Magnetic resonance (MR) imaging at 3 T has proved superior to 1.5 T in the brain for detecting numerous pathologic entities including hemosiderin, tiny metastases, subtle demyelinating plaques, active demyelinating plaques, and some epileptogenic foci, as well as small aneurysms with MR angiography. 3 T is superior to most advanced imaging techniques including diffusion, diffusion tensor imaging, perfusion, spectroscopy and functional MR imaging. The increased signal/noise ratio at 3 T permits higher spatial resolution. Initially spine imaging at 3 T proved more difficult with less successful results. During the past 7 years, technological advances in magnet and surface coil design as well as improved radio frequency transmitters and pulse sequence design in combination with the large body of knowledge accrued by radiologists and physicists during a nine year experience with clinical imaging of the spine with the doubled B0, has resulted in 3 T MRI of the spine achieving a reputation similar to that for brain imaging.
- •
3 T imaging of the spine shows improvements compared with 1.5 T.
- •
Increased signal/noise ratio (SNR) allows for thinner slices and increased in-plane resolution and therefore better spatial resolution.
- •
There is increased conspicuity of paramagnetic contrast at 3 T.
- •
At 3 T, increased SNR also improves diffusion imaging, diffusion tensor imaging (DTI), spectroscopy, functional magnetic resonance (MRI) imaging, spinal magnetic resonance (MRA) angiography and arterial spin labeling (ASL).
- •
Increased susceptibility on T2 weighted (T2WIs) images and increased SNR produces improved blood level oxygen dependence (BOLD) effect at 3 T. The same combination with low dose gadolinium enhances perfusion imaging.
- •
Increased chemical shift effect at 3 T improves spectroscopy and fat saturation (FS).
- •
Parallel Imaging (PI) has a synchronous effect with 3 T which permits the use of higher PI factors. This shortens scan times thus ameliorating the increased SAR that is inherent when doubling the B0 from 1.5 T to 3 T.
- •
The goal is to improve the image quality and spatial resolution for each and every pulse sequence compared with 1.5 T.
- •
Use thin slices and increased in-plane resolution in evaluation of cervical and thoracic spines. With T2 sagittal spin-echo sequences, BLADE helps diminish patient motion artifacts when scanning patients; especially young children and patients with movement disorders.
- •
Use 2 mm sagittal and 2.5 mm slice thickness in the axial plane with T2 turbo spin echo (TSE) with or without FS, or use ultrathin sections with either T2 SPACE (sampling perfection with application optimized contrast by using different flip angle evolution) for subtle foraminal or lateral recess encroachment in patients with radiculopathy, and ultrathin three-dimensional (3D) or even two-dimensional (2D) imaging with the best sequence your manufacturer provides for differentiating cord gray and white matter.
- •
3D sequences mitigate flow (pulsation) artifacts that are exacerbated at 3 T.
- •
T2 SPACE should be obtained only when seeking very high spatial resolution with pulsation dampening. SAR friendly but lacks adequate intramedullary contrast resolution and shouldn’t be used tor detecting subtle abnormalities in cord signal.
- •
T1 fluid-attenuated inversion recovery (FLAIR) should be the only T1 sagittal sequence for any region in the spine. It allows for best differentiation of all interfaces between all of the following; bone, disc with spinal cord, conus and cauda equina and best delineates soft tissue and/or bone with CSF. FS is as robust with T1 FLAIR as with T1 spin echo sequences.
- •
For T1-weighted images in the axial plane for the thoracic or cervical regions, use T1 volumetric interpolated breath-hold examination (VIBE) or your manufacturer’s comparable sequence. Cord–cerebrospinal fluid (CSF) interfaces are obscured with T1 spin echo or conventional spin echo (CSE) in any plane. T1 VIBE and other 3D T1 gradient echo (GRE)-based techniques permit adequate detection of contrast enhancement while providing excellent spatial resolution and superb delineation of cord dimension as well as differentiation between cord-CSF and/or nerve root–CSF. The dampening of pulsation artifacts makes it even better for delineating these soft tissue–CSF interfaces. This author prefers VIBE FS for pre and post contrast axial T1 WIs.
