Etiology
Pulmonary edema is defined as an abnormal accumulation of fluid in the extravascular compartments (interstitial and airspace) of the lung. Traditionally, pulmonary edema has been divided into hydrostatic edema and permeability edema based on the presumed mechanism. Hydrostatic edema is caused by an elevation in pulmonary capillary pressure, and permeability edema is caused by disruption of the capillary endothelium, resulting in protein leakage into the surrounding tissue. This distinction into two categories is problematic. First, severe elevation in pulmonary capillary pressure in left ventricular failure may result in endothelial cell damage, resulting in a mixture of hydrostatic and permeability edema. Second, permeability edema is now known to occur in conditions that do not cause diffuse alveolar damage, such as hantavirus pulmonary syndrome and interleukin therapy. Permeability edema in these settings behaves very differently from permeability edema in patients with diffuse alveolar damage (acute respiratory distress syndrome [ARDS]). For these reasons, a modern definition of pulmonary edema should incorporate four main categories based on pathophysiology: hydrostatic edema, permeability edema with diffuse alveolar damage (DAD), permeability edema without DAD, and mixed edema caused by both hydrostatic and permeability edema.
The most common cause of hydrostatic pulmonary edema is a rise in pulmonary venous pressure secondary to disease of the left side of the heart. Increased pressure within the left atrium is transmitted to the pulmonary veins as a result of back pressure, most often from a failing left ventricle or obstruction to left atrial outflow. Rarely, venous hypertension is caused by stenosis of the pulmonary veins themselves, such as occurs in congenital or acquired venoocclusive disease or fibrosing mediastinitis. Other common causes of hydrostatic pulmonary edema are renal disease, hypervolemia, and liver failure. Both acute and chronic renal disease, with or without uremia, can be associated with acute pulmonary edema. A major contributing cause in these cases is left ventricular failure; however, it is likely that decreased protein osmotic pressure, hypervolemia, and increased capillary permeability also have roles. Administration of large volumes of intravenous fluids has been shown to cause pulmonary edema in patients who do not have underlying heart disease. Fluid overload is an important cause of hydrostatic edema in the postoperative period, in the elderly, and in patients with borderline cardiac or renal failure. Pulmonary edema occurs with increased frequency in patients who have cirrhosis or acute hepatic failure or have undergone liver transplantation. It is likely that the edema in these patients is due to a combination of increased capillary pressure, increased endothelial permeability, and decreased plasma osmotic pressure. Finally, hydrostatic pulmonary edema is also a well-described but uncommon complication of head trauma, seizures, and raised intracranial pressure.
Common Causes of Hydrostatic Pulmonary Edema
- •
Cardiogenic
- •
Left ventricular failure
- •
Mitral valve disease
- •
Left atrial myxoma
- •
- •
Obstruction of the pulmonary veins
- •
Primary (idiopathic) venoocclusive disease
- •
Fibrosing mediastinitis
- •
- •
Neurogenic
- •
Head trauma
- •
Seizures
- •
Increased intracranial pressure
- •
- •
Decreased capillary osmotic pressure
- •
Renal disease
- •
Fluid overload
- •
Cirrhosis
- •
Clinical Presentation
The main clinical manifestations of acute hydrostatic pulmonary edema are dyspnea, tachypnea, and orthopnea. Clinical findings include peripheral and central cyanosis, tachycardia, pallor, peripheral edema, and elevated jugular venous pressure. In the most severe cases the patient may expectorate frothy, blood-tinged fluid.
In patients in whom pulmonary edema develops insidiously, the symptoms may be mild, and dyspnea may occur only during exertion. Other suggestive symptoms in these patients include a history of orthopnea and paroxysmal nocturnal dyspnea. Some patients with chronic left-sided heart failure may have minimal symptoms even in the presence of extensive pulmonary edema evident on the chest radiograph. Laboratory testing, including B-type natriuretic peptide (BNP) and N-terminal fragment (NT-proBNP), are elevated in situations causing increased myocardial stress or in patients with renal failure. A normal laboratory value can be used to essentially rule out heart failure in patients presenting to the emergency department with dyspnea.
