High-quality MR imaging of the hepatobiliary system is increasingly being used to evaluate focal and diffuse liver disease in children. Advances in magnetic resonance hepatobiliary imaging including magnetic resonance cholangiopancreatography, elastography, and iron quantification provide valuable noninvasive information about the bile ducts and hepatic parenchyma. This article discusses up-to-date MR imaging techniques and imaging findings of common diseases of the pediatric hepatobiliary system.
Key points
- •
High-quality MR imaging of the hepatobiliary system is increasingly being used to evaluate focal and diffuse liver disease in children.
- •
Advanced methods in MR imaging of the hepatobiliary system provide valuable noninvasive information including magnetic resonance cholangiopancreatography, elastography, and iron quantification.
- •
MR imaging is a powerful tool in evaluating hepatobiliary disease in the pediatric population.
Introduction
Magnetic resonance (MR) imaging is commonly used for evaluation of liver diseases in pediatric patients because of its lack of ionizing radiation and its ability to provide comprehensive evaluation of liver diseases, focal liver lesions, and the biliary system. MR imaging technology continues to advance with improvements in motion compensation techniques, assessment of contrast enhancement, and better characterization of liver disease. Sequences such as elastography and iron quantification can provide additional information about the hepatic parenchyma and may help avoid the need for liver biopsy.
MR imaging in the pediatric population has its challenges because proper patient preparation, the need for sedation, and appropriate coil selection are important factors that must be carefully considered to ensure an adequate examination. Up-to-date working knowledge of techniques and tools available to perform MR imaging of the hepatobiliary system and familiarity with common disease processes that are encountered in the pediatric population are essential. This article provides a review of current imaging techniques and a review of common imaging manifestations of hepatobiliary diseases in pediatric patients.
Imaging techniques
Patient Preparation and Equipment
Patient preparation is vital to ensure optimization during imaging in pediatric patients. Each MR examination must be tailored to the age, size, and expected level of cooperation of the child. Patients should have nothing by mouth for 4 hours before the examination to minimize bowel peristalsis artifact and make certain the gallbladder is distended. When available, a child life specialist may meet with the patient and parents beforehand to explain what to expect from the examination and answer questions. Practicing breath-hold instructions and providing these instructions with consistency helps to improve image quality. If power injection of contrast is needed during the examination, adequate peripheral venous access should be obtained.
Sedation
The need for sedation or anesthesia should be carefully considered. Children older than 6 years should be evaluated on a case-by-case basis to determine whether sedation is warranted. Usually children younger than 6 years require some degree of sedation to reduce the amount of voluntary motion during the scan. MR imaging–compatible goggles allowing patients to watch movies during the examination can be used in children between the ages of 5 and 8 years of age to help reduce the need for sedation. Neonates (<3 months of age) can frequently be imaged without sedation using the “feed and wrap” method whereby the child is fed, tightly swaddled, and laid on the scanner table with hearing protection in place.
Hardware
Both 1.5-tesla (1.5T) and 3T field strength magnets can be used, although smaller patients may benefit from the higher signal in 3T machines. The smallest possible radiofrequency coil that covers the anatomy of interest should be selected. Typically, 16- to 32-channel phased-array body coils are used. Smaller coils, such as cardiac arrays, work well for most children, although very large patients may need a torso array.
Standard Sequences
Standard sequences included on most MR imaging examinations of the hepatobiliary system include T2-weighted images (T2WI) in the axial and coronal planes, balanced steady-state free precession (SSFP) images, and in-phase and out-of-phase imaging. The field of view, slice thickness, and matrix are parameters that must be adjusted for each individual patient size and clinical question.
