Lumbosacral Anatomy
Osseous Anatomy
The lumbosacral spine consists of five lumbar (L1–L5) and five sacral vertebrae (S1–S5) and their associated intervertebral discs, nerves, muscles, ligaments, and blood vessels. Each vertebra consists of a vertebral body, vertebral arch, and seven processes. The vertebral bodies are responsible for absorbing most of the axial forces exerted on the vertebrae. The vertebral arches and the dorsal part of the vertebral body along the vertebral column collectively form the spinal canal that houses the thecal sac. The arches contain pedicles on each side that predominantly blend into the superior articular process. From this, bony intersections arise the transverse processes, which are present bilaterally. Each vertebra has additional inferior processes. The superior and inferior processes articulate with the inferior and superior processes of the adjacent vertebrae, respectively, to create the facet joints. Approximately 20% of the axial load placed on the lumbosacral spine is transmitted through the facet joints. The seven processes of vertebrae serve as both origin and insertion points of the paraspinal muscles. They therefore are integral to the overall function of the spine, including its ability to move, bear-weight, and maintain proper alignment.
The lumbar vertebrae have several unique properties that distinguish them from vertebrae of other vertebral levels. They have characteristically larger vertebral bodies, and shorter, thicker spinous processes. The larger bodies are a testament to the lumbar region’s duty to bear a significant proportion of the weight of the upper limbs and trunk. Intervertebral discs are cartilaginous structures that provide additional cushioning between each vertebra. Stability is further conferred to the lumbar spine through the anterior and posterior longitudinal ligaments that run vertically on the ventral and dorsal aspects of the vertebral column, respectively.
The five sacral vertebrae are fused together to form the sacrum, an upside-down triangular bone that resides at the base of the lumbar spine. With the exception of the L5-S1 intervertebral disc, due to ultimate fusion of the sacral vertebrae by adulthood, the sacral segments are not typically separated by intervertebral discs. The sacral promontory, the most anterior part of the sacrum, articulates with L5, forming part of the lumbosacral joint. In addition to the intrinsic stability imparted by the intervening intervertebral disc, this joint is further strengthened by the iliolumbar and lumbosacral ligaments.
The inferior portion of the sacrum articulates with the coccyx, which is another small triangular bone at the bottom of the spine that is thought, by many, to be a vestigial structure. Within the sacrum itself lies the continuation of the vertebral canal, the sacral canal , that terminates at an apex termed the sacral hiatus . While in the adult spine, the spinal cord typically ends at the level of L1-L2 as the conus medullaris , both dura mater and the filum terminale, which is a ligamentous condensation of pia mater, extends down from the conus through the sacral canal to the coccyx and serves as an anchor for the spinal cord. Computed tomography (CT) has been found to be a primary imaging modality for demonstrating osteological pathology, including trauma, fractures, scoliosis, and in pre- and postoperative patients.
Lumbosacral Intervertebral Discs
The intervertebral disc allows for flexibility of the spine while concomitantly conferring strength and shock absorptive ability. Intervertebral discs are composed of two main components: the nucleus pulposus centrally, and the annulus fibrosus , circumferentially. The nucleus pulposus has a gelatinous composition that allows for dissipation of force and protection against injury. The annulus fibrosus is made of organized collagen I fibers that encircle and maintain the integrity of the inner nucleus pulposus. The nerve supply to the intervertebral disc is limited to only the outer annulus through the sinuvertebral nerve. Attaching the disc to adjacent vertebrae are the cartilaginous endplates. These endplates serve two counteracting functions—to both be porous enough to allow for nutrient transport between disc cells, while remaining sufficiently sturdy to mitigate mechanical failure. Pathological changes within the endplates including Schmorl nodes, calcification, fractures, and tears have clinically been correlated with disc degeneration and back pain.
