various reactions (Fig. 4.3).
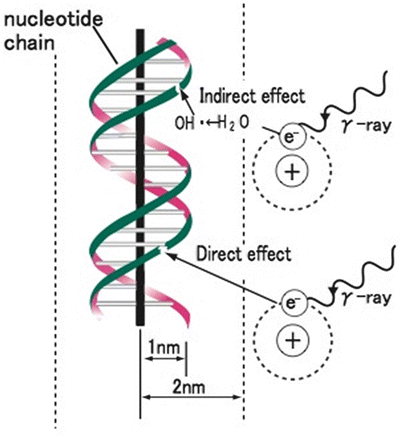
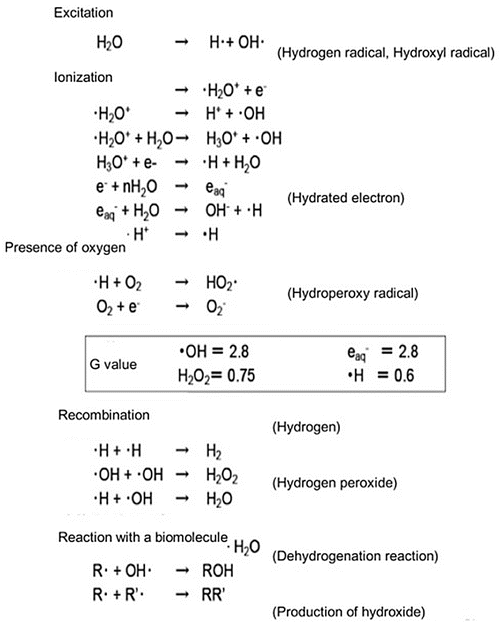
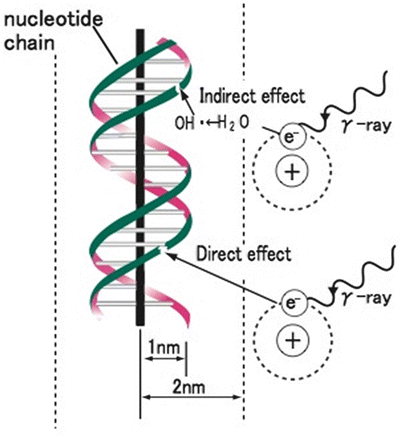
Fig. 4.2
Direct and indirect actions of radiation. Indirect action (upper), the secondary electron interacts with, for example, a water molecule to produce a hydroxyl radical (OH·), which produces a damage to the DNA. Direct action (lower), a secondary electron resulting from absorption of a photon interacts with the DNA to produce damages. Modified from [1]
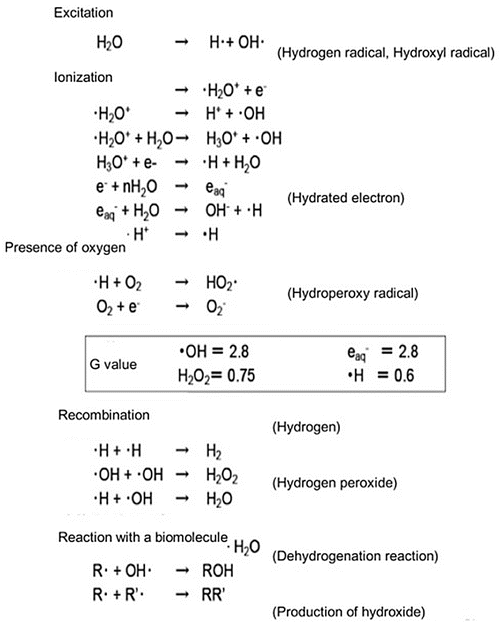
Fig. 4.3
Radiation chemical processes of water molecules after ionization radiation and the efficiency, G-value of major products
4.1.3 Direct and Indirect Actions of Radiation
Low-LET radiations (photon) show a uniform, sparse spatial distribution of ionization in cells. High-LET particles bring about a dense ionization along their track through energy deposit to the medium, showing distributions called track structures (Fig. 4.4). Charged-particle beams that form the Bragg’s peak in matter change the ionization density along the traveling direction, showing complexities.
