The introduction of high-field magnetic imaging (≥3 T) has made noninvasive arterial spin labeling (ASL) a realistic clinical option for perfusion assessment in vascular disorders. Combined with the advances provided by territorial imaging of individual intracerebral arteries and the measurement of vascular reactivity, ASL is a powerful tool for evaluating vascular diseases of the brain. This article evaluates its use in chronic cerebrovascular disease, stroke, moyamoya disease, and arteriovenous malformation, but ASL may also find applications in related diseases such as vascular dementia.
- •
ASL provides additional information in cerebrovascular diseases
- •
ASL is easy to implement and quick to perform
- •
ASL is nowadays available from all vendors
- •
ASL is recommended especially at high-fields (≥3 T)
- •
Vascular disorders in general: add global arterial spin labeling (ASL; eg, 3 minutes pulsed ASL [PASL]/pseudo continuous arterial spin labeling [pCASL] with background suppression) to the standard clinical protocol if possible
- •
In large vessel stenosis or occlusion, an additional acquisition after vascular challenge should be considered
- •
Experimentally, territorial ASL can also be considered in large vessel disease, but the sequence is still not clinically available from the vendors
This article reviews the basic principles of ASL, their implementations, and what information can be obtained in addition to CBF using such methods. The main clinical applications of ASL in vascular disorders are reviewed, in particular chronic cerebrovascular disease, stroke, moyamoya disease, arteriovenous malformation (AVM), and neurodegenerative diseases of potential vascular origin, such as vascular dementia. In addition, pulse sequence recommendations are given in Table 1 .
Sequence | TR (s) | Readout | Bolus Definition | TI/PLD | Special |
---|---|---|---|---|---|
QUASAR/ITS-FAIR | 3–4 | EPI | 500–600 ms | 100–3000 ms | Saturation pulse |
Multi-TI | TI+1 | 3D-GRASE | 500–600 ms | 100–3000 ms | Background suppression |
CASL/pCASL | 4–5 | EPI or GRASE or Spiral | 1.8 s | 1.2 s | Background suppression |
ASL
Two main implementations of ASL exist. The first approach uses continuous labeling of the blood water during its passage through a label plane typically labeling extracranial arteries close to, or at, the cerebromedular junction (continuous ASL [CASL]). Labeling is typically performed continuously for 1 to 2 seconds, after which a postlabeling delay is inserted before imaging. This delay allows the labeled blood to reach the region of interest in the brain and lets it clear from the feeding arteries. The second approach is based on a single inversion pulse that spatially labels the blood over an extended region in the neck (pulsed ASL [PASL]). Again, a postlabeling delay is inserted before imaging, for the same reasons. To get a perfusion-weighted image, a control experiment needs to be performed without any labeling of the blood, and it is the subtraction of the 2 (control minus label) that gives the perfusion-weighted image ( Fig. 1 ). The signal differences for normal CBF levels are in the order of 0.5% to 2.0% of the full tissue signal and therefore several control-label pairs need to be acquired and averaged to achieve sufficient SNR in the perfusion-weighted images.
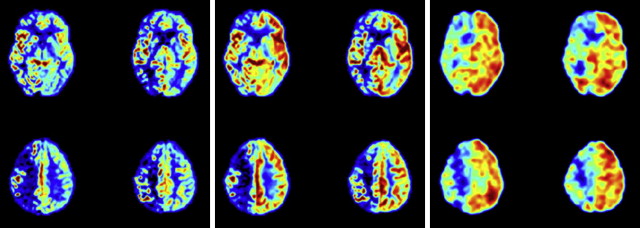
Until recently, PASL was the preferred method because of its ease of implementation and minimal hardware requirements. CASL necessitated special labeling coils or the use of a transmit/receive head coil, which is now obsolete in clinical practice because of the introduction of parallel imaging using large arrays of receiver coils used in combination with the quadrature body coil as the transmit coil. This method has changed with the recent alternative implementation of CASL called pseudo-CASL, which has gained popularity because of minimal hardware requirements and superior SNR, compared with PASL.