T2-weighted sequences
T2WI of the liver can be done with fast-spin echo (FSE), volumetric, single-shot fast-spin echo (SSFSE), or short inversion time inversion-recovery sequences. Conventional T2WI of the hepatobiliary system can be acquired via two-dimensional (2D) T2-weighted, fat-suppressed FSE in the axial plane using respiratory-triggered or navigated sequences. These FSE images provide better evaluation of abdominal anatomy with higher signal-to-noise ratio (SNR) than SSFSE. This sequence allows good visualization of liver contours and is helpful in evaluating the intensity of the T2 signal of intrahepatic lesions; a useful piece of information when evaluating focal liver lesions. Echo times (TEs) for 1.5T are usually 80 to 90 milliseconds and around 70 to 80 milliseconds for 3T. Because of the required acquisition time and breath-hold needed for this sequence, it may be prone to respiratory motion artifact if not optimized.
2D SSFE T2WI can be performed with a breath-hold or can be manually triggered by the technologist for children with irregular breathing patterns. These are typically acquired more rapidly than the conventional FSE T2WI and can be helpful when respiratory motion artifact is present. SSFSE images are usually acquired in the coronal and axial planes.
Rapid acquisition and motion insensitivity are some of the advantages of balanced SSFP images. In addition, bile ducts, vascular structures, and fluid collections are all hyperintense with this sequence, and can serve as a problem-solving sequence when other sequences are degraded by motion artifact.
In-phase/out-of-phase
In-phase and out-of-phase images are essential when evaluating the liver to detect fat or iron deposition. The out-of-phase image should be acquired on the first echo, therefore resulting in signal loss on out-of-phase images as fat, and signal loss on in-phase images as iron. These images can usually be done with a breath-hold with older cooperative patients and with free breathing in younger patients. Note that the echo times are slightly different on 1.5T (2.3 milliseconds out-of-phase and 4.6 milliseconds in-phase) and 3T (1.15 milliseconds and 2.3 milliseconds).
Additional Noncontrast Sequences
T1-weighted images/spoiled gradient
Precontrast three-dimensional (3D) T1-weighted gradient-echo or spoiled gradient images are often helpful for anatomic visualization and comparison with postcontrast images. These offer rapid volumetric acquisition in a single breath-hold with high resolution. The disadvantage to this approach is lower SNR than traditional T1-weighted imaging (T1WI).
Diffusion-weighted imaging
Diffusion-weighted imaging (DWI) is a complementary sequence to T2WI and reflects the degree of cellularity of a tissue. As such, qualitative restricted diffusion within a focal liver lesion is a good predictor of malignancy and can be helpful in distinguishing between malignant and benign lesions. In addition to detection and characterization of focal lesions, it can be used in the assessment of cirrhosis because parenchyma with fibrosis or cirrhosis also shows restricted diffusion. DWI is a rapid acquisition sequence that is relatively robust to motion artifact but has low resolution.
MR cholangiopancreatography
MR cholangiopancreatography (MRCP) has been shown to be an effective noninvasive method of visualizing pancreaticobiliary disease in pediatric patients with a diagnostic accuracy of around 90% to 100%. MRCP consists of FSE heavily weighted T2WI with long TEs (up to 1000 milliseconds) to allow visualization of the pancreatic and biliary ducts. The coronal and axial imaging planes are typically used with either a breath-hold or with free breathing using respiratory gating. These can be obtained as thin-slice 2D or 3D and can then be reconstructed into maximum-intensity projection (MIP) images. 3D images are usually obtained in the coronal plane whereby MIP reformats can then be made in any plane. Coronal images are also useful as a global assessment of the ducts because many ducts can be seen in a single image. The axial plane is optimal for evaluation of tiny calculi or ductal filling defects, and visualization of the pancreatic duct. The addition of intravenous secretin (to distend the pancreatic duct), and negative oral contrast (to reduce bowel signal) have both been described as supplemental options.
Elastography
MR elastography (MRE) is a noninvasive technique used to assess liver stiffness, which is a surrogate for liver fibrosis. MRE assesses mechanical stress or shear waves as they propagate across the liver parenchyma. The wave data generate a quantitative map of tissue stiffness in kilopascals (kPa), which provides quantitative data of the liver stiffness or fibrosis. It is important to ensure that regions of interest (ROI) are selected appropriately to avoid large blood vessels and regions of low amplitude to thus allow an accurate estimate of liver stiffness ( Fig. 1 ). Technical issues related to use of MRE have been described, but a high technical success rate has been shown despite these issues.