- •
In the author’s experience, STIR and T1 FLAIR with phase contrast are the two best sagittal sequences for evaluating demyelinating disease at 3 T; a new 3-point Dixon technique (IDEAL on GE medical systems MRI systems) that should be available on all fourth-generation and upgraded third-generation 3 T systems. This sequence provides better FS in patients who have pronounced susceptibility artifact from metal after surgery. It also provides excellent FS at the cervicothoracic junction for both soft tissue neck and brachial plexus imaging at 3 T.
- •
There are multiple techniques (increasing bandwidth, turbo factor and matrices and decreasing, slice thickness and TE; orienting the frequency direction parallel to the long axis of metal may also help) mitigate susceptibility artifact thus improving image quality at any field strength because susceptibility scales with field strength.
- •
Because susceptibility artifacts occur in the frequency direction, orienting frequency encoding parallel to the long axis of metal will help diminish these artifacts in patients with spine hardware.
- •
There is a new sequence available on latest generation scanners that decreases both the in-plane and out-of-plane distortion that occurs with metal. It is designated slice encoding for metal artifact compensation (SEMAC).
- •
Try advanced imaging techniques with late-generation 3 T systems. The author routinely does diffusion and DTI on all patients with multiple sclerosis (MS) spectroscopy and perfusion for tumors. The author tries T2-weighted perfusion technique (CBV) and dynamic contrast enhancement (DCE) with permeability (Ktrans).
- •
The authors, facility has lost less than 10 patients in 3 years due to claustrophobia or body habitus related to the ultrashort, 70 cm bore size. The short bore length also decreases SAR as with single-voxel spectroscopy when differentiating between an infectious-inflammatory lesion involving the cord and a primary cord tumor. Tractography can help differentiate between a cord glioma and ependymoma because ependymomas usually arise from the ependymal surface of the central canal and should theoretically splay white matter tracts apart, whereas gliomas tend to infiltrate tracts.
- •
Similar to 3 T of the brain, when performing spinal cord perfusion, only half to one quarter the standard dose of gadolinium given at 1.5 T is necessary for all contrast enhanced spine imaging at 3 T.
- •
Despite the significantly increased cost associated with new-generation wide-bore and shorter-bore systems, the financial burden is compensated by the imager’s ability to scan obese or extremely large, muscular patients whose body habitus may result in MR imaging that is nondiagnostic or equivocal if imaged on an open MRI scanner or perhaps even a 1.5 T, system. Most claustrophobic and very large patients tolerate being scanned in a 70-cm diameter, short-bore 3 T system.
Nearly a decade has passed since 3 T was first made practical for spine MR imaging with the advent of a compatible 8-channel phased array spine coil. A limited supply of these coils prevented widespread use until late 2004. Most first-generation 3 T systems were not ready for routine clinical use. The only way that early 3 Ts could cope with the increased energy deposited in patients with the doubled magnetic field strength (B0) was by interrupting the scan to allow cooling.
Our first-generation 3 T was a long-bore (215 cm) system that was delivered in early 2003, followed by our first spine coil in May 2003. There were frequent scan interruptions and inadequate computer processing power to rapidly formulate the increased data generated by the thinner slices and increased matrices (in-plane resolution) that should be obtained using 3 T. As a result, patient scan times were excessively long and susceptible to motion artifact. Patient throughput was impossible and the quality of spine scans was not equal to those imaged on our 1.5 T.
One manufacturer’s first 3 T system precluded the user from adjusting receiver bandwidth, inversion times (TI), or turbo factor (TF; TF = ETL), resulting in poor susceptibility compensation and suboptimal contrast for certain pulse sequences including T1 FLAIR, which, in many experienced 3 T users’ opinions, is important for optimizing 3 T spine protocols ( Table 1 ).