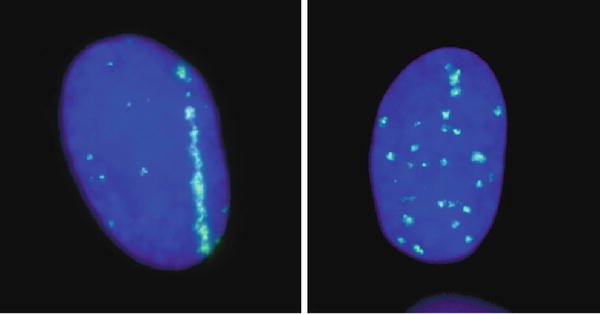
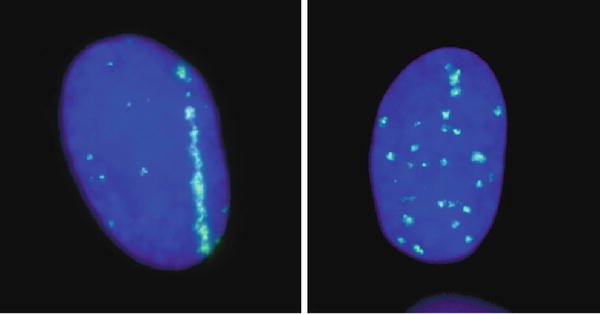
Fig. 4.4
Biological track structure of heavy-ion traversal in cell nuclei. DNA double-strand breaks were visualized after very high-LET horizontal iron-ion beam (left) and X-rays (right). Clear structure after iron beam traversal was observed, but no such structure for X-rays
Various physical and chemical processes are involved in the biological effects as direct and indirect effects of radiations and details of these processes can be found in other materials [2]. Particle beams have more different characteristics than photon beams that originate from the differences in their ionization-density distributions. Unlike low-LET photon beams, for example, high-LET particle beams show an ionization density higher than necessary for cell killing in some area in matter and also produce concentrated damages such as multiple DSBs or complex lesions. Thus, particle beams have different and severe biological effects from those of low-LET radiations.
4.2 Biological Elementary Processes
The organs comprising a human body are composed of tissue, which is a collection of cells with various functions to achieve specific objectives. The impact of ionizing radiations on a human body is initiated in the cells. Ionizing radiations delivered to a human body have the most serious impact on the genes that contained cell nucleus or DNA molecule. DNA damage causes the transmission of erroneous information, makes cell growth difficult unless repaired, and produces abnormal conditions such as mutations or carcinogenesis. This damage in a cell impairs functions of the organ as damage to the tissue, eventually influencing the individual. A DNA molecule consists of a pair of backbone chains with the four (ATGC) nucleobases, which are involved in the encoding of genetic information. DNA is a long molecule encoding a huge amount of genetic information. The DNA contained in one human cell weighs approximately 6 pg, and the human genetic information is written as 4-bit data in approximately 32 hundred million base pairs.
A process of cell proliferation is carried out through a “cell cycle” sequence consisting of the four (M/G1/S/G2) phases. Certain types of cells are usually proliferating by repeatedly dividing to supply functional cells. In contrast, many somatic cells (nonreproductive cells) usually stay in another G1-like interphase (G0 phase) without proceeding to the S phase, performing their own functions.
4.2.1 DNA Damages
The most important biological damage of cells after exposure of ionizing radiation is believed to be DNA damage. Many kinds of DNA damages will generate in a cell [3]. Damages or losses of nucleotide bases and DNA single-strand breaks (SSBs) are potentially to be repaired without errors of genetic information because of the existing right genetic information in the complementary DNA strand. Numbers of double-strand breaks (DSBs) are also rejoined to reconstruct DNA strand by the nonhomologous end joining (NHEJ) and homologous recombination (HR) systems. Damaged site is reconstructed by using the genetic information in complementary strand of sister chromatid by the HR system and shows error-free repair. Some nucleotide bases near the damage site are removed to clean up the damaged end, rejoin the strands without correct genetic information in the NHEJ process; thus it will be an error-prone rejoining. In addition, productions of complex lesion of DNA breaks are expected for heavy-ion exposure, because the density of ionization must be very high at around the traversal of the ion.
When cells are exposed to ionizing radiation, DNA damages in the form of SSBs, DSBs, base damage, or their combinations are frequent events. It is known that the complexity and severity of DNA damage depend on the quality of radiation and the microscopic dose deposited in small segments of DNA, which is often related to the linear transfer energy (LET) of the radiation. Experimental studies have suggested that under the same dose, high-LET radiation induces more small DNA fragments than low-LET radiation, which affects Ku binding with DNA end efficiently and might be a main reason for high-LET radiation-induced RBE since DNA DSB is a major cause for radiation-induced cell death. In this work, we proposed a mathematical model of DNA fragments rejoining according to NHEJ mechanism.