As humans age, the proteoglycan and collagen composition within the intervertebral disc are altered. Specifically, the pulposus loses its water content and ultimately becomes more fibrotic. Consequently, the disc thins and then ultimately will calcify during the terminal stages of the degenerative process. These changes ultimately impair mobility and increase the likelihood of onset of various disc-related pathologies that will be discussed in the later sections. Pathologies such as degeneration of intervertebral discs and radiculopathy can be visualized and studied on CT and magnetic resonance imaging (MRI) with contrast enhancement.
Anomalous Lumbosacral Anatomy
Anatomical variation seen in the lumbar and sacral region is partially due to sexual dimorphism and partially due to congenital defects that influence the movement and function of the vertebral column. The sacrum can vary slightly among males and females. Females usually have a shorter and wider sacrum and a less prominent sacral promontory that functions to help with easier delivery during childbirth. Another anatomical variant seen in the lumbosacral region is the existence of lumbosacral transitional vertebrae. These can result from either of the following: (1) L5 fusing with the sacrum (sacralization), which yields six sacral vertebrae, and (2) S1 transitioning to a lumbar vertebra (lumbarization), resulting in only four sacral vertebrae. The lumbarized S1 vertebra typically has features similar to lumbar vertebrae. Either of these processes can occur partially or completely, unilaterally or bilaterally. These physiologic variations may impair the range of motion and weight-bearing abilities, which may contribute to spine pathology and lower back pain. Due to its superior spatial resolution, CT may be the best imaging modality for characterization of lumbosacral transitional vertebra pathologies.
Neurovasculature of the Lumbosacral Spine
The main blood supply to the lumbar vertebrae are the subcostal and lumbar arteries. The sacral vertebrae are supplied by the medial and bilateral sacral arteries. The red marrow of each vertebra’s body receives a separate source of nutrients from vertebral canal branches from these main arteries. The spinal veins that run longitudinally along the vertebral canal form valveless venous plexuses. Blood flow direction is thus bidirectional and is contingent on pressure gradients.
The nervous supply to both the sacral and lumbar vertebrae is from branches derived from the terminal spinal nerves. Meningeal branches of these spinal nerves innervate the lumbar and sacral vertebrae themselves. In the caudal spine, the spinal nerves increase in diameter, while the diameter of the intervertebral foramina decreases. This anatomical relationship contributes to certain pathologies of the lumbosacral spine to be discussed later. By providing visualization of nerve roots, the cauda equina, and the spinal cord, CT myelography is useful in diagnosing these various pathologies.
Lumbosacral Musculature
Both the lumbar and sacral vertebral regions serve as origin and attachment sites for numerous muscles. The erector spinae, interspinalis, intertransversarii, latissimus dorsi, rotatores, and serratus posterior inferior muscles all originate within the lumbar spine. The piriformis muscle originates from the anterior surface of the sacrum. Furthermore, the coccygeus, the iliac muscles, gluteus maximus, multifidus lumborum, and erector spinae muscles all have various attachment site or sites of origin in the sacrum. These paravertebral muscles atrophy with age through both degradation of skeletal muscle and infiltration of fat tissue. These age-related changes increase the spine’s susceptibility to pathology and may serve as an additional pain generator. Furthermore, they may be correlated to poorer outcomes following spine surgery. CT scans can be used to assess muscular integrity via visualization of the functional cross-sectional area (FCSA) and fatty infiltration of atrophied paravertebral muscles.
Indications for Lumbosacral Computed Tomography
Compared with plain radiography and magnetic resonance imaging (MRI), the ability of computed tomography (CT) to provide ultrafine spatial anatomic detail allows for optimal evaluation of bony anatomy and pathology. Furthermore, image reformatting and three-dimensional reconstructions allow the surgeon to better visualize spinal anatomy in the setting of difficult to visualize regions of the axial skeleton (e.g., sacrum) and when evaluating complex pathologies, e.g., severe spinal deformities, high-grade spondylolistheses, complex fractures, and neoplasms.
In light of these latter advantages, use of CT has become the gold standard for the initial evaluation of the traumatized lumbosacral spine and for evaluating bony fusion. Importantly, in the setting of neural compression, CT allows for better distinction between compression secondary to soft tissue versus bony pathology. Furthermore, CT is more sensitive than MRI and plain radiography when it comes to detecting pars defects and subtle pars fractures.