CSPx Routine | TR | TE | TI | TA | Matrix | BLADE | IPAT | Turbo Factor | Gated | ST (mm) | BW Roffset (Hz/px) |
---|---|---|---|---|---|---|---|---|---|---|---|
Sagittal T2 TSE 22 cm | 3000 | 100 | n/a | 2:50 | 320 × 320 | Yes | Yes 2 | 21 | No | 2.0 | 260 |
Sagittal T1 FLAIR 22 cm | 2000 | 18 | 800 | 3:06 | 320 × 256 | No | Yes 2 | 6 | No | 3 | 217 |
Axial T2 TSE FS 16 cm | 5000 | 84 | n/a | 5:02 | 256 × 192 | No | Yes 2 | 16 | No | 2.5 | 279 |
Axial T2 MEDIC | 650 | 17 | n/a | 5:33 | 512 × 384 | No | Yes 2 | n/a | No | 2.5 | 399 |
7 elements | |||||||||||
Abbreviations: BW, bandwidth; n/a, not applicable. |
CSP Cord | TR | TE | TI | TA | Matrix | BLADE | IPAT | Turbo Factor | Gated | ST (mm) | BW (Hz/px) |
---|---|---|---|---|---|---|---|---|---|---|---|
Sagittal T2 STIR 22 cm | 4000 | 47 | 220 | 2:50 | 320 × 256 | No | No | 16 | No | 2.0 | 252 |
Sagittal T1 FLAIR 22 cm | 2000 | 18 | 800 | 3:06 | 320 × 256 | No | Yes 2 | 6 | No | 3 | 217 |
Axial T2 FS 16 cm | 5000 | 84 | n/a | 5:02 | 256 × 192 | No | Yes 2 | 16 | No | 2.5 | 279 |
Axial T1 VIBE 16 cm | 6.37 | 2.45 | n/a | 2:54 | 256 × 248 | no | Yes 2 | n/a | No | 3 | 360 |
Sagittal T1 FLAIR FS after 22 cm | 000 | 18 | 800 | 3:06 | 320 × 256 | No | Yes 2 | 6 | No | 3 | 217 |
Axial T1 VIBE after 16 cm | 6.37 | 2.45 | n/a | 2:54 | 256 × 248 | No | Yes 2 | n/a | No | 3 | 360 |
C and T Spine for Cord DW Imaging | TR | TE | EPI Factor | TA | Matrix | BLADE | IPAT | Number of Shots | Diffusion Weightings | ST (mm) | BW (Hz/px) |
