Multidetector Computed Tomography
Multidetector computed tomography (MDCT) has become a fundamental technique of pancreatic imaging. Today, higher image quality can be obtained in abdominal imaging, and this is even more significant in pancreatic imaging, in which the reduction of acquisition time, the possibility of multiple phases of enhancement imaging, and higher resolution images in all three spatial planes, with the possibility of excellent multiplanar image reconstructions, permit MDCT to provide excellent images of the anatomic layout of the pancreas and its vasculature. This technique is now used to diagnose a variety of congenital, neoplastic, inflammatory, and traumatic pancreatic disease processes.
Pre-Examination Considerations
MDCT imaging of the pancreas is largely based on the study of the enhancement pattern of the lesions and the anatomic variants of the normal pancreas.
The first step of an MDCT study of the pancreas should be the administration of negative oral contrast medium to the patient, to distend the stomach and duodenum. Negative oral contrast medium should be preferred because it permits an easier evaluation of gastric and duodenal wall lesions and does not mask radiopaque stones in the common bile duct or pancreatic calcifications.
Contrast-enhanced imaging of the pancreas is based on different contrast medium parameters, such as iodine concentration, volume administration, and injection rate.
The use of intravenous iodinated contrast media is routine with MDCT, and the dose and rate of contrast injection must be adapted to the higher scanning speeds of MDCT.
The maximum amount of iodine should not exceed 35 to 45 g, independently from the concentration of the contrast medium used.
Achieving high concentrations of contrast enhancement to study the pancreas is possible either with increasing the increment of the injection rate or the iodine concentration of the contrast medium used (total iodine dose being kept constant); the latter is more important because it does not depend on the intravenous access and vessel diameter as the first is.
The contrast enhancement of the extracellular/extravascular space depends on the concentration gradient between intravascular and extracellular/extravascular spaces, the volume of the extracellular/extravascular space, the permeability of organ microvasculature and cellular interfaces, and surface area and time. A high concentration gradient between intravascular and extracellular/extravascular spaces allows a high influx of contrast material into the extracellular/extravascular space and contributes to high organ enhancement.
Keeping the rate of contrast injection and the total dose of iodine constant, the use of contrast medium with higher concentration improves significantly the arterial and portal venous phase enhancement compared with contrast medium with lower concentration.
As a result, the use of high concentrations of contrast medium improve conspicuity of hypovascular and hypervascular lesions in the pancreas.
Scanning Technique
A plain computed tomography (CT) scan of the upper abdomen is performed using 10-mm slice collimation to cover the pancreas ( Figure 45-1 ). Depending on the scanner type, a pancreatic phase is performed using 1- to 2-mm slice collimation. Acquisition of the pancreatic phase is usually at a delay of 35 to 40 seconds after a bolus of 125 to 150 mL of iodinated contrast medium injected at a rate of 4 to 5 mL/s. The scanned area extends from the diaphragm to below the transverse duodenum in a single breath-hold. A weight-based approach to intravenous contrast medium administration is now considered more appropriate to optimize the iodine dose for a study. An iodine dose of 550 mg/kg can be used for both pancreatic and vascular enhancement, which translates into 1.8 to 2 mL/kg.
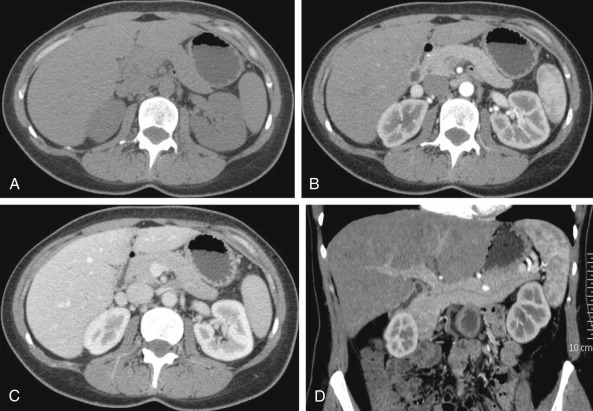
For the next phase, the patient is instructed to breathe deeply after the pancreatic phase acquisition, and a second spiral acquisition is performed at a 70- to 80-second scan delay (see Figure 45-1 ). This is the portal venous phase, which covers the entire upper abdomen using 2.5- to 5-mm slice collimation, depending on the patient’s body habitus. This phase is critical for the detection of small hypodense liver metastases and the diagnosis of venous encasement by a tumor. Early arterial phase scans can be performed if a CT angiogram is desired.
