The use of MR guidance for endovascular intervention is appealing because of its lack of ionizing radiation, high-contrast visualization of vessel walls and adjacent soft tissues, multiplanar capabilities, and potential to incorporate functional information such as flow, fluid dynamics, perfusion, and cardiac motion. This review highlights state-of-the-art imaging techniques and hardware used for passive tracking of endovascular devices in interventional MR imaging, including negative contrast, passive contrast, nonproton multispectral, and direct current techniques. The advantages and disadvantages of passive tracking relative to active tracking are also summarized.
Key points
- •
Steady-state free precession (SSFP) sequences have become the preferred sequences for endovascular interventional MR imaging.
- •
Passive tracking using negative contrast uses marker materials in device construction that cause localized magnetic field inhomogeneities resulting in dephasing of adjacent proton spins and a local signal void or susceptibility artifact on MR imaging without the use of active radiofrequency (RF) components.
- •
Passive tracking can also be achieved with positive contrast using paramagnetic materials that cause focal T1 shortening (bright spot), such as gadolinium, within the lumen or on the surface of a catheter. Additional passive tracking methods include nonproton multispectral, and direct current techniques.
- •
The main advantage of passive catheter tracking over active tracking is simplicity and absence of radiofrequency (RF) heating, as no additional MR imaging scanner software, wires or electronics are required for most passive tracking techniques.
- •
Because device coordinates are not registered with passive tracking methods and imaging plane is not automatically updated, automatic visualization and correct imaging plane selection is difficult.
Introduction
The use of MR imaging for guidance of endovascular interventions has emerged as a feasible alternative or adjunctive imaging modality to digital subtraction angiography (DSA). MR imaging guidance offers several potential advantages. The absence of ionizing radiation during MR imaging is particularly attractive, as there may be substantial radiation exposures to both the patient and the interventionalist during DSA. In addition, although DSA is limited to luminal information, MR imaging can also provide high-contrast visualization of the vessel wall and adjacent soft tissues with moderately high spatial resolution, allowing for the identification of vulnerable atherosclerotic plaques, the ability to monitor the effect of endovascular therapy on adjacent tissues, and to recognize possible complications, such as vascular perforation, hemorrhage, and infarction, all in real time. MR imaging also offers unrestricted multiplanar imaging capabilities and the opportunity to obtain physiologic and functional information such as flow velocity, 4D flow imaging and computational fluid dynamics, temperature, diffusion, and perfusion.
Concurrent advances in the design of real-time MR fluoroscopy and MR angiography pulse sequences and the development of MR safe and compatible endovascular devices have facilitated progress from in vitro and animal feasibility studies of MR guidance for endovascular interventions to their translation into clinical care. This review highlights state-of-the-art imaging techniques and hardware used for passive tracking of devices in endovascular interventional MR imaging.
Introduction
The use of MR imaging for guidance of endovascular interventions has emerged as a feasible alternative or adjunctive imaging modality to digital subtraction angiography (DSA). MR imaging guidance offers several potential advantages. The absence of ionizing radiation during MR imaging is particularly attractive, as there may be substantial radiation exposures to both the patient and the interventionalist during DSA. In addition, although DSA is limited to luminal information, MR imaging can also provide high-contrast visualization of the vessel wall and adjacent soft tissues with moderately high spatial resolution, allowing for the identification of vulnerable atherosclerotic plaques, the ability to monitor the effect of endovascular therapy on adjacent tissues, and to recognize possible complications, such as vascular perforation, hemorrhage, and infarction, all in real time. MR imaging also offers unrestricted multiplanar imaging capabilities and the opportunity to obtain physiologic and functional information such as flow velocity, 4D flow imaging and computational fluid dynamics, temperature, diffusion, and perfusion.
Concurrent advances in the design of real-time MR fluoroscopy and MR angiography pulse sequences and the development of MR safe and compatible endovascular devices have facilitated progress from in vitro and animal feasibility studies of MR guidance for endovascular interventions to their translation into clinical care. This review highlights state-of-the-art imaging techniques and hardware used for passive tracking of devices in endovascular interventional MR imaging.
