MR angiography is a powerful tool in evaluating anatomy and pathology when applied to the male pelvis. MR angiography produces high-quality images of the arterial system approaching the resolution of CT angiography, without ionizing radiation. Additional advantages include the ability to obtain angiographic images in the absence of contrast material with non–contrast-enhanced MR angiographic techniques. Blood pool contrast agents, such as gadofosveset, have significantly improved the quality of venous system imaging. Steady state imaging with blood pool contrast agents allows for acquisition of superior-quality high-resolution images and other time-intensive techniques.
Key points
- •
Magnetic resonance (MR) angiography is a powerful tool in evaluating anatomy and pathology when applied to the male pelvis. MR angiography produces high-quality images of the arterial system approaching the resolution of CT angiography, without ionizing radiation.
- •
Additional advantages include the ability to obtain angiographic images in the absence of contrast material with non–contrast-enhanced MR angiographic techniques, which may be necessary in patients with renal disease or with allergy.
- •
The recent introduction of blood pool contrast agents, such as gadofosveset, has significantly improved the quality of imaging of the venous system, because it is no longer dependent on the first-pass imaging. Steady state imaging with blood pool contrast agents allows for the acquisition of superior-quality high-resolution images and other time-intensive techniques.
- •
The extended imaging time also allows for the testing for functional consequences of provocative maneuvers.
Introduction
The male pelvis is complex anatomically and contains many structures unique to the male anatomy, including the penis, scrotum, testicles, seminal vesicles, and prostate gland, as well as structures present in all humans, such as the bladder and rectum. These structures are dependent on a complex network of blood vessels to both supply the organs with blood and return the blood to the circulatory system. Perturbations of the vascular network can lead to diminished function of the male pelvic organs, including erectile dysfunction and diminished fertility. In extreme cases, disruption of blood flow can lead to catastrophic consequences, such as tissue loss and necrosis, as in testicular torsion or ischemic priapism. Examination of the male pelvic vasculature thus may provide valuable insight into male pelvic pathology as well as help with surgical or endovascular treatment planning. This article explores the use of MR angiography in the examination of the male pelvis.
MR angiography is a noninvasive technique, much like CT angiography, that can provide detailed characterization of the male pelvic vasculature. The main advantage of MR angiography is the lack of ionizing radiation, which means it can be performed in younger patients and repeated examinations can be done without concern for the deleterious consequences associated with ionizing radiation. Several additional advantages include the ability to perform noncontrast imaging, which can provide information on velocity and direction of blood flow and can be performed in patients with iodinated contrast allergy and those with kidney disease. In addition, the recent introduction of blood pool contrast media allows for steady state imaging, which can be used for provocative maneuvers as well as high-resolution image acquisition.
Introduction
The male pelvis is complex anatomically and contains many structures unique to the male anatomy, including the penis, scrotum, testicles, seminal vesicles, and prostate gland, as well as structures present in all humans, such as the bladder and rectum. These structures are dependent on a complex network of blood vessels to both supply the organs with blood and return the blood to the circulatory system. Perturbations of the vascular network can lead to diminished function of the male pelvic organs, including erectile dysfunction and diminished fertility. In extreme cases, disruption of blood flow can lead to catastrophic consequences, such as tissue loss and necrosis, as in testicular torsion or ischemic priapism. Examination of the male pelvic vasculature thus may provide valuable insight into male pelvic pathology as well as help with surgical or endovascular treatment planning. This article explores the use of MR angiography in the examination of the male pelvis.
MR angiography is a noninvasive technique, much like CT angiography, that can provide detailed characterization of the male pelvic vasculature. The main advantage of MR angiography is the lack of ionizing radiation, which means it can be performed in younger patients and repeated examinations can be done without concern for the deleterious consequences associated with ionizing radiation. Several additional advantages include the ability to perform noncontrast imaging, which can provide information on velocity and direction of blood flow and can be performed in patients with iodinated contrast allergy and those with kidney disease. In addition, the recent introduction of blood pool contrast media allows for steady state imaging, which can be used for provocative maneuvers as well as high-resolution image acquisition.
Non–contrast-enhanced MR angiography
Non–contrast-enhanced MR angiography, unlike conventional x-ray angiography and CT angiography, does not require intravenous contrast administration. Instead, unenhanced MR angiography takes advantage of the physiologic flow of blood to construct images based on flow-induced signal variations to characterize the lumen of blood vessels. Unenhanced MR techniques create images based on 2 basic characteristics of blood flow that relate to the signal of flowing blood relative to the stationary spins of static tissue. The first is referred to as amplitude effects, where the blood flowing into a chosen slice has a different longitudinal magnetization from the stationary tissue in the given slice. The signal intensity is dependent on the duration of the blood in the slice. The other characteristic is phase effects, which refers to the changes in transverse magnetization that occur as blood flows along the magnetic field gradient compared with the stationary spins of static tissue.