Dynamic Imaging
Contrast-enhanced imaging of the pancreas is usually performed in three phases. The first phase is the early arterial phase, which is obtained approximately 20 seconds after contrast administration (see Figure 45-1 ). In this phase, contrast medium is preferentially concentrated within the arterial tree with almost no enhancement of the pancreatic parenchyma. The delayed arterial phase, also called the pancreatic phase, is acquired nearly 35 to 40 seconds after contrast agent administration. In this phase, the pancreatic parenchyma enhances optimally. On the third phase, the portal venous phase, usually acquired at 65 to 70 seconds after contrast agent administration, we have the highest contrast uptake by the portal venous vessels, as well as the liver parenchyma (see Figure 45-1 ).
The bolus tracking techniques, performed by placing a region of interest marker in the aorta just above the level of the pancreas and starting to acquire images when arterial enhancement peaks reach a predetermined Hounsfield unit (HU) trigger value (usually 120 to 130 HU), helps determine the exact timing of scan delay for a precise individual study for each patient.
Scanning for the pancreatic phase starts 15 seconds after threshold is reached.
Image Postprocessing
A large variety of image processing options are available, such as curved reformatted images, minimum intensity projections, volume-rendered images, standard coronal and sagittal plane reformatted images, and coronal oblique reformatted images (see Figure 45-1 ). Among these, curved reformatted images give important information about vascular involvement and ductal abnormalities ; minimum intensity projections can be used to visualize low-attenuation structures, such as pancreatic and common bile ducts, whereas maximum intensity projections can evaluate high-attenuation structures, such as peripancreatic vasculature. Volume-rendered images are of great aid in visualization of the peripancreatic vessels and tumor encasement.
Such additional two-dimensional (2D) and 3D reformatted images provide fundamental information about tumor extent, peripancreatic vascular involvement, or ductal abnormalities, which may be difficult to evaluate on axial images and are also useful to display the lesion characteristics to surgeons and gastroenterologists.
Magnetic Resonance Imaging
Recent technologic innovations to both hardware and software have made magnetic resonance imaging (MRI) a reliable technique for studying the pancreas. MRI is potentially the only diagnostic tool that makes it possible to simultaneously image the pancreatic parenchyma and focal (neoplasms, autoimmune pancreatitis) or diffuse (acute or chronic pancreatitis) pathologic processes, reveal dilatation of pancreatic and bile ducts, and identify gallstones, peripancreatic or hemorrhagic fluid accumulations, lymphadenopathies, hepatic metastases, and vascular anomalies.
In many centers, MRI is currently considered the third method for studying the pancreas, after ultrasonography and CT, not so much because of the technical problems but because of the high costs and, above all, the limited “machine time” useable by departments dealing with pancreatic disease.
Technical Aspects
To achieve the best possible abdominal evaluation with MRI, it is important to use a high-intensity magnetic field (>1.0 tesla) that guarantees high signal-to-noise ratio (SNR), phased-array surface coils, automatic injectors, powerful gradients, and rapid sequences that can provide images without artifacts resulting from movement or breathing.
The phased-array surface coils integrate the signal arriving from several coils, reconstructing the final image with a high SNR that ensures greater spatial resolution for the images acquired. The recent development of high-power gradients has made it possible to obtain rapid sequences that ensure better images of the abdomen during breath-holding, thus eliminating the artifacts resulting from breathing that decrease image quality.
The fat saturation obtained with high field units improves the latitude for nonadipose tissues and reduces the artifacts along the phase-encoding direction resulting from movement of subcutaneous adipose tissues during scanning.
The improvement in gradient performance and the development of new pulse sequences (e.g., the T1-weighted volumetric sequences with fat suppression or the half-Fourier rapid acquisition with relaxation enhancement [RARE] sequences) augment the possibility of obtaining better anatomic coverage with more homogeneous saturation of the signal emitted by the adipose tissue.
The development of rapid sequences, associated with fat saturation, enables dynamic imaging of the upper abdomen during the administration of gadolinium chelates to study pancreatic parenchyma vascularization, to identify and characterize focal lesions, to evaluate any infiltration or involvement of the peripancreatic vessels, and to identify and characterize focal lesions in the liver.