Finally, in patients with contraindications to MRI (the presence of certain pacemakers or defibrillators), CT myelogram is a useful surrogate to assess for soft tissue pathology. Specific benefits of CT are outlined in further detail later.
Stenosis Secondary to Bony Pathology
A comprehensive understanding of the pathologic condition being addressed is mandatory in the surgical planning process. In addition to ligamentous overgrowth and disc-related pathology, lumbar degenerative stenosis is often the consequence of hypertrophied or asymmetric facets or osteophytosis. These findings are often noted within the setting of adjacent segment degeneration (a purely radiographic finding) or adjacent segment disease (radiographic finding with clinical consequences) that oftentimes develops at levels adjacent to previous fusions. These rates may approach 36.1% in the lumbar spine and 25.6% in the cervical spine, at 10-year follow-up following arthrodesis. In this setting, CT allows for the most accurate depiction of these latter bony structures. Distinguishing between primarily osseous versus soft tissue neural compression may be the deciding factor between the choices of surgical approach, maximizing the safety of the stenosis operation and optimizing success rates. Finally in conjunction with upright lateral plain radiographs, similar to supine MRI, supine CT may be useful in the diagnosis of dynamic stenosis that may occur in the setting of segmental instability (e.g., mobile spondylolisthesis). It has even been suggested that this latter method may be, in fact, superior to the more widely accepted standing flexion/extension lateral radiographs for the detection of segmental translation.