There are several aspects to consider when interpreting or quantifying ASL data. This article focuses on the main aspects of clinical interest, whereas a more complete description can be found elsewhere (for example, see Ref. ). The main strength of ASL is its noninvasiveness, which allows the performance of repeated perfusion studies such as in neuroscience, pharmacologic, or patient follow-up studies, but it also makes it an ideal choice for the pediatric population or patients with contraindications for contrast injections. However, the same noninvasive use of the blood water as an endogenous contrast bolus also becomes its main clinical disadvantage, because the magnetically labeled bolus decays with the T 1 of blood, and therefore has a limited lifespan. Regions to which the travel time of the blood from the labeling area exceeds ∼2 seconds are hard to get perfusion information from. Because of their location, such areas experience slow flow because of a stenosis or long collateral pathways making the CBF map appear dark, suggesting no or little flow, whereas it could be late-arriving flow. This error is intrinsic to the method and cannot be overcome.
In addition to being a potential problem, information on the transit time of the blood might have significant diagnostic usefulness, just as in other perfusion measurement methods (eg, dynamic susceptibility-based MR imaging and perfusion CT). In ASL, there are several ways to gather information about the transit time, with the most common ones making use of repeated experiments at different postlabeling delays or inversion times (TI). This method allows assessment of the arterial travel time (ATT) from the labeling region to the tissue of interest, which improves quantification and helps guide the interpretation of the ASL data. However, it does not solve the problem of late-arriving blood beyond the sensitivity limits of ASL in which the bolus disappears with the T 1 relaxation of blood. Therefore, clinical experience is needed to be aware of it and possibly interpret the result in other ways than just as a quantitative CBF measure. As already mentioned, delayed perfusion is a predictor of bad outcome (eg, in stroke).
Another issue common to all perfusion modalities is related to motion artifacts during acquisition. The need for several control-label pairs makes ASL acquisition last typically 2 to 5 minutes, and it is therefore prone to motion artifacts in uncooperative patients. Background-suppressed ASL methods minimize this effect, but motion can still affect the resulting perfusion map and interpretation has to be done with care in these cases.
However, ASL has the unique advantage of being capable of labeling single vessels independently, and therefore it is able to assess the extent of different perfusion territories from all major vessels supplying the brain, thereby including important clinical information on collateral perfusion ( Fig. 2 ). In addition, vascular reactivity can easily be assessed using an acetazolamide challenge together with ASL, and both methods used together make ASL an ideal tool for assessment of vascular disorders, especially because they can be combined with quantitative measurement of CBF. In addition, ASL perfusion measurements may be used in evaluation of the effectiveness of medication treatments in patients with cerebrovascular disease.
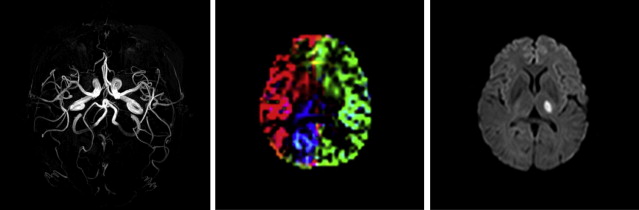
Chronic cerebrovascular disease
The most obvious example of chronic cerebrovascular disease is a stenosis or occlusion of one of the brain feeding arteries. The most well-known example of extracranial stenoses is the stenosis at the origin of the internal carotid artery (ICA). Patients with recent symptoms on the side of an ICA stenosis are considered for carotid surgery if the stenosis has a certain severity, typically greater than 70%. However, this procedure only prevents recurrent ischemic stroke in less than 20% of patients, meaning that most of the patients currently being operated on would not have experienced any future stroke if they had not had the surgery. In addition, the operation has a risk of causing an ischemic event and it would therefore be beneficial to improve patient selection to carotid endarterectomy. ASL may be helpful to select patients for carotid surgery in 2 ways. First, perfusion imaging at rest can discriminate between brain tissue that is underperfused and brain tissue with a normal perfusion. Patients with severely decreased perfusion on the side of the carotid artery stenosis may benefit most from carotid endarterectomy compared with patients with intact perfusion collaterals, for instance via the circle of Willis, which thereby compensates for the stenosis. Most strokes from a carotid artery stenosis are considered to be thromboembolic. The presence of a vulnerable plaque and therefore high risk of thromboembolism in combination with hypoperfusion (eg, measured with ASL) increases the patient’s risk of a recurrent stroke, according to the washout hypothesis introduced by Caplan and Hennerici. In these patients, the low perfusion decreases the ability of the vasculature to wash out small emboli.