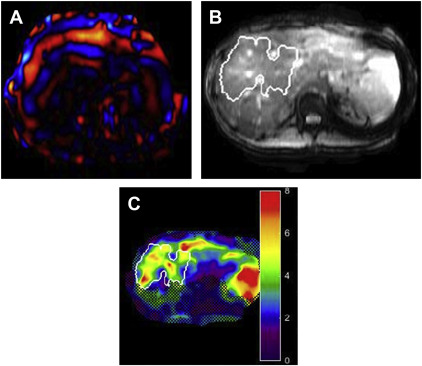
Iron quantification
Hepatic deposition of iron can occur in disease processes, such as hemochromatosis, beta-thalassemia, or excessive blood transfusions. The iron causes local distortion in the magnetic fields, particularly affecting the T2* transverse relaxation, causing loss of signal intensity that is proportional to the iron deposition. MR iron quantification is a useful and noninvasive way to quantify the amount of hepatic iron deposition with high correlation to biopsy samples. In addition, MR iron quantification helps determine the severity of disease and monitor therapy with high sensitivity, specificity, and positive and negative predictive values. Techniques for performing MR imaging of hepatic iron quantification in pediatric patients have been well described.
Contrast selection
Both extracellular and hepatobiliary gadolinium-based agents are available and commonly used in hepatobiliary imaging. The decision of which contrast agent to use should be based largely on the clinical indication. Extracellular contrast agents are the most commonly used agents and are excreted via the kidneys. To evaluate liver parenchymal enhancement and hepatic vasculature, and for characterization of most hepatic tumors, extracellular agents work well. If there is suspicion that a focal hepatic mass may represent an area of focal nodular hyperplasia (FNH), a hepatobiliary agent is often selected, as FNHs retain hepatobiliary agents on delayed (10- to 20-minute) images and can thus be distinguished from other focal liver tumors. Hepatobiliary agents are excreted both renally and hepatically and create contrast-enhanced functional and anatomic MRCP-type images. Hepatobiliary agents have poor hepatic uptake and excretion in the setting of poorly functioning hepatocytes (bilirubin level >3 mg/dL) and may not be effective in these patients.
Dynamic contrast imaging
Dynamic imaging allows the evaluation of contrast in multiple phases through the vasculature and tissues, which in turn can help in identifying vascular anatomy and variants in addition to better tumor characterization.
Imaging sequences
Typically, a 3D gradient-echo sequence (THRIVE; Philips, VIBE; Siemens, LAVA; GE Healthcare) is used in the setting of low breath-hold times of 15 to 20 seconds and relatively quick acquisitions in various phases. These can be performed with free breathing in smaller children. As these images are 3D or volumetrically acquired, they can be reconstructed in additional planes if needed. Radial filling of k-space with free-breathing 3D gradient-echo sequences, such as StarVIBE (Siemens Healthineers), may be used and help to diminish respiratory motion artifact.
Timing of phases
Typically, images are obtained during an arterial, portal venous, and equilibrium phase. Additional delayed series can be obtained as needed. Timing of these phases is more variable in children than in adults because of variability in age, size, and intravenous catheter size. Methods for timing include fluoroscopic triggering from the abdominal aorta, a best-guess estimate, or by using a calculated delay. If a hepatobiliary agent is used for contrast, the standard dynamic postcontrast images described earlier are usually used with addition of 10- to 20-minute delay images.
Intrinsic liver disease
Congenital and Genetic Disorders
Autosomal recessive polycystic kidney disease
Autosomal recessive polycystic kidney disease (ARPKD) is the most common heritable cystic renal disease in childhood. Patients with ARPKD always have some degree of congenital hepatic fibrosis (CHF), and the degree of each organ involvement is usually inversely proportional to one another, with children with mild renal disease having worse hepatic fibrosis ( Fig. 2 ). Cysts in both the liver and kidneys appear hypointense on T1WI and hyperintense on T2WI, with no enhancement of the cysts on postcontrast images. Additional imaging clues of ARPKD/CHF include atrophy of the right lobe and hypertrophy of the left lateral segment and caudate lobe. Small left medial segments are rarely seen in CHF and are often normal and enlarged, and can help distinguish CHF from cirrhosis. Patients with CHF can have enlargement of the hepatic artery with associated large multiacinar regenerative nodules.