While a much more common cause of neural compression in the cervical spine, ossification of the posterior longitudinal ligament (OPLL) can also lead to neural compression in the cervical and lumbosacral spine ( Fig. 1 ). The prevalence of OPLL in the United States has been reported to range from 0.1% to 0.7% with a mean age of the onset of 50 years. MRI, while still useful to qualify neural compression in this setting, may be limited in characterizing the extent of ossified ligamentous tissue. In these instances, CT is indispensable for surgical planning and characteristic CT features of OPLL have been described. For example, while initially described in the setting of cervical neural compression, the double-layer sign may be pathognomonic for dural penetrance of the thecal sac by OPLL ( Fig. 2 ). It is defined as the presence of a hypodense linear mass (representing a nonossified PLL) sandwiched between two hyperintense ossified rims. When this is identified preoperatively, spinal surgeons should anticipate an increased likelihood for cerebrospinal fluid leak intra-operatively, if the surgical approach mandates a decompression at the affected level.
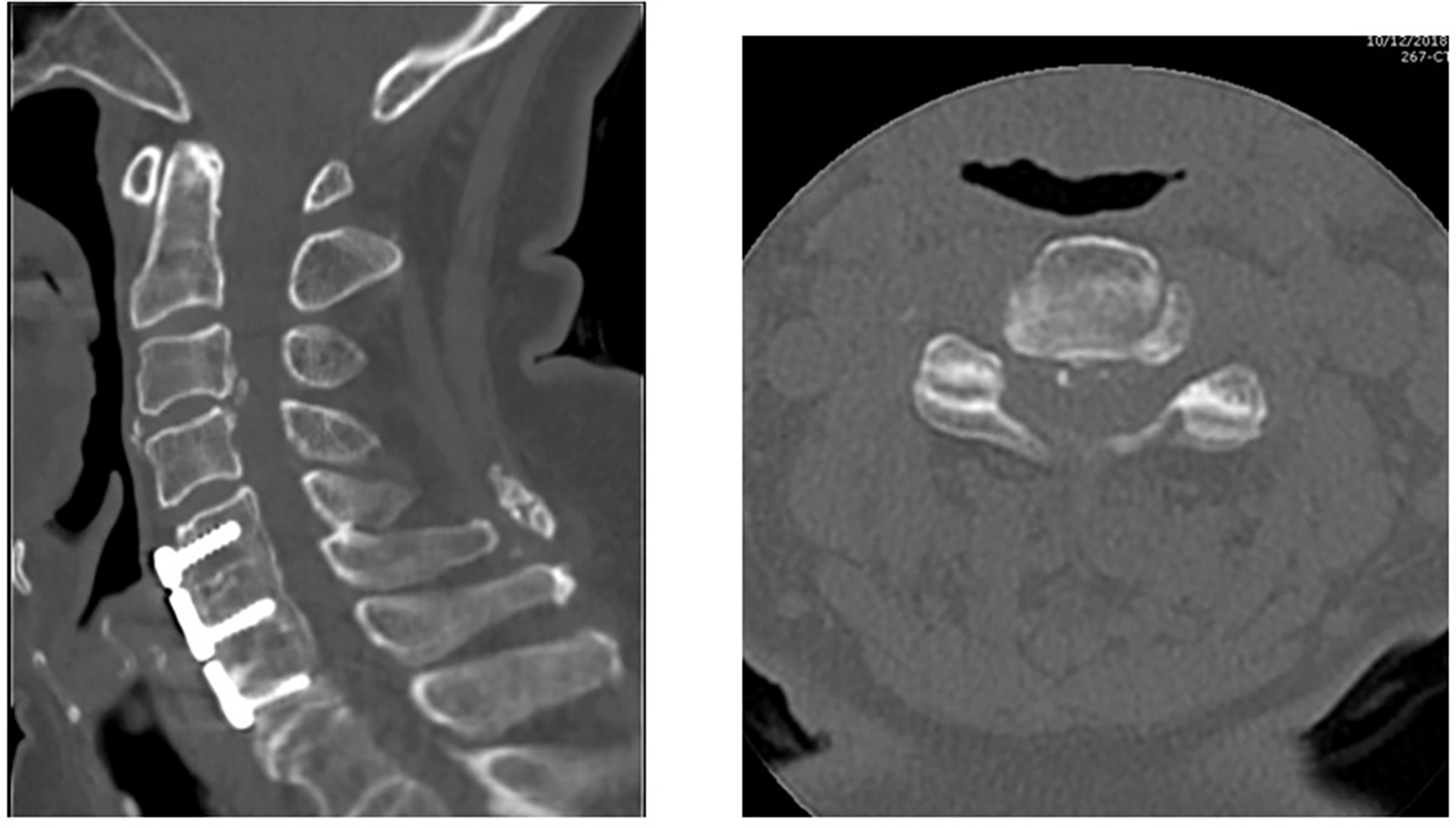
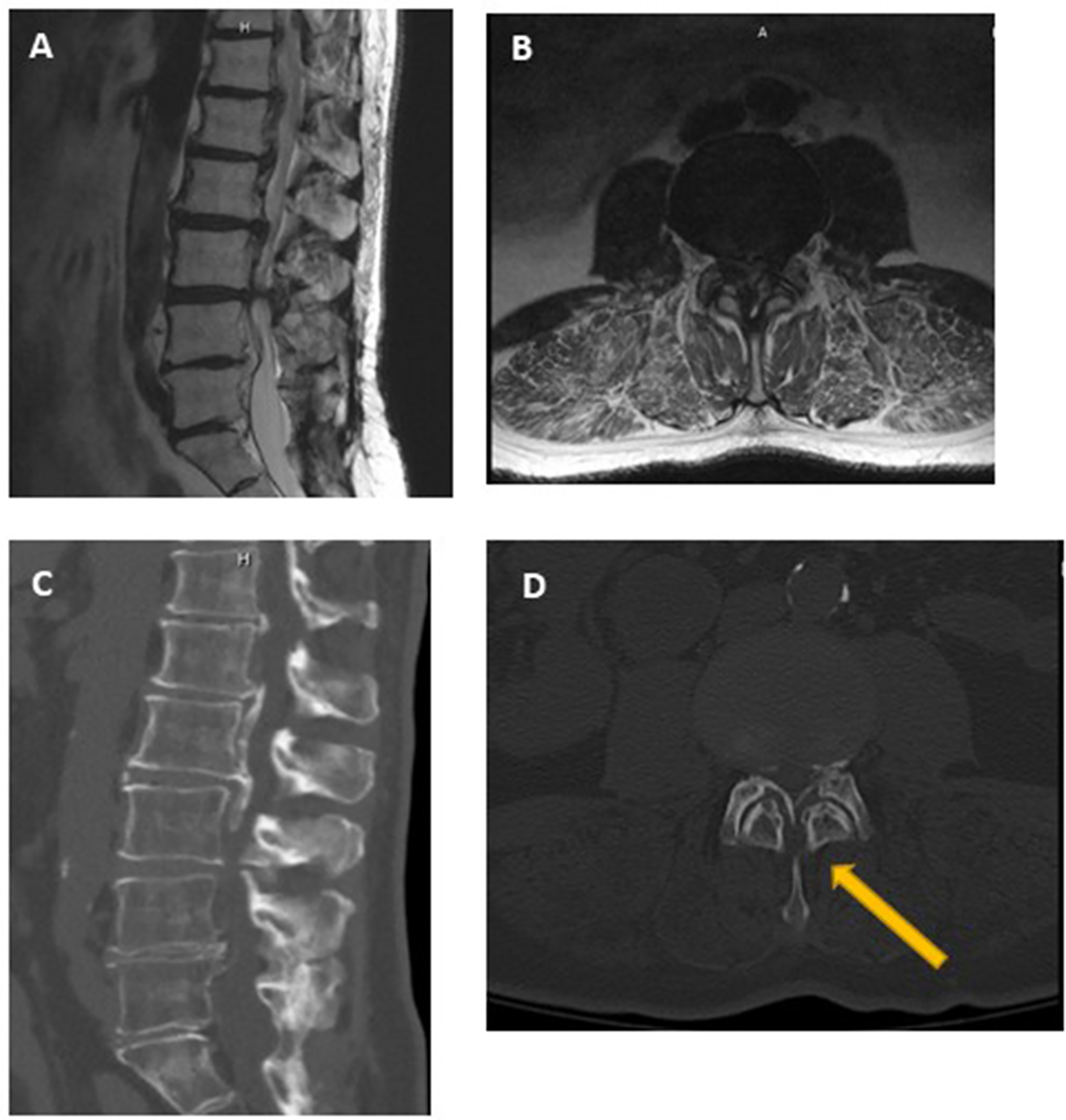
In general, OPLL can be divided into four types based on CT findings :
- (1)
Continuous : OPLL spans multiple vertebral bodies in addition to crossing intervening disc spaces,
- (2)
Segmental : OPLL is limited to the vertebral body margins,
- (3)
Mixed : combination of the aforementioned,
- (4)
Others : OPLL may be primarily limited to the disc level.
While these latter classifications of OPLL may be useful prognosticators in the setting of myelopathy, no such correlations that we are aware of have been described in the setting of lumbosacral OPLL.
Finally, there is a high correlation between OPLL and ossified ligamentum flavum, the combination of which can potentially lead to circumferential neural compression. Again, a CT scan is invaluable in identifying these less common etiologies of stenosis and for planning the optimal surgical approach. Furthermore, as the risk of dural violation is potentially higher in these instances, this knowledge can be shared early on with patients during the preoperative planning process while discussing the risks and benefits of surgery.
Indications for CT Myelography
CT myelography combines the diagnostic benefits of CT with myelography. The addition of the myelogram allows for improved visualization of the neural elements ( Fig. 3 ). A myelogram is typically obtained by injecting noniodinated radiopaque contrast dye into the subarachnoid space via lumbar puncture. To obtain a CT myelogram, a traditional CT scan is then performed immediately following this.