Pathophysiology
Under normal steady-state conditions, there is continual flow of fluid and protein from the pulmonary microvasculature to the interstitium; these substances are then returned to the bloodstream by the lymphatics. The volume of water and protein movement is dependent on the balance of pressure across the pulmonary microvasculature and on the permeability of the microvascular membrane. The factors that govern the formation and removal of extravascular water within the lungs are described by the fluid transport equation originally proposed by Starling.
An increase in microvascular hydrostatic pressure or a decrease in protein osmotic pressure in the microvascular lumen will result in the transudation of fluid from the microvessels into interstitial tissue (hydrostatic pulmonary edema). Sufficient accumulation of fluid in this compartment constitutes interstitial edema; when the storage capacity of the interstitial space is exceeded and epithelial cell damage occurs, edema floods into the alveolar airspaces ( Fig. 54.1 ). The pulmonary interstitium itself can be divided into two compartments: an alveolar septal (parenchymal) compartment and a peribronchovascular and interlobular septal (axial) compartment. Although the alveolar septal compartment constitutes a large percentage of the total interstitial space, its relatively low compliance means that fluid tends to accumulate to a much lesser extent within it than in the peribronchovascular and interlobular septal connective tissue.
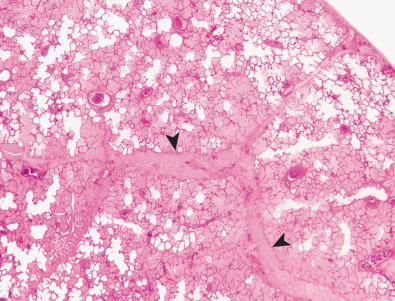
The pathogenesis of acute pulmonary edema secondary to head trauma, seizures, and raised intracranial pressure is poorly understood but likely is secondary to both hydrostatic and permeability mechanisms. Most studies suggest that the main mechanism is increased microvascular pressure (hydrostatic pulmonary edema). Experimental studies have shown transient, massive sympathetic discharge from the central nervous system, which results in generalized vasoconstriction, shift of blood volume into the pulmonary vascular compartment, and elevation of pulmonary microvascular pressure. It has also been shown that the sympathetic activity increases pulmonary vasomotor tone, causing constriction of pulmonary veins and a subsequent increase in pulmonary capillary hydrostatic pressure. There is also evidence that a direct negative inotropic effect on the heart contributes to the pathogenesis of the edema. Finally, elevated hydrostatic pressure may cause endothelial injury resulting in secondary permeability edema manifesting with high protein–content edema fluid.
Manifestations of the Disease
Radiography
Hydrostatic edema results in two principal radiologic patterns related to whether the fluid remains localized in the interstitial space or whether it also occupies the airspaces.
Predominantly Interstitial Edema
Transudation of fluid into the interstitial spaces of the lung inevitably constitutes the first stage of pulmonary edema because the capillaries are situated in this compartment. Although this transudation of fluid constitutes the first stage of fluid accumulation in the lungs, classic teaching and radiology dogma state that the initial radiographic sign in interstitial pulmonary edema is pulmonary venous hypertension. This sign manifests with redistribution of blood flow from the lower to the upper lung zones ( Fig. 54.2 ), with the upper lobe arteries becoming larger than the accompanying bronchus ( Fig. 54.3 ). This redistribution is termed by many authors as cephalization of the vasculature. Unfortunately, this sign is often not present in interstitial pulmonary edema or is difficult to accurately determine on radiography. Furthermore, redistribution of blood flow can be assessed reliably only on radiographs performed at maximal inspiration in the erect position. In a study of 86 patients with acute myocardial infarction, pulmonary vascular redistribution (cephalization) was present in only 1 patient with an elevated wedge pressure. Pistolesi and colleagues determined that redistribution of pulmonary blood flow (cephalization) does not correlate with wedge pressure but likely reflects chronic structural vascular changes and occurs in patients with chronically elevated venous pressures, such as those with chronic and recurrent heart failure or in patients with long-standing mitral stenosis (see Fig. 54.2 ).