4.2.2 Chromosome Aberrations
One set of chromosomes in a human individual consists of 22 pairs of autosomes (any of the chromosomes in a cell other than the sex chromosomes) and two sex chromosomes, all of which are inherited from one’s parents. In the DNA synthetic phase of the cell cycle, one copy of the genetic information is created and associated with the original one as a pair of sister chromatids, bound at the centromere, in order that the same genetic information may be distributed to two daughter cells in the coming mitotic phase. In the metaphase of the mitotic phase, DNA, distributed homogeneously within a cell nucleus in other phases, condenses to form 46 structures that can be microscopically observed as chromosomes. In the anaphase, 46 pairs of sister chromatids are pulled apart to transmit the genetic information to the two daughter cells.
In the cells of which DNA has been damaged by radiation exposure, abnormality can be observed as a chromosomal aberration which is effective as an indicator of radiation exposure as it is easy to quantify and correlates well with radiation-caused cell death. Various types of chromosomal aberration (Fig. 4.5) are caused by radiations, depending on the phase of the cell when its DNA is damaged. If it occurs during the period from the first pause (G1 phase) to the early DNA synthetic phase (early S phase), the so-called chromosomal aberration can be observed, which appears to be caused by simultaneous cleavage of both of the sister chromatids. If a cleaved chromosome is not reunited, “terminal deletion” and “fragment formation” are observed. After a chromosome is cleaved at the two sites, the center section turns over and is then reunited with the remaining fragments. This phenomenon is called “inversion.” If the termini of this center section rejoin with one another, a “circular chromosome” is formed, with the fragments left over. After two chromosomes are cleaved, they can rejoin with each other to form a dicentric chromosome, which has two kinetochores (structures of protein associated with DNA located at the centromere region). This phenomenon is called “mutual translocation.” On the other hand, if DNA damage occurs late in the DNA synthetic phase (late S phase) or the second pause (G2 phase), chromatid aberration is observed, with damage showing on either of the sister chromatids (Fig. 4.5).
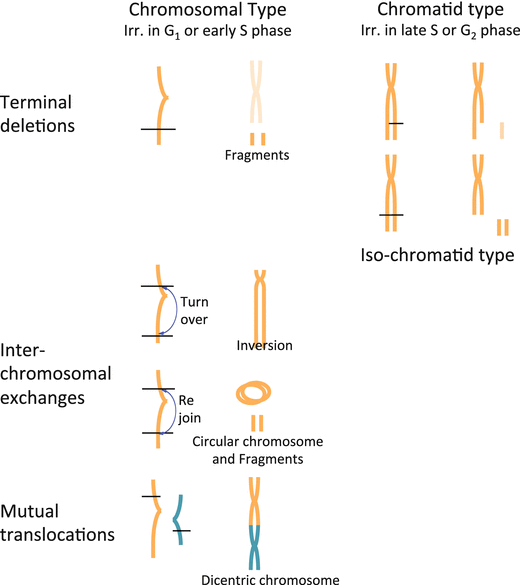
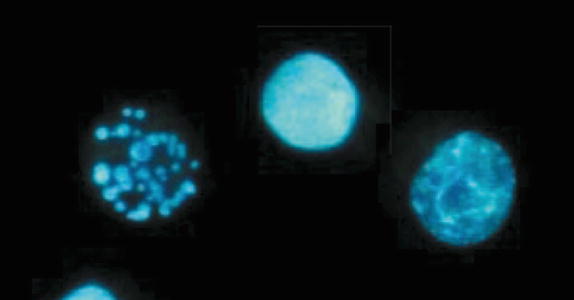
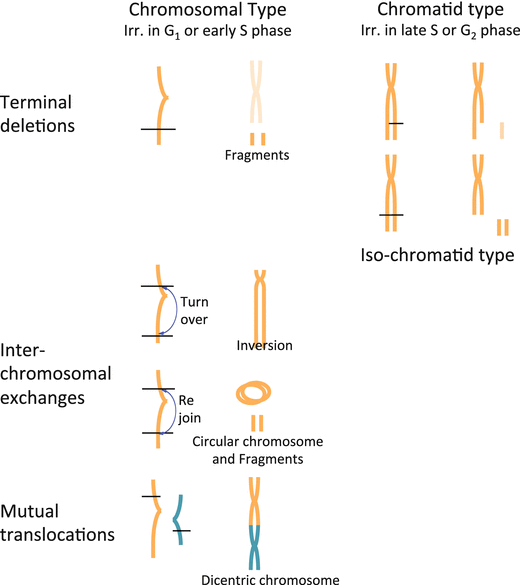
Fig. 4.5
Types of chromosomal aberrations. Many iso-chromatid-type aberration can be seen after high-LET radiation
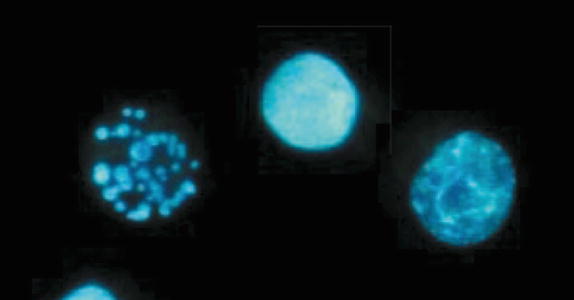
Fig. 4.6
Apoptotic cells. A finely fragmented (left), condensed (middle), and being fragmented (right) DNA
4.2.3 Cellular Effects
Many of the multiplied human cells undergo cell cycle about once a day, with one parent cell dividing into two daughter cells; the number of the cells doubles again and again. A tens-of-micron single cell proliferates into a macroscopic colony with a diameter of several millimeters after a dozen days or so. When these cells are irradiated, some of them die owing to the resulting damage, while others survive. The surviving cells can form a colony, but the colonies show different morphology from those made by unirradiated cells, and the number of colonies they form is usually smaller. There are several cell death modes, but they are generally classified into reproductive death and interphase death.