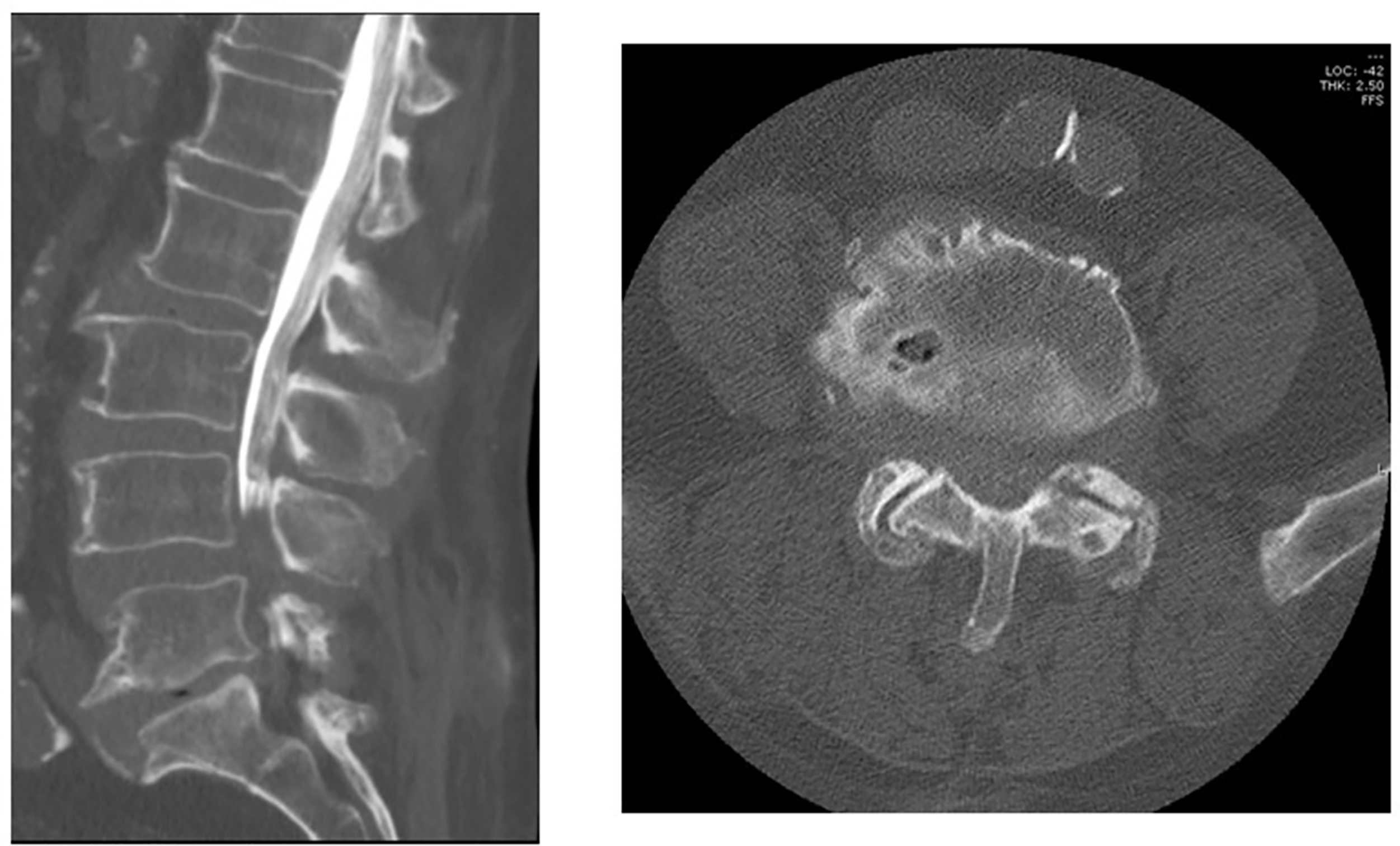
The most common indications for CT myelography are: (1) the evaluation of neural compression when MRI is contraindicated (the presence of certain pacemakers or defibrillators) and (2) when osseous neural compression is suspected. When planning revision spinal surgery, CT myelograms can additionally prove useful, particularly in the setting of complex spinal deformity, or when substantial metal artifact on MRI interferes with accurate interpretation of imaging, i.e., if metal artifact reduction sequence [MARS] MRI is not readily available.