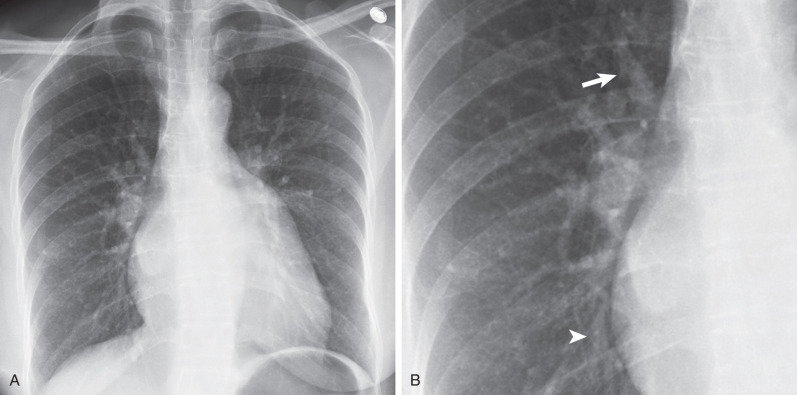
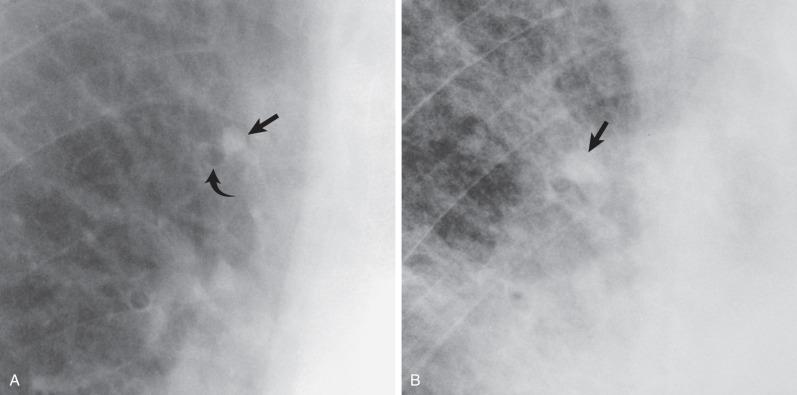
Therefore the first reliable radiographic evidence of interstitial pulmonary edema is fluid accumulating within the perivascular interstitial tissue and interlobular septa. As a result of this localization, edema fluid produces a characteristic radiographic pattern of loss of definition of segmental and subsegmental pulmonary vessels (i.e., vascular indistinctness) and thickening of the interlobular septa (Kerley A and B lines; Fig. 54.4 ). Unfortunately, vascular indistinctness relies on subjective assessment and can be caused by many factors other than interstitial edema, including motion, differences in equipment, lung volume, and patient rotation. A more objective finding, and often the most reliable radiographic sign of early interstitial edema, is subpleural interstitial edema. This sign manifests with thickening of the interlobar fissures (thick fissures) caused by fluid accumulation in the interstitium beneath the visceral pleura (see Fig. 54.4 ). Thick fissures are usually visible earlier than Kerley B lines because there are two layers of visceral pleural that contribute to the thickening, and the fissures are larger and longer than interlobular septa. It is important to note that a thick fissure does not represent pleural effusion within the fissure but rather thickening of the subpleural interstitium.