4.2.3.1 Reproductive Death
Even after irradiation, the surviving cells can continue proliferating; cells have the ability to repair damage to their DNA or chromosomes and many cells can remove these damage. After repair, the cell cycle goes into a temporary pause, which is called G1 block or G2 block. These additional pauses in the cell cycle result in mitotic delay, which is often observed for the first division after irradiation. Approximately 1-Gy irradiation often causes a 1-hour mitotic delay.
Reproductive death is the mode of death of the irradiated cells that have lost reproductive ability after several cell divisions. These cells may still retain ability to synthesize DNA and proteins. These cells, however, have various functional aberrations; for example, the nucleus divides but fail in cytokinesis (the last stage of cell division following the nuclear division, division of cytoplasm) and the cell grows but cannot divide or shows abnormal morphology. In radiobiological observations after irradiation, which aim at counting the reproductive cell deaths, a colony consisting of 50 or more cells (after 5 to 6 divisions) is regarded as a colony originating from a surviving cell. The cell survival rate is determined from the ratio of the number of colonies formed in a plate to the number of irradiated cells seeded in the plate.
4.2.3.2 Interphase Death
With this mode, irradiated cells die without dividing. Interphase death, therefore, cannot be determined by the colony formation method. The cells are usually observed by morphological methods such as the dye exclusion method and fluorescence staining. Apoptosis and necrosis are mostly known as active and passive interphase death.
Apoptosis is an interphase death occurring even at very low doses, often observed in lymphoid stem cells. It is an active programmed cell death and a mode to exclude unnecessary cells. Cells with irreparable damage to their genetic information are identified and actively excluded to make spaces into which the surrounding normal cells can proliferate, resulting in maintenance of the entire function they are involved in. Cells undergoing apoptosis become round and are divided into units called “apoptotic vesicles.” The nuclei are condensed, with DNA being cleaved into nucleosomes. The fundamental units of chromatin, the material of which eukaryotic chromosomes are made, consisting of cores of histone proteins around which are coiled about 160 bp of DNA. The divided cells are then scavenged by phagocytes such as macrophages, so that spaces for proliferation are created.
Necrosis is often observed in cells exposed to very high doses of radiations where the cells lose their functions, melt or break, and die. Necrosis is observed in nerve, muscle, and other cells, but unlike programmed cell death such as apoptosis, necrosis is not accurately controlled and means the passive death of nonfunctional cells (Fig. 4.6).
4.3 Models for Radiobiological Effects
In many cases, the biological effects of ionizing radiations are determined from the exposure dose. These effects can be expressed as a function of the dose, with the dose-effect relationship shown graphically, which enables these effects to analyze quantitatively. Plotting the rate of surviving cells against the irradiation dose can draw survival curves, and models to analyze these curves have been proposed with which the effects of ionizing radiations can be quantitatively studied.
4.3.1 Target Theory
In order to quantitatively describe the properties of radiobiological effects using survival curves, models to explain the shapes of these curves have been proposed. The target theory is the most classical model. This model assumes “parts of a cell are sensitive to ionizing radiations and regarded as targets for radiations”; “the targets are much smaller than the entire cell, but essential for survival of the cell”; and “when these targets are hit by radiations, the cell can lose its functions to become inactivated (killed).” This is the idea of “all or nothing”; cells can survive, unless they are hit. The linear quadratic model takes the place of this theory now especially in the field of radiotherapy.