CT myelography may also be useful for the diagnosis of a postoperative cerebrospinal fluid leak (CSF) and the presence of a pseudomeningocele. In the former, contrast extravasation outside of the thecal sac is diagnostic.
Finally, CT myelography can be as useful as an MRI for the diagnosis of arachnoiditis. Traditionally, three distinct patterns of arachnoiditis have been described based on MRI findings.
- (1)
Central adhesion of nerve roots : nerves are clumped into one cord-like structure,
- (2)
“Empty thecal sac” sign : nerves are adherent to the meninges. With CT myelogram, only the high-intensity contrast agent is seen within the thecal sac. Nerves appear to be missing,
- (3)
A nondistinct arachnoid mass within the thecal sac : this is typically considered to signal the end stage of the inflammatory response. The arachnoid mater appears as a nonspecific mass-like structure that may result in a contrast block.
Similar findings can be seen with CT myelography, although with perhaps less distinctive ability. Ultimately, however, radiographic findings do not appear to correlate with clinical symptomatology.
Infection and Tumor
Metastases from primary pulmonary, renal, thyroid, breast, and prostatic malignancy are the most common tumors found in the spine. Primary tumors arising in the spine are rare. These include tumors of spinous structures and bone, which include intradural extramedullary tumors, intramedullary tumors, and extradural primary tumors such as chondrosarcoma, osteosarcoma, and chordoma.
Compared to plain radiographs, CT allows for improved visualization of bone mineralization and may subsequently allow for earlier detection of osseous lesions. CTs are particularly useful in the diagnosis of certain osteogenic tumors, such as osteoid osteomas or osteoblastomas, as they are not depicted with the use of plain radiography and have very characteristic appearances on CT. Consequently, CT has become the diagnostic test of choice for these latter pathologies.
Furthermore, as discussed prior, image reformatting options and three-dimensional reconstructions grant surgeons the ability to depict even minute discrepancies in bone mineralization that may allow for earlier diagnosis of tumors. Additionally, understanding whether or not a tumor is osteolytic or osteoblastic can help in both tumor diagnosis and surgical planning. Finally, while not as sensitive as MRI in this regard, CT may also allow for the identification of any concomitant soft tissue mass. Consequently, the acquisition of CT scans has become a staple of any preoperative plan that involves tumor resection.
While MRI remains the imaging modality of choice for the evaluation of spine infections, CT offers valuable diagnostic information and is an essential part of the preoperative workup. From a diagnostic standpoint, CT may help in the distinction between infection and tumor. For instance, infection generally results in vertebral body osteolysis, whereas tumor can be osteoblastic and may be more likely to involve the posterior elements.
CT may additionally help in differentiating between pyogenic spondylitis and a granulomatous infection (e.g., tuberculosis or fungal infection). Granulomatous infections may exhibit radiographic traits similar to tumor such as pedicle invasion and associated soft tissue masses. Paraspinal calcifications and vertebral fragmentation are unique features of tuberculous infections that may also be seen on CT. Finally, in the setting of a concomitant neurologic deficit, CT myelogram can be obtained in patients with contraindications to undergoing MRI.
Lumbosacral Trauma
The spiral CT scan has become the standard for the initial radiologic evaluation of the polytraumatized patient. With the use of thin-section techniques and multiplanar reformatting, CT allows for a quick evaluation of the bony anatomy with high sensitivity in detecting fractures. Furthermore, as routine acquisition of CT imaging of the chest, abdomen, and pelvis is performed in the traumatic setting, reformatting of the existing data to specifically evaluate the spine can often be included and enhanced based on the current CT scan. This ultimately decreases overall radiation exposure and evaluation times.
In general, CT remains the diagnostic study of choice for detecting posteriorly displaced fracture fragments that may result in spinal cord compression (i.e., burst fractures) and for the evaluation of bony involvement of the posterior osseous structures ( Fig. 4 ). Misdiagnosis of burst from compression fractures can occur up to 25% of the time when relying solely on plain radiography. It is important to note, however, that the diagnosis of posterior ligamentous injury, which is crucial to injury management, is best ascertained with the use of MRI.