---|---|---|---|---|---|---|---|---|---|---|---|
Sagittal multishot echoplanar DW imaging | 2000 | 60 | 64 | 2:44 | 128 × 128 | No | 2 | 5 | 0 400 600 | 3 | 1028 |
TSP Routine | TR | TE | TI | TA | Matrix | BLADE | IPAT | Turbo Factor | Gated | ST (mm) | BW (Hz/px) |
---|---|---|---|---|---|---|---|---|---|---|---|
Sagittal T2 TSE 32 cm | 2500 | 89 | n/a | 4:22 | 384 × 307 | No | Yes 2 | 16 | Yes | 2.0 | 266 |
Sagittal T1 FLAIR 32 cm | 2000 | 21 | 800 | 3:50 | 320 × 256 | No | Yes 2 | 8 | No | 3 | 217 |
Axial T2 FS 18 cm | 3500 | 88 | n/a | 3:18 | 256 × 192 | No | No | 12 | Yes | 3 | 337 |
11 elements | — | — | — | — | — | — | — | — | — | — | — |
TSP Cord | TR | TE | TI | TA | Matrix | BLADE | IPAT | Turbo Factor | Gated | ST (mm) | BW (Hz/px) |
---|---|---|---|---|---|---|---|---|---|---|---|
Sagittal T STIR 32 cm | 4000 | 57 | 220 | 4:42 | 384 × 288 | No | No | 17 | Yes | 2 | 250 |
Sagittal T1 FLAIR 32 cm | 2000 | 21 | 800 | 3:50 | 320 × 256 | No | Yes 2 | 8 | No | 3 | 217 |
Axial T2 FS ran upper and lower 18 cm | 2800 | 75 | n/a | 3:32 | 256 × 192 | No | No | 12 | Yes | 3 | 337 |
Axial T1 VIBE 18 cm | 5.38 | 2.45 | n/a | 2:49 | 256 × 248 | No | Yes 2 | n/a | No | 3 | 360 |
Sagittal T1 FLAIR FS after 32 cm | 2000 | 21 | 800 | 3:50 | 320 × 256 | No | Yes 2 | 8 | No | 3 | 217 |
Axial T1 VIBE after | 5.38 | 2.45 | n/a | 2:49 | 256 × 248 | No | Yes 2 | n/a | No | 3 | 360 |
LSP Routine | TR | TE | TI | TA | Matrix | BLADE | IPAT | Turbo Factor | Gated | ST (mm) | BW (Hz/px) |