Nevertheless, the presence of normal perfusion in a resting condition does not always mean that the vasculature is not compromised. The first-line compensation mechanism for a decrease in perfusion pressure is dilation of the arterioles, resulting in lower resistance and higher flow, thereby maintaining a constant CBF. However, in some patients this autoregulatory ability is compromised because it reaches its maximum diameter to recruit flow (eg, via collaterals). Further vascular events with an accompanying reduction in perfusion pressure could cause ischemia.
The advantage of MR imaging is that, within the same scan session, carotid plaque imaging, perfusion imaging, and a vascular reactivity challenge can be performed using, for example, acetazolamide and ASL, allowing assessment of plaque rupture risk, baseline perfusion status, and the ability to compensate in future vascular events. Autoregulation status can be assessed by combining a baseline or rest perfusion scan with a perfusion measurement after a vasodilatory challenge. Cerebrovascular reserve capacity (CVR) is then defined as the percentage CBF change between baseline and vasodilatation. ASL is advantageous for such measurements because of its noninvasiveness, which allows repeated perfusion measurements before and after the vasodilatory challenge after a short time span. In comparison, the use of SPECT for the same type of examination would take place over several days, with the baseline scan typically performed after the challenge scan. When using ASL, breath holding, carbon dioxide inhalation, or acetazolamide injection can be used for measuring the cerebrovascular reserve capacity. Only a subgroup of patients with carotid artery stenosis who were studied with ASL showed compromised cerebrovascular reserve capacity of the affected hemisphere ; possibly the ones benefiting the most from surgery. Although further follow-up studies are needed, it can be inferred from reactivity studies using transcranial Doppler (TCD) that this group has the highest risk of a recurrent stroke.
In addition to ASL measurements of global CBF, the possibility of assessing the area of the perfusion territories with ASL may be important in patients with carotid artery stenosis or other cerebrovascular diseases. Patients often present with double-sided stenoses and it is not always clear which side is the symptomatic one, so knowledge of collateral flow could be useful in this decision. In a previous study of subacute patients who had strokes, classifying according to anatomic location of the infarct alone would result in misclassification of the feeding territory in approximately 10% of patients. This error could have implications for patients with multiple stenosed extracranial arteries for whom optimal benefit of operation is expected if the symptomatic vessel is operated on. Intuitively, unless plaque imaging shows otherwise, it would be most beneficial to intervene on the side feeding the smallest area , although further studies are needed to confirm this.
As mentioned earlier, ASL is also capable of measuring the arrival time of the blood at the brain tissue. The arterial transit time parameter includes information on the collateral flow, which may result in a delayed arrival of the arterial blood at the brain tissue level. In patients with more severe obstruction and, for instance, leptomeningeal collateral flow, the ATT is very long. In this regard, the travel time may be an indicator of the quality of the collateral perfusion in patients with extracranial or intracranial stenoses or occlusions. Several studies in patients with carotid artery stenosis or carotid occlusion showed a delayed arrival time in a subgroup of patients in the hemisphere ipsilateral to the carotid lesion. With careful interpretation of ASL images at a single delay time, the delayed arrival of the arterial blood can be appreciated with label still present in the arterial vasculature feeding this specific brain region. Especially in patients with a carotid artery occlusion, the arrival times might be delayed to such an extent that the typical time delay between labeling and imaging (1600–2000 milliseconds) is not enough to allow the protons in the labeled water to reach the brain tissue. In patients with carotid artery stenosis, the arrival time is often within normal limits or only increased by a few hundred milliseconds, which still allows the bolus to clear from the feeding arteries at typical postlabeling delays of 1800 milliseconds.