Protocol
The present protocol calls for use of the following techniques: T1-weighted, gradient recalled echo (GRE) sequence axial imaging with “in-phase” and “out-of-phase” echo time (TE); T2-weighted RARE axial imaging; T2-weighted half-Fourier RARE axial and coronal thin slice (5 to 6 mm) imaging; axial and coronal MR cholangiopancreatography (MRCP); and T1-weighted GRE precontrast axial imaging with fat suppression on the axial plane, possibly with 3D technique, before and during intravenous administration of gadolinium chelates.
MRCP, an MRI technique that permits noninvasive study of the bile and pancreatic ducts without the use of a contrast medium, has replaced direct cholangiography in most diagnostic indications. The underlying principle behind MRCP is the use of T2-weighted sequences with very long TE (>800 ms) so that when the echo is detected, only the present stationary fluids (bile and pancreatic juices) are able to provide a signal; the parenchymatous organs, on the other hand, are completely relaxed and thus do not emit any signal. This results in good contrast resolution between the bile and pancreatic ducts and the parenchymatous organs. During intravenous administration of secretin, dynamic MRI improves visualization of the principal pancreatic and secondary ducts, thus permitting functional imaging of the pancreas.
Imaging Appearance
T1-Weighted Images
The pancreas is intrinsically hyperintense on T1-weighted images, with values higher than are seen in other hypochondriac organs ( Figure 45-2 ). The reason for this is not fully known. The hypotheses advanced are the high quantity of pancreatic juice proteins within the pancreas glands; the presence of large quantities of endoplasmic reticula in the acinar cells; or the presence of paramagnetic ions such as manganese. In the elderly, the high signal intensity of the pancreas can be lower than that of the liver, most likely as a result of age-related fibrosis.
T1-weighted GRE images can be used with a fat suppression pulse ( Figure 45-3 ). Eliminating the signal emitted by the adipose tissues, which has a short T1 relaxation time, redistributes the gray scale over a narrower range, thus making the sequence more sensitive to minor variations in signal intensity.
The T1-weighted GRE images are used for dynamic imaging of the pancreas after intravenous administration of gadolinium chelates ( Figure 45-4 ). The dynamic imaging is performed by acquiring 25- to 30-second images (arterial-capillary phase), 45-second images (pancreatic phase), and 80- to 90-second images (portal vein phase) (see Figure 45-4 ). T1-weighted fat-suppressed coronal images are obtained 5 to 10 minutes after gadolinium administration.
Because of its glandular nature, the pancreas is a highly vascularized organ. For this reason it produces 76% to 115% higher signal intensity during the first minute after gadolinium administration. On the delayed images, the pancreas shows signal intensity similar to that of the liver.
The T1-weighted GRE images with fat saturation and the arterial phase after contrast administration are the most sensitive sequences for identifying focal pancreatic lesions. The portal vein phase is the most useful in evaluating portal vein pathologic processes and identifying lymphadenopathies. The delayed images, obtained 10 minutes after administration of the contrast medium, are instead more useful in identifying cholangiocarcinoma, ascending cholangitis, peritoneal abscesses, and metastases.
The T1-weighted images in GRE sequences, obtained with “in-phase” and “out-of-phase” echo times, are useful in identifying hepatic steatosis, lymphadenopathy, infiltration of the peripancreatic adipose tissue in acute pancreatitis, accumulation of fluids with high methemoglobin or protein concentrations, and adrenal adenoma.
T2-Weighted Images
In T2-weighted images, the parenchymal signal is generally hypointense versus the hepatic parenchyma ( Figure 45-5 ). Under physiologic conditions, the T2-weighted images provide excellent visualization of the bile and pancreatic duct anatomy and pathologic processes as well as of any peripancreatic fluid accumulations, neuroendocrine tumors, and hepatic metastases.
Other uses of T2-weighted images include the identification of signal hypointensity of fibrosis and iron deposited during hemochromatosis, responsible for the marked hypointensity of the pancreatic gland signal.
Magnetic Resonance Cholangiopancreatography Images
MRCP is an imaging technique that is able to noninvasively assess bile and pancreatic ducts, in a multiplanar fashion, without injection of contrast material ( Figure 45-6 ). MRCP takes advantage of the inherent contrast properties of stationary fluid in the biliary and pancreatic ducts.
The interest in MRCP in the past 5 years is due to its accuracy, safety, and availability with nearly all modern scanners, so that MRCP has replaced diagnostic endoscopic retrograde cholangiopancreatography in most indications.