---|---|---|---|---|---|---|---|---|---|---|---|
Sagittal T2 TSE 26 cm | 4300 | 85 | n/a | 2:41 | 384 × 288 | no | Yes 2 | 16 | No | 3 | 266 |
Sagittal T1 FLAIR 26 cm | 1800 | 19 | 733 | 4:32 | 320 × 256 | No | Yes 2 | 7 | No | 3 | 265 |
Axial T2 FS 18 cm | 4300 | 80 | n/a | 3:54 | 256 × 192 | No | no | 16 | No | 3 | 305 |
Axial T1 18 cm | 700 | 23 | n/a | 4:37 | 256 × 192 | No | No | 4 | No | 3 | 300 |
9 elements | — | — | — | — | — | — | — | — | — | — | — |
LSP for Metastases, Primary Tumor, Infection, Inflammation, Substitute STIR 2.5 mm for Sagittal T2 TSE plus T1 FLAIR Sagittal with FS Before and After Gadolinium for these and After Surgery | ||||
---|---|---|---|---|
Light Metal: CSP | TE | BW | TF | ST |
T2 sagittal TSE | 74 | 488 | 24 | 2.5 |
T1 sagittal FLAIR | 14 | 488 | 6 | 3 |
T2 axial FS | 84 | 434 | 21 | 2.5 |
T1 FSE | 11 | 362 | 4 | 3 |
Heavy Metal: CSP | TE | BW | TF | ST |
---|---|---|---|---|
T2 sagittal TSE | 76 | 751 | 36 | 2.5 |
T1 sagittal FLAIR | 14 | 401 | 6 | 3 |
T2 axial FS | 84 | 454 | 24 | 2.5 |
T1 axial FSE | 11 | 362 | 4 | 3 |
Light Metal: LSP | TE | BW | TF | ST |
---|---|---|---|---|
T2 sagittal TSE | 73 | 482 | 24 | 3 |
T1 sagittal FLAIR | 19 | 347 | 6 | 3 |
T2 axial FS | 69 | 488 | 24 | 3 |
T1 axial FSE | 23 | 349 | 4 | 3 |
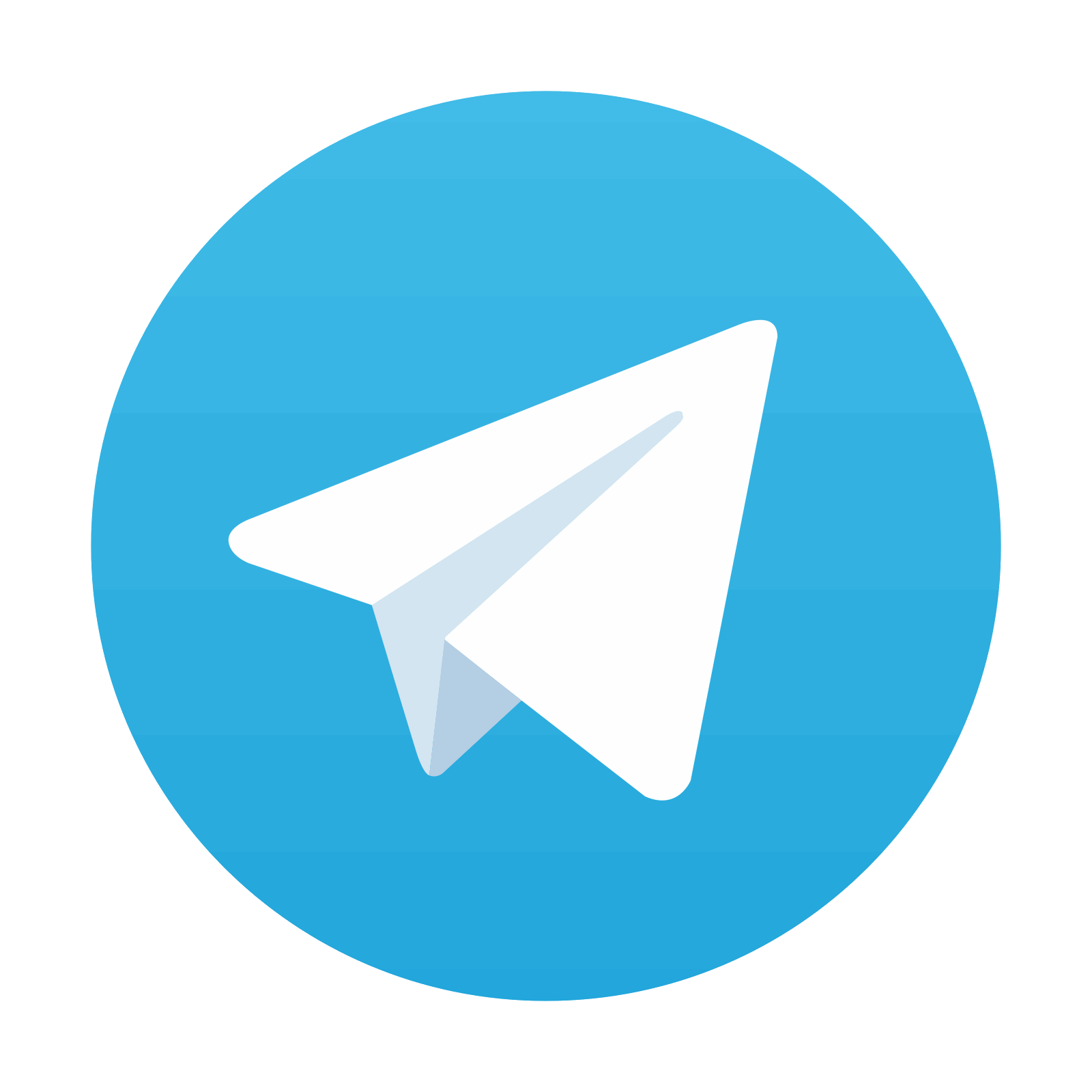
Stay updated, free articles. Join our Telegram channel

Full access? Get Clinical Tree
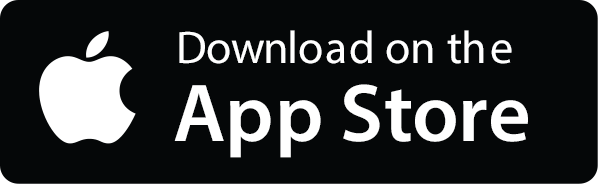
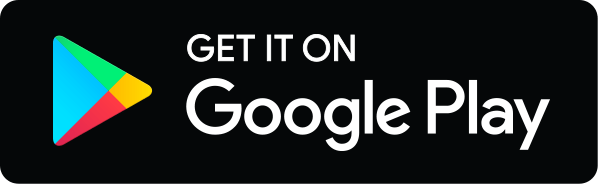