For patients with atherosclerotic carotid artery occlusion, MR imaging examinations are often performed to show the eventual presence of infarcts. Because bypass surgery has not shown a clear benefit for these patients, surgery is nowadays less of an option and the focus is on keeping the remaining vessels open. Identification of the subgroup with a severe stenosis in one of the nonoccluded feeding arteries, such as the contralateral ICA or vertebrobasilar arteries, is important so that the possibilities of endarterectomy or stenting can be considered before further disease progression or a fatal event. Timely intervention on a severe stenosis in one of these nonoccluded arteries may benefit the patient by augmentation of the collateral flow toward the diseased hemisphere, and this assessment can be done with ASL. Territorial ASL shows the presence and extent of collateral contributions from the external carotid artery, contralateral ICA, and vertebrobasilar arteries, and CVR can be assessed with preacetazolamide and postacetazolamide challenge. In this way, patients with well-developed collateral perfusion and reserve capacity (eg, in chronic ICA stenosis) can be distinguished from the more critical group of patients with inadequate collateral flow and compromised reactivity after a vasodilatory challenge. An example of such a high-risk patient who could benefit from revascularization is shown in Fig. 1 . The patient has a right internal carotid occlusion and presents with both hypoperfusion and reduced vascular reserve capacity.
In patients with vertebrobasilar stenosis or occlusions, collateral flow at the level of the circle of Willis may compensate flow delivery to the posterior brain areas via the posterior communicating arteries. However, a recent study in patients with vertebrobasilar artery disease using ASL showed lower CBF in the posterior circulation in patients compared with a group of healthy controls. The presence of decreased perfusion or reactivity could therefore indicate the subgroup of patients with vertebrobasilar atherosclerotic lesions that would benefit most from revascularization such as stent placement. Furthermore, in patients with both vertebrobasilar and carotid atherosclerotic lesions, information about the perfusion territories would show which artery is feeding the ischemic area. For instance, in an area such as the thalamus, which can be fed from both the carotid and the vertebrobasilar arteries, it may not be clear which artery is responsible for the presence of an infarct when multiple stenosis of extracranial arteries are present.
Patients with chronic intracranial atherosclerotic lesions may also be at high risk of a recurrent stroke, and ASL can be useful in the depiction of areas with severely decreased perfusion. Furthermore, territorial ASL may show the extent of collateral contributions to specific brain regions. For instance, with an atherosclerotic lesion in the middle cerebral artery, it can visualize possible contributions from the vertebrobasilar arteries via posterior-anterior leptomeningeal collaterals. In these cases, ASL arrival time parameters combined with a vascular challenge can be used to assess the quality of the collateral perfusion (eg, whether autoregulation has been exhausted and how fast the bolus arrives compared with normal tissue). Currently, treatments strategies of both medical and interventional (stent) origin are being evaluated for patients with chronic intracranial arterial lesions. Although only a limited number of ASL studies have been performed in these patients groups, ASL may be performed in the future to select patients best suited for different treatments according to perfusion, vascular reserve, and the extent of collateral perfusion.