The basic principle underlying MRCP is that body fluids such as bile and pancreatic secretions have high signal intensity on T2-weighted MR images whereas background signal generates no or little signal. The images of the pancreaticobiliary tree produced by MRCP are similar in appearance to those obtained by direct cholangiographies (i.e., endoscopic retrograde cholangiopancreatography [ERCP] or percutaneous transhepatic cholangiography [PTC]).
MRCP has been initially applied in investigating the biliary ducts; the first reports have focused on the accuracy of MRCP in detection of biliary duct dilatation and choledocholithiasis. The application of MRCP to the assessment of the pancreatic duct is less common because pancreatic disease is less frequent compared with biliary disease and the size of the main pancreatic duct is smaller compared with the common bile duct, resulting in SNRs that are less advantageous. Therefore, the visualization of the pancreatic ducts is more challenging compared with that of the biliary ducts.
Despite these challenges, with the use of a surface coil, MRCP clearly showed the main pancreatic duct in the head, body, and tail of the gland, respectively, in 79%, 64%, and 53% of cases. Furthermore, in patients with chronic pancreatitis, MRCP showed moderate to high agreement with ERCP in assessing ductal abnormalities. In this group of patients, a high number of false-negative results have been reported because of the small size of the main pancreatic duct, especially in the tail of the pancreas and of the side branches.
MRCP images are able to demonstrate biliary and pancreatic duct lithiasis, stenoses, pancreatic cysts, and accumulations of peripancreatic fluids. As opposed to direct cholangiography, the T2-weighted MRCP images also can be used to study the ducts above a tight stenosis.
The exogenous administration of secretin also improves visualization of pancreatic ducts on MRCP (S-MRCP). To obtain better results, MRCP images should employ a slice thickness that encompasses the pancreatic ducts, their emergence in the duodenum, and the common bile ducts. A negative contrast agent consisting of 200 mL of superparamagnetic iron oxide particles can be administered to eliminate overlapping fluid-containing organs ( Figure 45-7 ).
Therefore, secretin can be employed as a contrast agent because it improves visualization of the pancreatic ducts ( Figure 45-8 ). However, at the same time, because it physiologically stimulates the exocrine pancreas, when its use is combined with high temporal resolution imaging, functional studies can be performed assessing the dynamics of pancreatic secretion and the pancreatic exocrine reserve. Thus, S-MRCP enables morphologic and functional evaluation of the pancreas.
Evaluation of the Response to Secretin.
Secretin improves visualization of the main pancreatic duct (see Figure 45-8 ), particularly in patients with normal or minimally dilated pancreatic ducts. In this group of patients, there was a significant improvement in main pancreatic duct visualization: 65% (164 of 252) of the segments before and 97% (245 of 252) after secretin. A nonsignificant improvement in main pancreatic duct visualization was also observed in patients with severe chronic pancreatitis, from 91% (85 of 93 segments) to 100% (93 of 93 segments). This was probably related to the enlargement of the main pancreatic duct, which represents the most frequent sign of severe chronic pancreatitis.
The hallmark of ERCP diagnosis of early chronic pancreatitis is represented by the dilatation of side branches. Because of the small size of these branches, MRCP is not able to routinely recognize dilated side branches, therefore leading to a high false-negative rate. Secretin administration improves the visualization of the side branches (from 4% to 63% of the patients), thus aiding in diagnosing chronic pancreatitis in its early stage ( Figure 45-9 ). This improved visualization of dilated side branches makes S-MRCP a promising alternative to diagnostic ERCP, with the endoscopic approach indicated only for therapeutic purposes.
Secretin administration also improves visualization of endoluminal filling defects. This improvement, however, is not significant in patients with severe chronic pancreatitis, because the main pancreatic duct is enlarged, containing a larger amount of fluid that encompasses the low-intensity protein plugs along most or all of their circumference. This improvement is therefore particularly important in mild or moderate chronic pancreatitis with a minimally dilated main pancreatic duct where there might be insufficient pancreatic secretion to delineate the whole circumference of the protein plugs. The detection of endoluminal filling defects is important in planning adequate treatment, such as interventional ERCP and/or lithotripsy.
Pancreas divisum, a congenital abnormality that results from the failure of fusion of the dorsal and ventral ducts during organogenesis, has a prevalence of 12% at MRCP. There are other additional anatomic variants that share the feature of egression of the major fraction of pancreatic secretions via the dorsal duct orifice. These variants, characterized by a dominant dorsal duct, are found in nearly 10% of the population, double the prevalence of pancreas divisum. Dynamic MRCP after secretin stimulation improved detection of pancreas divisum in 23% of the patients.