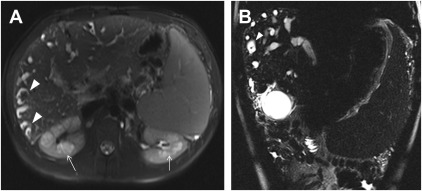
Glycogen and lipid storage diseases and mucopolysaccharidoses
Glycogen storage diseases (GSDs) are a group of metabolic disorders caused by an inborn error of a particular enzyme that affects the production or breakdown of glycogen. There are multiple subtypes, many of which have associated eponyms including von Gierke (type 1a) and Pompe (type II). Most types result in hepatomegaly evident on imaging. MR imaging can be performed to provide liver volumes to monitor progression of the disease, thus providing accurate hepatic size and avoiding radiation from computed tomography (CT). GSDs have also been noted to have an increased prevalence of hepatic adenomas, particularly subtypes Ia, Ib, and III ( Fig. 3 ). In addition, types Ia and Ib may undergo malignant transformation to form hepatocellular carcinomas, whereas types III and IV tend to progress to cirrhosis.
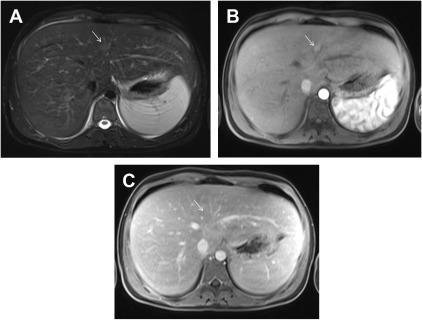
Lipid storage disorders represent a diverse group of diseases that includes Gaucher, Niemann-Pick, Fabry, Schindler, Krabbe, Farber, and Wolman diseases, and metachromatic leukodystrophy. These are related by their deficiency of lysosomal hydrolase, which leads to abnormal lysosomal accumulation of sphingolipids. The main deposition location of these sphingolipids is the central nervous system, which leads to a neurodegenerative process; however, the visceral accumulation of these substrates leads to organomegaly resulting from lipid and cholesterol deposition. This progresses to cirrhosis and liver failure before adulthood. MR imaging of the abdomen shows hepatomegaly with hepatic steatosis on chemical shift imaging, and ultimately nonspecific findings of cirrhosis and portal venous hypertension with disease progression.
Mucopolysaccharidoses are diseases caused by enzymatic activity in the breakdown of glucosamine glycans, leading to excess tissue deposition of mucopolysaccharides. Hurler (type I), Hunter (type II), Sanfilippo (type III), Maroteax-Lamy (type VI), and type VIIb specifically have hepatic manifestations with hepatomegaly, fibrosis, and cirrhotic patterns.
Wilson disease
Wilson disease is a rare autosomal recessive disorder of copper metabolism, which results in excess copper deposition in the liver secondary to an inability to remove excess copper from the liver to the plasma and bile. This leads to oxidative damage and can lead to acute hepatitis, chronic active hepatitis, fibrosis, and cirrhosis.
Imaging findings on MR imaging are nonspecific but include fat deposition and a nodular cirrhotic appearance of the liver. A prominent perihepatic fat layer has been described with Wilson disease, although this is not pathognomonic. Although copper deposition increases the liver attenuation on CT, it does not change the appearance of the liver on MR imaging because copper has no ferromagnetic effect. Given that there are no specific findings of Wilson disease on MR imaging, it should be used to evaluate for complications of the disease such as cirrhosis and not for the diagnosis.