The vascular interventional MR imaging suite
The use of MR imaging guidance for endovascular intervention requires balancing multiple trade-offs involving image quality, spatial and temporal resolution, patient accessibility, field of view, and cost. Higher-field-strength cylindrical bore scanners are capable of better image quality; however, they require small diameter bores compared with open MR imaging scanners and therefore limit patient accessibility. At present, open bore MR imaging scanners do not provide adequate field strength, gradient strength, or field uniformity for endovascular intervention. Clamshell and double-doughnut–shaped bores were initially introduced to improve patient accessibility during intraoperative MR imaging. Fortunately, arterial access at the groin for most endovascular procedures permits longer distances (40–80 cm) between the interventionist standing at the opening of the magnet bore and the target tissue of interest in the head, neck, chest, or abdomen, making commonly available cylindrical (or closed) bore clinical MR scanners suitable. Cylindrical bore magnets with shorter bore lengths (125 cm instead of 160 cm) and/or with larger bore diameters (70 cm instead of 60 cm) have been developed, allowing easier patient access and are commercially available. Most research sites now have hybrid systems consisting of a short-bore cylindrical MR imaging and DSA unit connected by a single floating table, also known as XMR systems ( Fig. 1 , [CR] ). This system allows for use of either imaging modality for different parts of a procedure and permits the use of DSA backup in case of difficulties during MR guidance.
Real-time MR imaging and MR angiography techniques for endovascular interventional MR imaging
Image acquisition during MR-guided endovascular procedures must be rapid enough to allow the interventionist to visualize changes within the patient as devices are externally manipulated in real time. Several pulse sequence approaches to achieve higher-temporal-resolution MR imaging for MR-guided interventions, also known as real-time MR imaging or MR fluoroscopy, have been explored. Higher temporal resolution usually comes at the expense of spatial resolution. Nevertheless, MR fluoroscopy sequences used today allow sufficient spatial and temporal resolution to track endovascular devices. Because each pulse sequence offers unique contrast characteristics, the ideal sequence depends on the properties of the device being tracked and the passive tracking method being used for device tracking (see passive tracking section), the contrast properties of the tissue of interest, and the need for intravascular contrast. Commonly used MR fluoroscopy sequences are summarized in Table 1 .
Contrast Weighting | Sequence | Comments |
---|---|---|
T1/T2 | Balanced SSFP (aka FIESTA, TrueFISP, or balanced FFE) |
|
T1+T2* | GRASS, FISP, FFE |
|
T2 | TOSSI (RARE, HASTE) TSE |
|
T1 | FSPGR FLASH T1-FFE |
|
SSFP sequences have become the preferred sequences for endovascular interventional MR imaging. SSFP provides excellent bright blood contrast and visualization of devices using passive tracking because of the high temporal resolution (short repetition time [TR]), high signal-to-noise ratio (SNR), and greater sensitivity to magnetic field inhomogeneities ( Fig. 2 ).
T2-weighted turbo spin echo, rapid acquisition with relaxation enhancement (RARE, HASTE) sequences, may also be used for verification of device location because of the flexibility in image contrast and fast acquisition of the pulse sequence. Additional methods to decrease image acquisition time include the use of parallel imaging, key-hole imaging, alternative k-space sampling such as spiral or radial trajectories, asymmetric truncated k-space sampling strategies, and recently the exploitation of k-space data sparsity using compressed sensing. Coupled with high-performance multiprocessor computers, real-time MR guidance is now capable of up to 10 frames per second, which is comparable to the temporal resolution of x-ray fluoroscopic guidance for most clinical endovascular interventions (4–15 frames per second).