Secretin also improves the accuracy of MRCP in assessing ductal abnormalities. When comparing MRCP and S-MRCP findings with those from ERCP in patients with recurrent episodes of acute pancreatitis, MRCP showed an overall sensitivity, specificity, and diagnostic accuracy of 53%, 100%, and 93%, respectively, whereas S-MRCP showed an overall sensitivity, specificity, and diagnostic accuracy of 94%, 97%, and 97%, respectively. The slight reduction in specificity of S-MRCP compared with MRCP is due to three false-positive findings.
Functional Evaluation.
The dynamic assessment obtainable with rapid imaging after secretin administration gives information on the main pancreatic duct flow dynamics and on the hydrodynamic changes induced by the increased fluid secretion and subsequent elimination into the duodenum ( Figures 45-10 to 45-12 ).
Papillary strictures can be detected by means of S-MRCP. Papillary stenosis is responsible for persistent dilation of the main pancreatic duct on delayed MRCP images after secretin administration. Ten minutes after secretin administration, the mean maximal diameter of the main pancreatic duct in patients with papillary stenosis was significantly larger than that in the control subjects without papillary stenosis; furthermore, there was no overlap between observed individual values for the patients with papillary stenosis and for the control subjects ( Figure 45-13 ).
Another treatable cause of impeded pancreatic secretion outflow is santorinicele, which occurs in patients with pancreas divisum. Santorinicele is a cystic dilatation of the distal dorsal duct, just proximal to the minor papilla. It is termed santorinicele in analogy with ureteroceles and choledochoceles, and it is believed to result from a combination of relative obstruction and weakness of the distal duct wall, either acquired or congenital. Santorinicele has been suggested as a possible cause of relative stenosis of the accessory papilla, which in association with unfused dorsal and ventral ducts results in high intraductal pressure. The increased intraductal pressure may be responsible for individual attacks of recurrent acute pancreatitis, which may be caused by temporary obstruction of the minor papilla during passage of protein aggregates. The hypothesis of reduced pancreatic outflow is further sustained by the remarkable reduction in size of the main pancreatic duct and of the santorinicele in patients who underwent sphincterotomy of the minor papilla on follow-up S-MRCP. Furthermore, patients who underwent endoscopic sphincterotomy also had clinical improvement with remission of symptoms.
Papillary stenosis, either idiopathic or the result of santorinicele, is responsible for a delay in duodenal filling after the administration of secretin. This duodenal filling after secretin stimulation can be used to semi-quantitatively evaluate the pancreatic secretion, which represents an indirect index of the pancreatic exocrine reserve (see Figure 45-10 ). The evaluation of the pancreatic exocrine reserve may be important because it can be used to help establish the clinical diagnosis and monitor the disease and its treatment. Currently, the most valuable pancreatic function tests are the duodenal and intraductal secretin tests with sampling of the duodenal juice or pure pancreatic juice. With invasive techniques, such as those just mentioned, the evaluation of the pancreatic exocrine function is performed by measuring bicarbonate output and concentration in the pure pancreatic juice collected after secretin stimulation.
Duodenal filling assessed by dynamic MRCP after secretin administration is a noninvasive means to assess pancreatic exocrine reserve. Patients with severe chronic pancreatitis showed a duodenal filling that was significantly inferior to that of patients with suspected chronic pancreatitis ( P < .001), probably reflecting a reduced pancreatic exocrine reserve in the advanced phase of the disease.
Therefore, the combination of MRCP and secretin administration enhances pancreatic duct visualization and reduces the high false-negative ratio of MRCP. The improved side-branch visualization in S-MRCP can allow prompt diagnosis of early chronic pancreatitis, making S-MRCP a valid, noninvasive alternative to diagnostic ERCP in this group of patients. Furthermore, the dynamic assessment of the pancreatic duct enables detection of causes of impeded pancreatic secretion outflow, such as papillary stenosis or santorinicele.