Time of Flight
Amplitude effects are the basis of time-of-flight (TOF) imaging. A given slice (2-D) or slab (3-D) is selected and the stationary tissue is saturated using gradient-echo sequences with very short repetition times reducing the signal from the stationary tissue. Flowing blood, in contrast, has not been saturated and thus has high signal relative to the saturated stationary tissue. The amplitude of the signal is related to the duration of the blood in the slice, or TOF. Thus, the signal intensity is related to the velocity of the blood flow, with higher velocity flow yielding higher signal intensity. The angle of the flow through the selected slice also contributes to signal intensity. Flow perpendicular to the slice has the shortest route through the slice leading to increased signal intensity.
As described previously, blood flowing into the selected slice from either direction produces high signal intensity; thus, the acquired images have both arterial and venous signal. The same principle of saturation of tissue can be applied to selectively image either arterial or venous flow. Signal from inflow can be reduced through the use of presaturation bands. Presaturation bands applied upstream to the selected slice saturate arterial inflow, resulting in the selective imaging of venous blood flow. Alternatively, presaturation bands applied downstream to the selected slice nullify signal from the venous blood flow, resulting in the selective imaging of arterial flow.
TOF images can be acquired through the use of either 2-D or 3-D techniques. In the 2-D technique, a stack of sequentially acquired single slices forms the basis of the image. The advantage of the single slice technique is that it permits good saturation of the stationary tissue optimizing the inflow effect, which in turn allows for the imaging of even in slow flow vessels. This method is best for flow perpendicular to the plane, because vessels not running perpendicular or even running parallel are subjected to saturation and signal is lost.
3-D TOF acquires an entire imaging volume simultaneously, usually a slab 30- to 60-mm in thickness. The benefit of 3-D TOF is the high spatial resolution and high signal-to-noise ratio. An additional benefit is that vessels running parallel are better depicted than in the 2-D method. A drawback of 3-D acquisition is the length of time the blood remains in the saturated slab. The extended transit time in the saturated slab may result in decreased blood signal intensity from the repeated radiofrequency pulses, with slow flow at greatest risk for signal loss. This technique, therefore, requires careful selection of slab thickness to optimize for the region of interest. An additional technique, multiple overlapping thin-slab acquisition (MOTSA), was designed to have the advantages of both 2-D and 3-D TOF. MOTSA is less susceptible to the signal loss because of saturation with 3-D TOF due to the thin slabs used and retains the high spatial resolution and signal-to-noise ratio. Reduced susceptibility to signal loss due to thinner acquisition slabs comes at the cost of longer acquisition times.
Phase Contrast
Phase-contrast angiography is based on phase effects to produce angiographic images. A bipolar gradient is applied in gradient-echo acquisitions. Stationary tissue is dephased and rephased to its original state, whereas moving tissue dephases in proportion to the flow velocity. Because the encoding gradients are defined to encode flows within a certain velocity range, the operator must determine the anticipated velocity to choose the appropriate encoding gradient because velocities outside of the range are poorly encoded.
Phase-contrast acquisitions allow for the detection of flow in any plane in 3-D space. This is accomplished by repeating the encoding in the X, Y, and Z axes. The images acquired from the 3 axes are then summed and subtracted from reference images performed without encoding gradient, leaving only the images of vessels. In addition, flow velocity can be determined noninvasively with phase-contrast imaging when the slice acquisition is perpendicular to the direction of blood flow. Flow rate can then be calculated from the product of the vessel area and flow velocity.
Contrast-enhanced MR angiography
Gadolinium-Based Contrast Agent
Classically, contrast-enhanced MR angiography has been performed with extracellular contrast agents, such as gadobenate dimeglumine (MultiHance [Bracco Diagnostics, Monroe Township, NJ, USA]) or gadopentetate dimeglumine (Magnevist [Bayer Health Care, Whippany, NJ, USA]). Extracellular contrast agents have a short blood pool half-life and rapidly distribute into the extravascular space, thus only allowing for first-pass images, making the timing of the bolus injection relative to imaging sequence exceedingly important, with little margin for error. The rapid extravasation of contrast into the extravascular space also limits the ability to obtain high-resolution images due to the length of time required for imaging.