4.3.2 Linear Quadratic Model
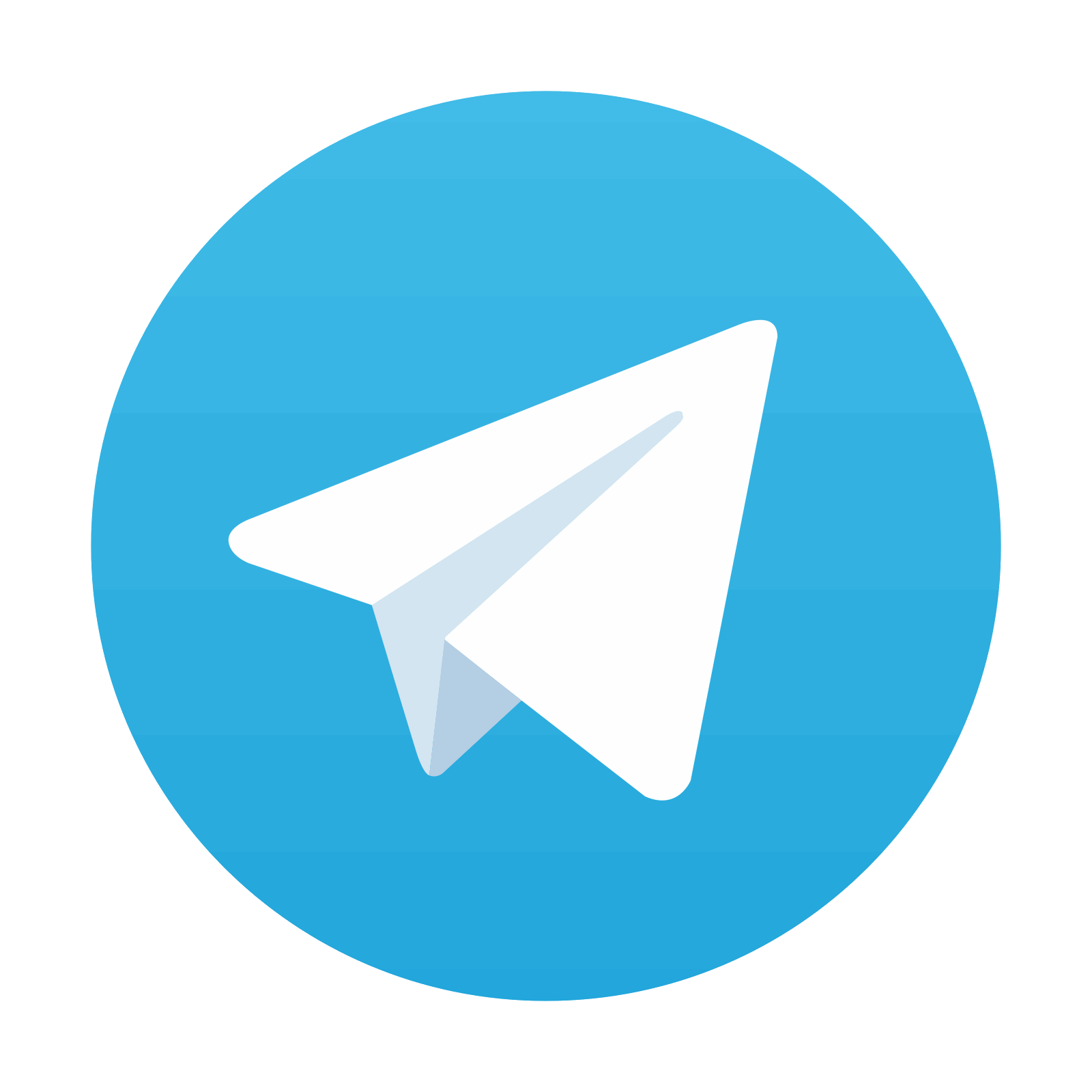
Stay updated, free articles. Join our Telegram channel

Full access? Get Clinical Tree
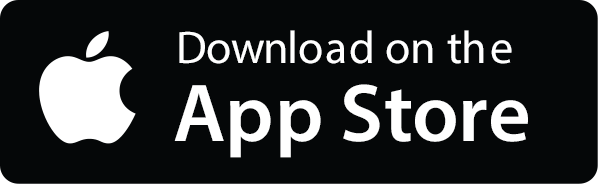
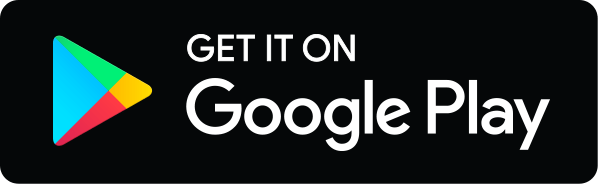