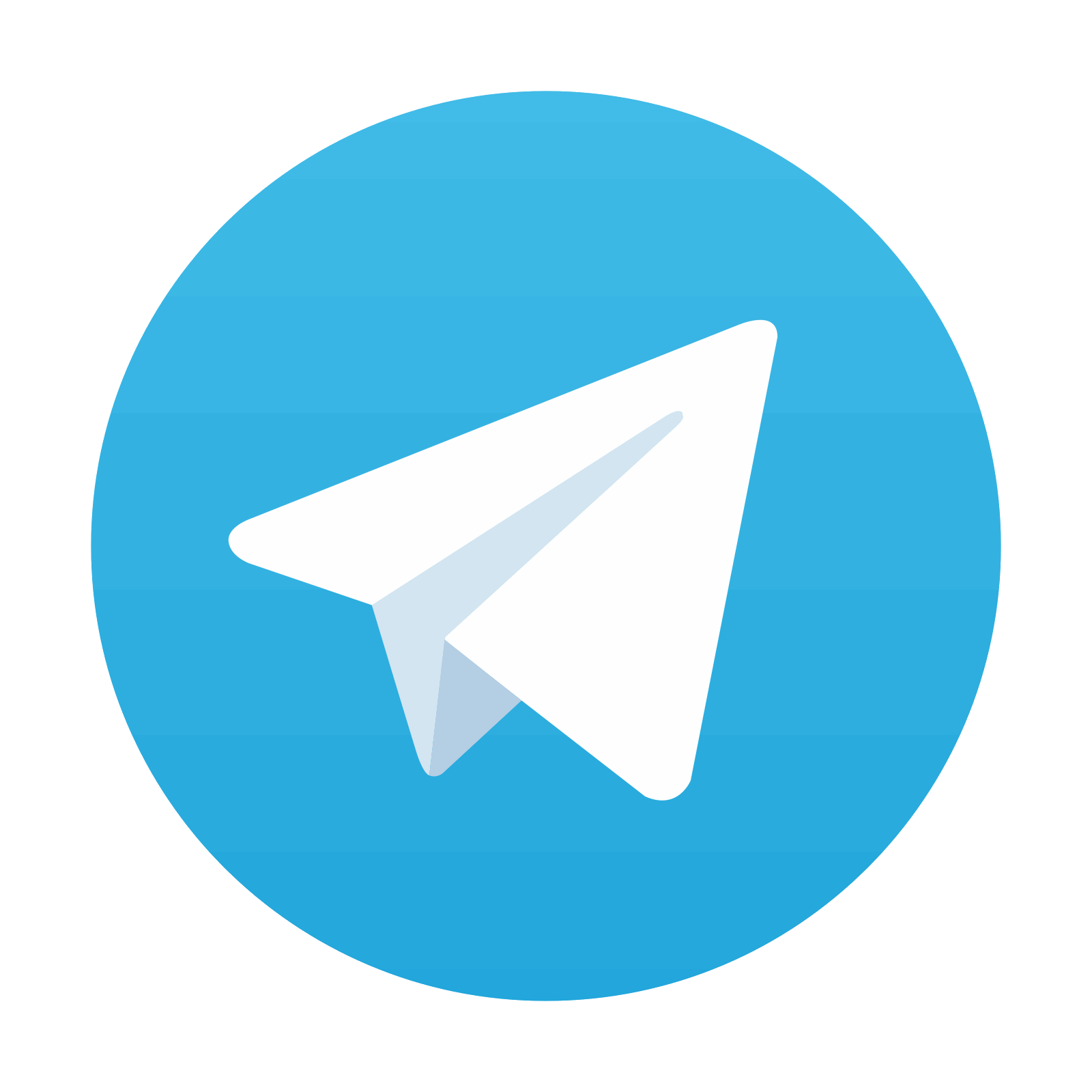
Stay updated, free articles. Join our Telegram channel

Full access? Get Clinical Tree
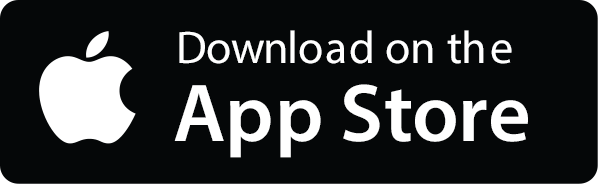
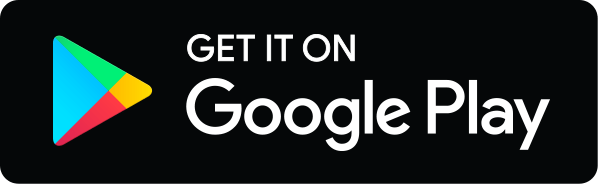