The limitations of imaging with extracellular agents led to the development of contrast agents with a prolonged intravascular half-life, referred to as blood pool contrast agents. Gadofosveset (Ablavar, Lantheus Medical Imaging, Inc, North Billerica, MA, USA), previously known as Vasovist and MS-325, is the first blood pool contrast agent approved for MR angiography by the Food and Drug Administration (FDA), in December 2008, and by the European Union, in 2005. Gadofosveset is a linear ionic gadolinium chelate, which reversibly binds to human serum albumin, which effectively prolongs the serum half-life by tethering the molecule in the intravascular space and protecting it from glomerular filtration.
Prior to its approval by the FDA, gadofosveset underwent extensive clinical testing. Clinical testing included dose-escalation studies, blinded placebo-controlled studies, and 4 phase III studies. Shamsi and colleagues provides a review of the safety of gadofosveset in 767 patients evaluated in phase II and phase III studies. They found that the safety profile was comparable with that of other gadolinium agents. The 3 most common adverse effects reported by the patients were pruritis (5.0%), headache (4.3%), and nausea (4.2%). Four (0.5%) of 767 patients reported 5 serious adverse events. The serious adverse events reported were chest pain, gangrene, anaphylactoid reaction, and, in one patient, hypoglycemia and aggravated coronary artery disease. Gangrene and chest pain were thought unrelated to gadofosveset. The anaphylactoid reaction resulted in itching and heavy soreness in the patient’s perineal region immediately after bolus injection, which resolved within 5 minutes after intravenous administration of antihistamine, and the patient continued with the MR examination.
The T1 relaxivity of gadofosveset is greater than the extracellular contrast agents in routine use. For example, gadofosveset has a T1 relaxivity in blood at 1.5 T, approximately 5-fold that of gadopentetate. The relative increased T1 relaxivity compared with other agents allows for a lower dose of contrast (0.03 mmol/kg) versus the 0.1 mmol/kg for most gadolinium-based extracellular contrast agents.
Like other bolus-injectable contrast media, first-pass MR angiography is easily performed with gadofosveset. The principal advantage of gadofosveset is the ability to perform steady state imaging, providing the opportunity to acquire images for approximately 45 to 60 minutes after a single bolus injection. This allows for the acquisition of time-intensive acquisitions, such as high-resolution images, cardiac-gated imaging, and imaging with provocative maneuvers. In addition, the extended period available for imaging allows for the imaging of vasculature at multiple anatomic sites.
Ultrasmall Paramagnetic Iron Oxide Particles
An alternative to gadolinium-based blood pool contrast agents is the use of ultrasmall paramagnetic iron oxide particles (USPIOs). USPIOs were initially developed by size fractionation of superparamagnetic iron oxide particles with gel chromatography, resulting in 70% of the particles smaller than 10 nm in size. The reduced particle size significantly increased the blood pool half-life as well as enabling the particles to cross the capillary endothelium into the interstitial space where particles ultimately accumulate in lymph nodes. The USPIOs have been extensively studied in lymphotropic nanoparticle MR imaging as a method to characterize lymph nodes as either benign or malignant in cancer patients and have demonstrated a good safety profile. The T1-shortening property of the nanoparticles along with the prolonged blood pool half-life allows for the mapping of the vasculature using T1-weighted gradient-recalled echo sequences. In 2009, ferumoxytol, a third-generation bolus-injectable USPIO, was approved by the FDA for the treatment of iron-deficiency anemia in adult patients with chronic kidney disease. Ferumoxytol has a 10- to 14-hour blood pool half-life and is bolus injectable, allowing for first-pass as well as equilibrium-phase MR angiography. Although ferumoxytol has not been specifically approved for use in MR angiography, its safety profile in patients with renal disease suggests that in patients with chronic kidney disease ferumoxytol may be a suitable substitute for gadolinium-based contrast agents. Sigovan and colleagues compared TOF MR angiography with ferumoxytol-enhanced MR angiography and found consistently superior image quality with the USPIO-based blood pool agent relative to TOF.
Arterial system
Anatomy
Understanding pelvic artery anatomy and its variations is important in the interpretation of pelvic MR angiography because the arterial anatomy may have important clinical implications as well as providing guidance in preprocedural planning either for surgery or endovascular approach. The main arterial supply to the pelvic organs and lower extremities originates at approximately the L4 level with the bifurcation of the aorta into the right and left common iliac arteries. The common iliac artery measures, on average, approximately 1.23 cm in men and 1.02 cm in women and courses inferolaterally along the medial border of the psoas muscle. The common iliac artery gives rise to the external and internal iliac arteries at the pelvic brim ( Fig. 1 ).