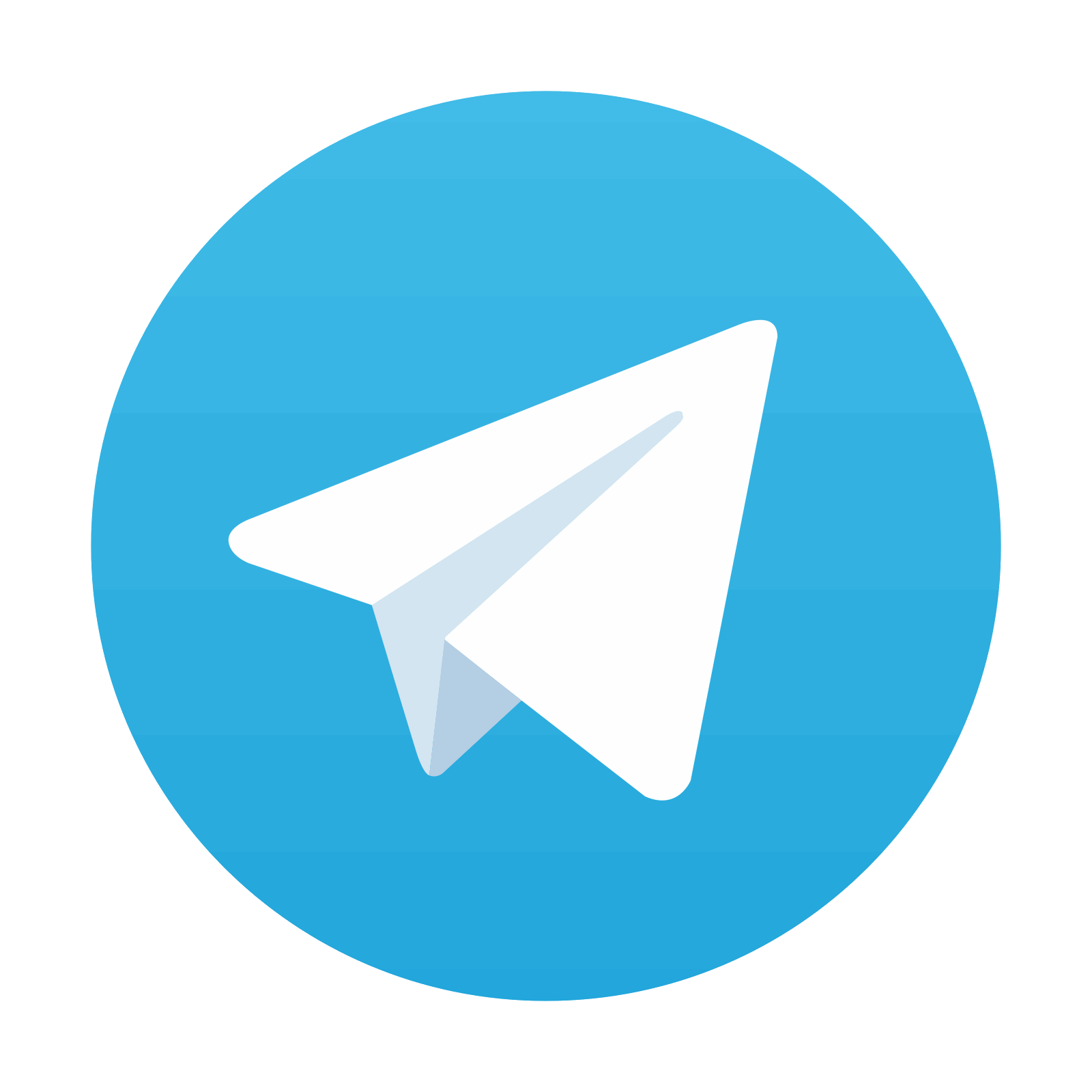
Stay updated, free articles. Join our Telegram channel

Full access? Get Clinical Tree
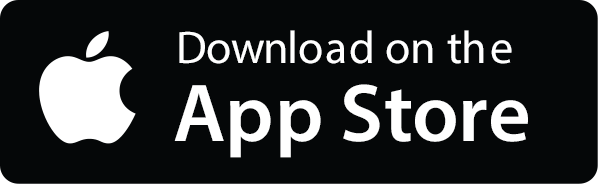
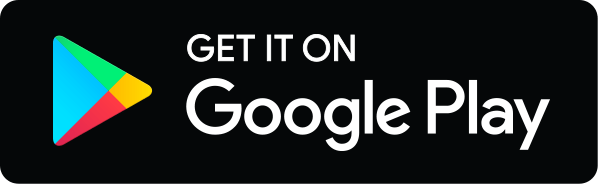