Acquired Disorders
Steatosis
Hepatic steatosis, or nonalcoholic fatty liver disease (NAFLD), is the most prevalent form of chronic liver disease in pediatric patients, affecting approximately 10% of children and adolescents. This occurs when excess fat is accumulated and deposited in the liver, and often is a marker of other underlying metabolic disorders such as insulin resistance and obesity. NAFLD encompasses a spectrum of clinico-histopathological changes including simple steatosis (fat accumulation), steatohepatitis or nonalcoholic steatohepatitis fat accumulation with accompanying inflammation, and eventually fibrosis and cirrhosis. Different patterns of fat deposition have been described including diffuse homogeneous, diffuse heterogeneous, focal, and multifocal deposition patterns.
MR imaging is commonly used as a definitive tool to evaluate for the presence of steatosis in the liver. This is obtained with in-phase and out-of-phase T1-weighted gradient-echo images. Signal loss on the out-of-phase image indicates the presence of fat ( Fig. 4 ). In addition to the identification of focal or diffuse steatosis, MR imaging can also be used to assess for complications of NAFLD, including fibrosis (via elastography) and cirrhosis.
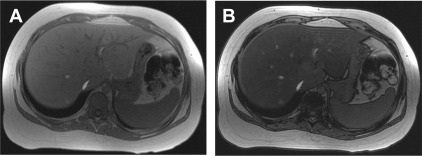
Siderosis
Siderosis, or excessive iron deposition in organs, occurs in the liver either from a primary iron overload (inherited) or secondary overload mechanism. Primary iron overload, also known as hereditary hemochromatosis, is an autosomal recessive disorder that results in a defective protein causing increased intestinal absorption of iron from the gut. Secondary overload syndromes include iron-loading anemias (sickle cell disease, thalassemia, sideroblastic anemia, chronic hemolytic anemia, aplastic anemia, and pyruvate kinase deficiency), chronic liver diseases (viral hepatitis, alcoholic fatty liver disease, and NAFLD), and complications of blood transfusion or hemodialysis.
MR imaging is useful in the detection and quantification of hepatic iron deposition using both in-phase/out-of-phase imaging and T2* measurement. Hemosiderin/iron deposition results in signal loss on sequences with longer echo times. Thus, iron deposition is seen as lower signal intensity within the liver on in-phase images (the opposite of fatty deposition as discussed earlier) secondary to the local field inhomogeneity caused by the iron, and can be used to quantify hepatic iron as discussed earlier ( Fig. 5 ).
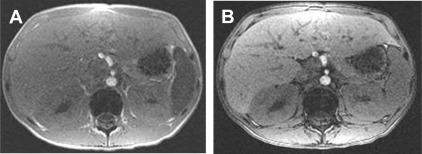
Infarction
Hepatic infarction is rare because of the dual blood supply of the liver via the hepatic arteries and portal vein systems. However, infarction may occur in the setting of acute shock/hypoperfusion, hypercoagulability, following a traumatic injury such as a motor vehicle collision, or postsurgical complications following liver transplantation or cholecystectomy with hepatic arterial thrombosis or stenosis. Infarcts are usually peripheral wedge-shaped areas that are hypointense on T1WI and hyperintense on T2WI. A geographic distribution can be seen with larger infarcts. In liver transplant patients, findings of infarct can also be seen as irregularly shaped, tubular lesions along the distribution of the bile ducts from hepatic artery insufficiency. Typically the diagnosis of liver infarction is evident by the clinical scenario, and should not be confused with focal steatosis, abscess, or tumor.