MR angiograms with intra-arterial gadolinium contrast may also be used for MR guidance of endovascular procedures ( Fig. 3 ). Similar to DSA, contrast-enhanced MR angiography can be overlaid onto subsequent image acquisitions for vascular road-map guidance. Although intra-arterial injection of gadolinium chelate is an off-label use, no adverse events have been reported so far. The US Food and Drug Administration total dose limit for gadolinium chelate is 0.3 mmol/L/kg body weight. A low-dose gadolinium injection protocol requires taking into account the blood flow rate in the artery of interest and avoids excess concentrations of gadolinium (resulting in T2/T2* spin-dephasing effects) or inadequate gadolinium concentrations (resulting in lack of T1 shortening). Because contrast delivery is local rather than systemic, much lower doses are needed, and thus, repeat intra-arterial contrast administration is practical. Gadolinium contrast dose limit issues may be at least partially ameliorated by using new gadolinium-based contrast agents that remain in the blood pool for longer periods and provide prolonged vascular enhancement (eg, Gadomer-17, Vasovist).
Different MR imaging pulse sequences may be interleaved and swapped as the procedure demands as the ideal pulse sequence for device visualization or tracking often differs from that needed for vascular road mapping, 2- and 3-dimensional visualization of tissue near the device, or therapeutic monitoring. Endovascular interventional MR imaging requires real-time changes in scan plane and pulse sequence parameters, and MR imaging vendors and third-party providers have started to develop interactive display and MR imaging control software that allow adaptation of parameters (eg, slice orientation, slab thickness, field of view (FOV), or contrast) ( Fig. 4 ).
Tracking of endovascular devices
Accurate, fast, and reliable visualization and localization of endovascular devices are essential requirements for safe and successful endovascular procedures. In DSA, catheters, guidewires, stents, clips, and pacemaker leads are visible because of the increased x-ray absorption of high-atomic-number metal components and markers relative to blood and tissues. The strong magnetic field in the MR imaging environment, however, restricts the use of ferromagnetic metals commonly used in the manufacture of guidewires and stents and in the braiding incorporated within catheter walls for improved torquability and pushability. In addition, the plastic polymers commonly used in catheter construction are difficult to visualize on MR imaging. The increasing availability and clinical utility of MR imaging scanners have created a significant demand for MR-compatible implants and devices such as titanium aneurysm clips and MR-compatible pacemakers. MR-compatible devices for endovascular interventional procedures, in contrast, are lacking because of a lower demand, high costs, paucity of MR imaging scanners dedicated to image-guided procedures, relative familiarity with alternate modalities for image guidance of procedures, and lack of training in MR-guided intervention. Nevertheless, several investigators have developed methods for endovascular device localization and visualization, or tracking, in the interventional MR imaging environment. These methods of device tracking are divided into 2 main categories: active and passive.
Passive tracking methods
With passive tracking, endovascular devices can be tracked on MR imaging by incorporating markers or materials in their construction that enhance, reduce, or distort the T1- and/or T2-weighted MR signal relative to blood or tissues, either focally using a marker at the tip or along the length of the device. Passive tracking methods are summarized in Table 2 .