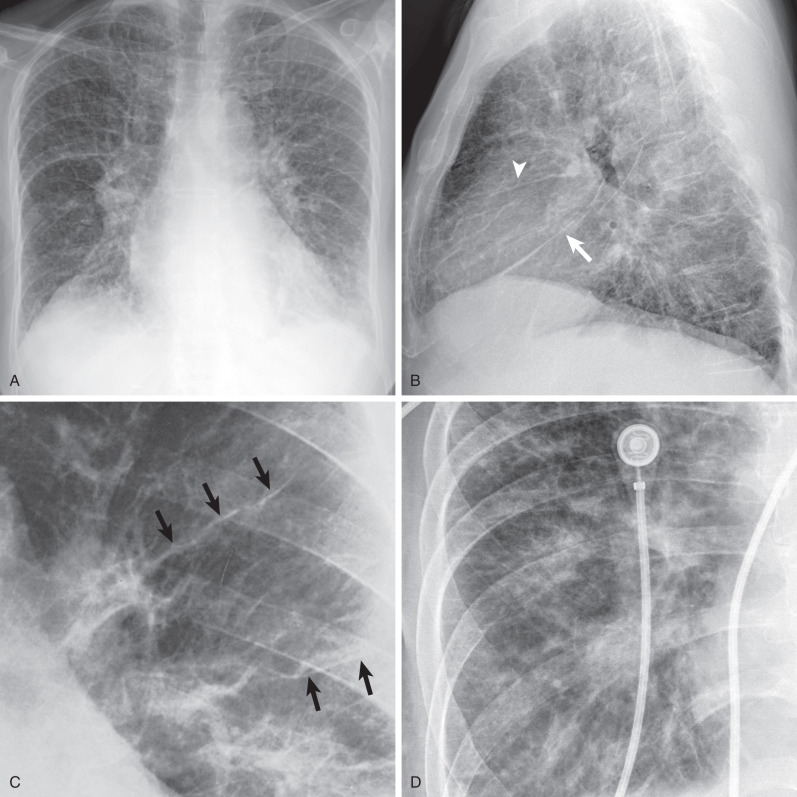
In circumstances in which edema fluid accumulates in the parenchymal interstitial tissue before the development of overt airspace edema, the accumulation is usually invisible or only faintly discernible radiographically as a “haze,” which tends to be predominantly lower zonal or perihilar in distribution. Although the severity of radiographic abnormalities correlates somewhat with pulmonary wedge pressure, there is often a phase lag between the elevation in wedge pressure and radiographic signs of pulmonary edema, possibly because of the time required for transudation of fluid into the extravascular space. The heart is generally enlarged; however, like pulmonary vascular redistribution, the heart size may be normal when the cause of the edema is recent myocardial infarction, coronary insufficiency, or restrictive cardiomyopathy.