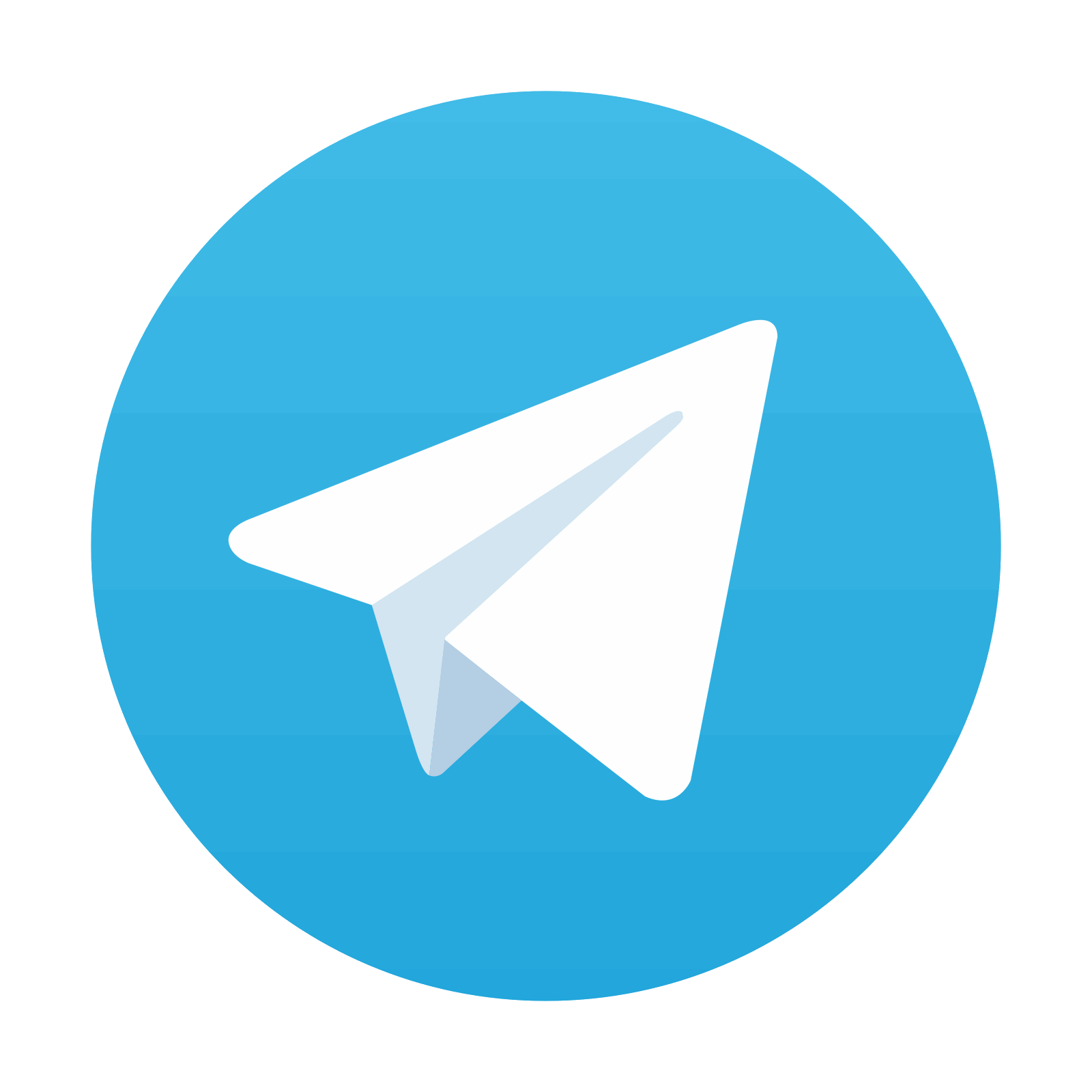
Stay updated, free articles. Join our Telegram channel

Full access? Get Clinical Tree
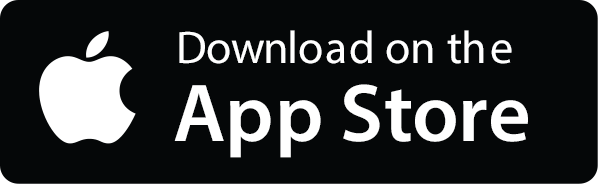
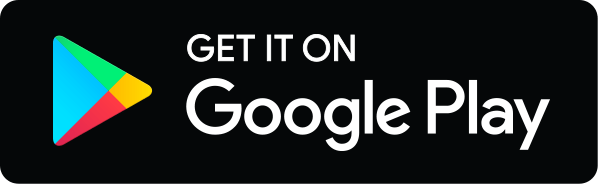