Passive Tracking Mechanism | Technology | Advantages | Disadvantages |
---|---|---|---|
Negative contrast | Markers with susceptibility artifact (material creating signal void) |
|
|
Positive contrast | Markers with T1 shortening (eg, gadolinium) |
|
|
Nonproton multispectral | Catheters filled with 19 F or 13 C | High CNR and/or SNR |
|
Direct current | Current applied to wire and/or coils create a local field distortion/signal void (negative contrast) |
|
|
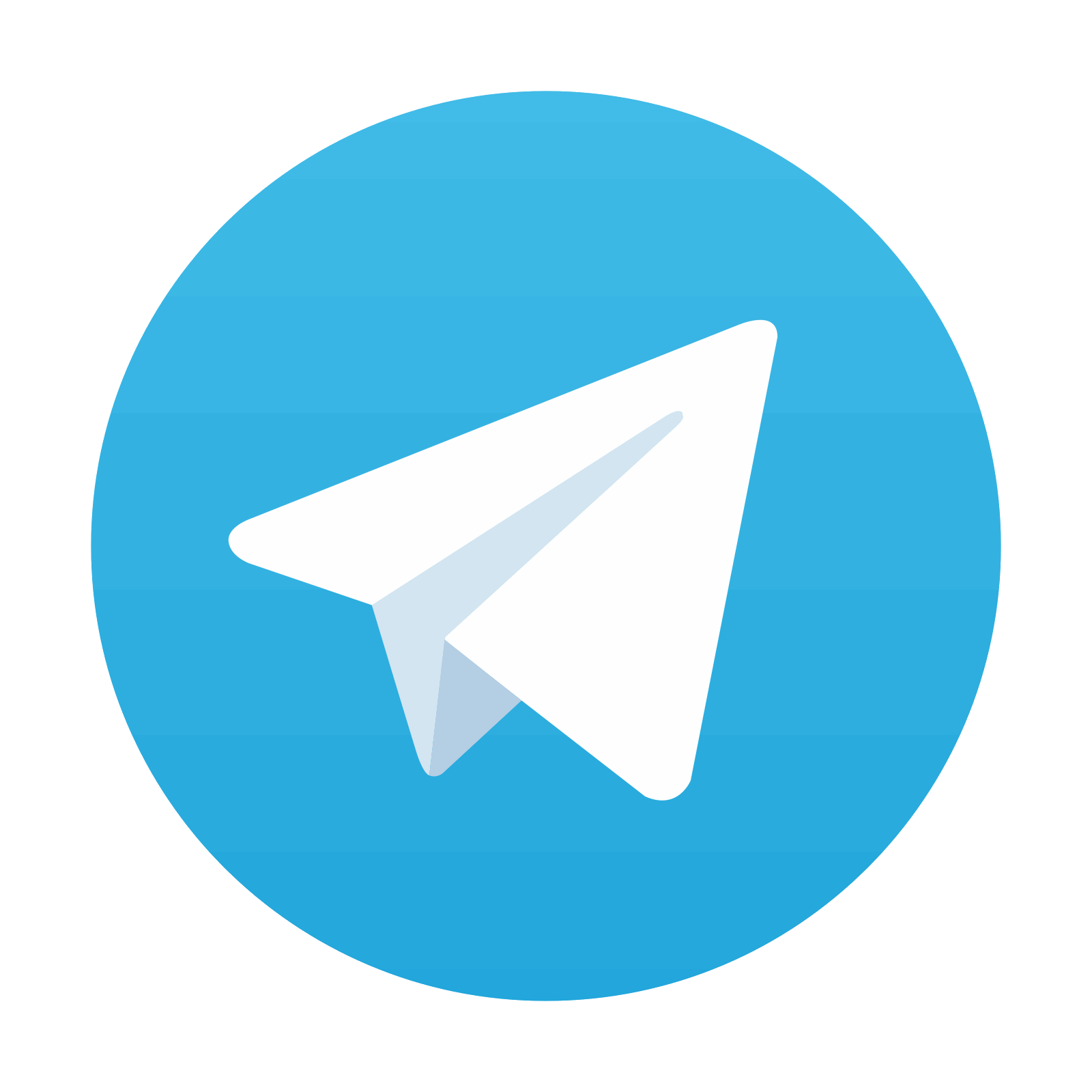
Stay updated, free articles. Join our Telegram channel

Full access? Get Clinical Tree
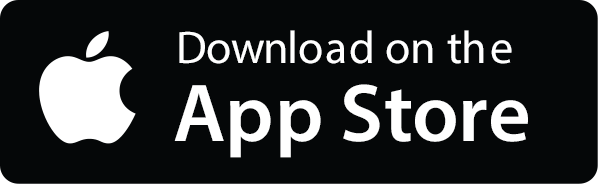
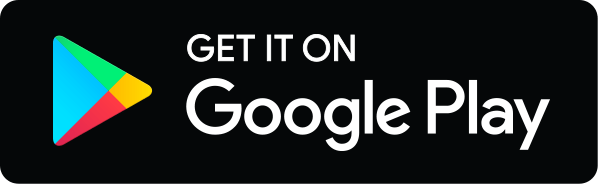