Evidence for interstitial pulmonary edema is also provided by an increase in the thickness of the walls of bronchi seen end-on in the perihilar zones. In the absence of chronic airway disease, such as bronchitis or asthma, these structures measure less than 1 mm in thickness. When fluid accumulates in the interstitial tissue surrounding them, their shadow thickens and loses its sharp definition ( Fig. 54.5 ). After adequate treatment of edema, all these radiologic signs may disappear within a matter of hours to as long as a few days.
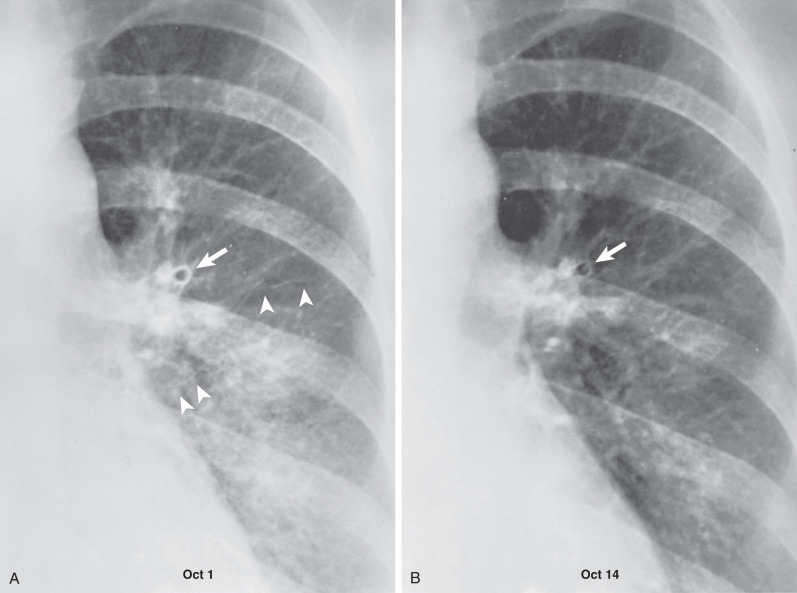
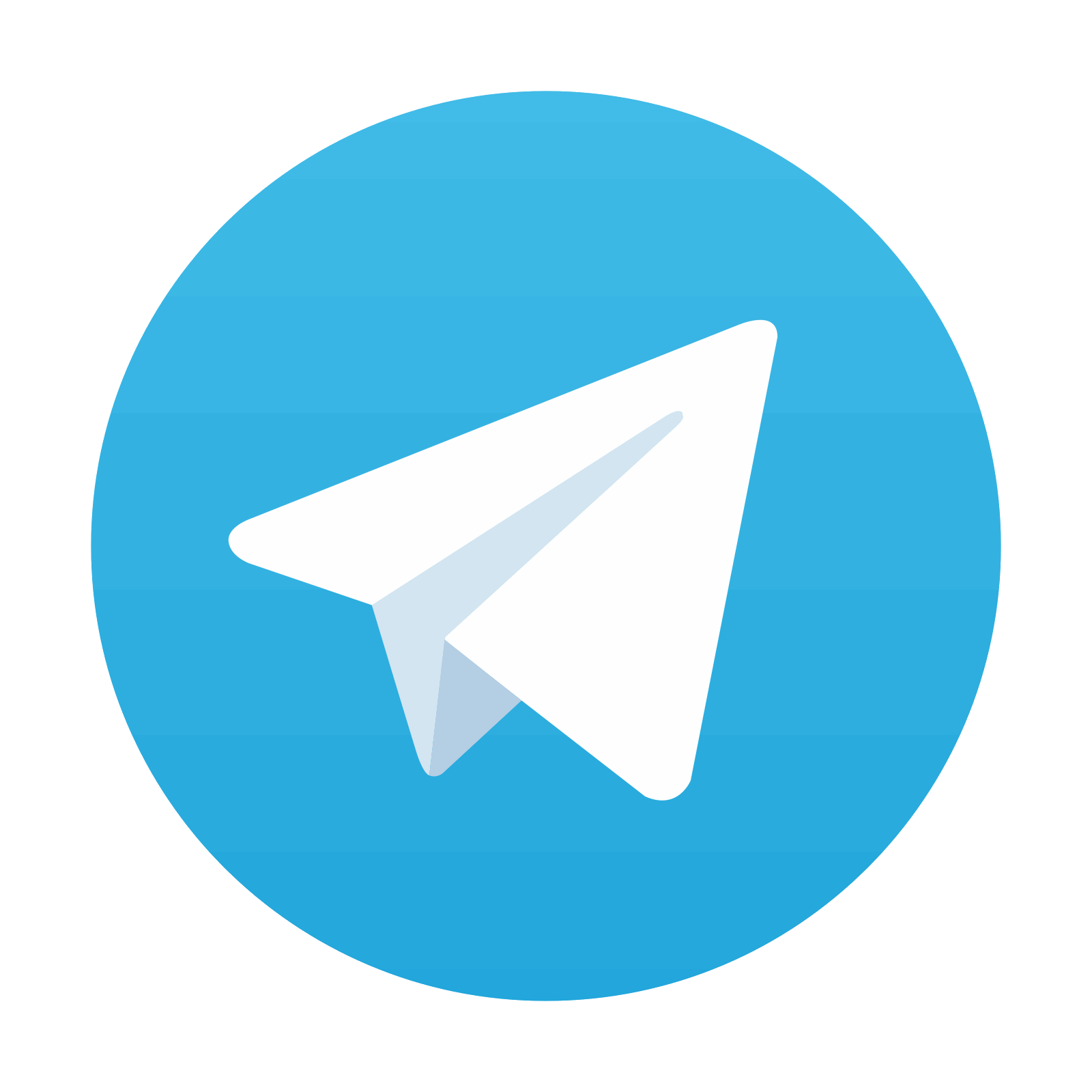
Stay updated, free articles. Join our Telegram channel

Full access? Get Clinical Tree
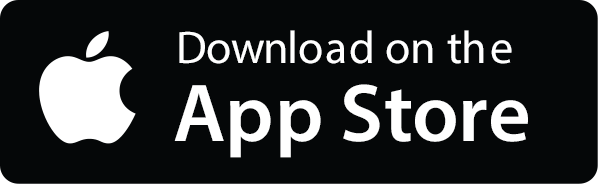
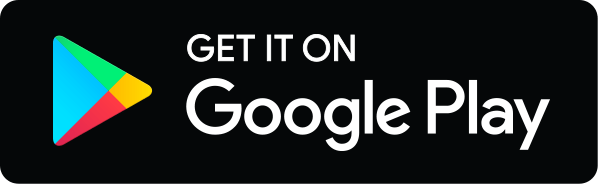