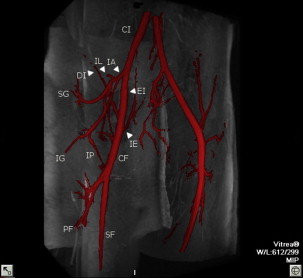
External iliac artery
The external iliac artery provides the arterial supply to the lower extremity and to the tissues of the lower abdominal wall. The course of the external iliac artery continues along the medial border of the psoas muscle and as it exits the pelvis at the inguinal ligament the artery continues as the common femoral artery providing the blood supply to the lower extremity. The deep circumflex iliac artery and the inferior epigastric artery are branches of the external iliac artery that feed the muscles and skin of the lower abdomen. The deep circumflex iliac artery arises laterally from the external iliac artery and follows the course of the iliac crest of the pelvis, whereas the inferior epigastric artery branches medially just superior to the inguinal ligament and ascends superiorly in the abdominal wall (see Fig. 1 ).
Internal iliac artery
Embryologically, the internal iliac artery, sometimes still referred to as the hypogastric artery, is derived from the umbilical artery. Postnatally, after the cessation of placental circulation, the distal segment of the umbilical artery becomes the medial umbilical ligament and the proximal segment persists as the internal iliac artery and superior vesical artery. The internal iliac artery is the predominant arterial supply to the pelvic organs and musculature of the pelvis and is subject to significant anatomic variation. Conceptually and anatomically, the branching pattern of the internal iliac artery can be divided into the anterior and posterior divisions. The posterior division supplies the musculature and osseous structures of the pelvis through the superior gluteal artery, iliolumbar artery, and lateral sacral arteries. The anterior division provides blood supply to the pelvic organs, such as the bladder and rectum, as well as the prostate, seminal vesicles, ejaculatory ducts, and penis in men (see Fig. 1 ).
Several classification schemas have been developed for the categorization of the internal iliac artery variant anatomy. The Yamaki classification system described in 1998 simplifies internal iliac artery classification based on the branching patterns of the superior gluteal, inferior gluteal, and internal pudendal arteries. Yamaki initially evaluated the Adachi classification in 645 pelvic halves in Japanese cadavers and found some branching patterns different from that described in the Adachi classification system. The Yamaki classification was thus developed to simplify the classification of the internal iliac artery into 4 groups of anatomic variants. Schematic representations of the 4 groups are shown in Fig. 2 . In group A (80% of cadaveric study), the superior gluteal artery arises independently whereas the inferior gluteal and internal pudendal arteries arise from a common trunk. Group B (15% cadaveric study) has a common posterior gluteal trunk from which the superior and inferior gluteal arteries arise with an independent origin of the internal pudendal artery. Independent origins of the superior gluteal, inferior gluteal, and internal pudendal arteries characterize group C, noted in 5.3% of the cadavers. Finally, in group D, only noted in 1 of 645 pelvic halves, the superior gluteal and internal pudendal arteries have a common origin and the inferior gluteal artery arises independently.
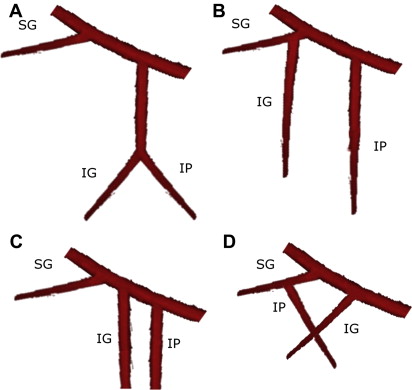
The superior gluteal artery is the largest branch of the internal iliac artery and supplies the gluteal muscles—maximus, medius, and minimus. It exits the pelvis at the superior aspect of the great sacrosciatic foramen above the piriformis muscle. The second largest branch of the internal iliac artery is the inferior gluteal artery, which provides blood supply to the gluteus maximus, piriformis, and quadratus femorus muscles and exits at the inferior aspect of the greater sacrosciatic foramen inferior to the piriformis muscle. The internal pudendal artery tends to be the third largest vessel of the internal iliac artery. The course of the internal pudendal artery is similar to that of the inferior gluteal artery as it exits the inferior aspect of the great sacrosciatic foramen, but it then curves around the sacrospinous ligament and re-enters the pelvis through the lesser sacrosciatic foramen. In the pelvis, the internal pudendal artery branches into the inferior rectal artery, perineal artery, and posterior scrotal branches and terminates as the common penile artery.
The obturator artery is an additional branch of the internal iliac artery but is subject to considerable anatomic variation. In approximately two-thirds of cases, the obturator artery arises from the internal iliac artery and extends to and exits the obturator foramen and divides into the anterior and posterior branches in a distinctive 90° angle. In the other one-third of cases, the obturator artery is a branch of the inferior epigastric artery from the external iliac artery, a variant sometimes referred to as the corona mortis , translated as the crown of death due to its susceptibility to traumatic injury. Additional branches of the internal iliac artery that arise from the anterior division include the superior vesical, inferior vesical, and middle rectal arteries.
Peripheral Vascular Disease
The noninvasive characterization of the patency of peripheral vasculature is an important application of MR angiography, with implications in the diagnosis of peripheral vascular disease as well as the appropriate selection of therapy. A prospective study was performed that compared both first-pass MR angiography and steady state MR angiography with gadofosveset in 334 arterial segments in 27 patients with digital subtraction angiography (DSA). First-pass MR angiography underestimated stenosis in 4.5% of the arterial segments and overestimated stenosis in 8% of the segments. High-resolution steady state imaging, however, was in agreement with DSA findings in 100% of the 334 arterial segments. The improved accuracy was attributed to the high-resolution sequences that reduced volume averaging artifact only possible in steady state imaging. A maximum intensity projection (MIP) image from first-pass MR angiography is shown in comparison with a DSA image in Fig. 3 .
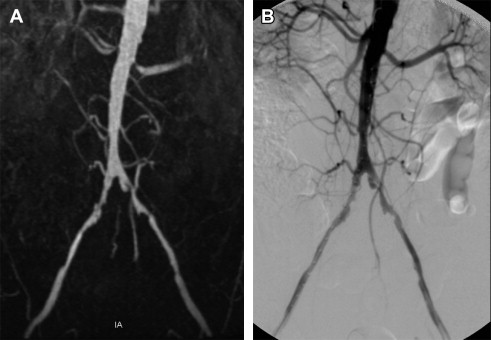
Iliac Artery Aneurysms
MR angiography is useful not only for characterizing the complex vascular anatomy of the male pelvis but also for evaluating the vessels for aneurysm, dissection, stenosis, and occlusion. Isolated or solitary aneurysms of the iliac arteries have a strong male predominance with an age-related increase in incidence. Aneurysm is derived from the Greek roots, ana (upon) and eurys (broad). In practical terms, aneurysm is often defined as the permanent focal dilation of the artery having at least a 1.5-times increase in diameter compared with the normal artery. In the context of the common iliac artery, aneurysm is defined by the Society for Vascular Surgery reporting standards as any permanent focal dilation of the iliac artery greater than 1.5 cm in diameter.
Iliac artery aneurysms are seen in approximately 10% to 20% of patients with abdominal aortic aneurysms, whereas isolated iliac artery aneurysms are rare, accounting for less than 2% of all aneurysmal disease. Iliac artery aneurysms are important to consider and recognize due to the risk of rupture and high mortality associated with rupture. As many as 33% of patients with iliac artery aneurysms present with rupture. A majority of patients with isolated iliac artery aneurysms, however, are either asymptomatic or have nonspecific symptoms. Nonspecific symptoms are often secondary to mass effect or compressive symptoms from the aneurysm, including lumbosacral pain, tenesmus, or constipation and urinary obstruction. Additional symptoms may include vascular symptoms, such as intermittent claudication, lower extremity pain from arterial occlusion, deep venous thrombosis (DVT) from iliac vein compression, or even high-output cardiac failure from arteriovenous fistula (AVF) from the erosion of the aneurysm into the iliac vein. Thus, most iliac artery aneurysms are found incidentally either during surgery on diagnostic imaging studies. Those patients presenting with rupture may have abrupt onset of abdominal, groin, or thigh pain and hypotensive shock.
As discussed previously, there is a strong male predilection for isolated iliac artery aneurysms, with an estimated male-to-female ratio of 7:1. Moreover, the incidence increases significantly with age, as evidenced by a 6.5-fold increase in incidence in patients 75 years and older compared with 55-year-old patients. Thus, a typical patient with isolated iliac artery aneurysm is an elderly man in his 7th or 8th decade of life. Surveillance MR angiography from an 83-year-old man with incidentally discovered isolated right common iliac artery aneurysm is shown in Figs. 4 and 5 . A vast majority of iliac artery aneurysms are secondary to degenerative changes resultant of atherosclerotic disease. Additional causes include para-anastomotic graft failure, penetrating injury from trauma, and iatrogenic causes related to surgery. Other reported causes include mycotic/infectious, vasculitis, and inherited disorders of connective tissue, including Marfan syndrome and Ehler-Danlos syndrome.
