Tumor characteristics and presentation
Low-grade glioma–surgical management and considerations
High-grade glioma–surgical management and considerations
Large tumors, significant neurologic deficit, and/or medically intractable seizures
Early surgical debulking with extensive resection while minimizing neurologic dysfunction
Extensive resection which minimizes neurologic dysfunction
Decompression of tumor provides symptomatic relief and reduces tumor burden
Gross total resection improves patient overall survival
Decreases risk for malignant transformation (Level III evidence)
(Level III evidence)
Use of image guided resection to achieve more extensive resection (Level I evidence)
Decompression of tumor provides symptomatic relief and reduces tumor burden
Small tumors without mass effect found on MRI, transient neurologic symptoms
Consider watchful waiting and delayed intervention
Extensive resection which minimizes neurologic dysfunction
Monitor for radiologic progression
Gross total resection improves patient overall survival
Monitor for clinical progression of neurologic symptoms
(Level III evidence)
Use of image-guided resection to achieve more extensive resection (Level I evidence)
Decompression of tumor provides symptomatic relief and reduces tumor burden
Tumors located in motor, sensory, or language functional areas and/or deep seated tumors
Stereotactic biopsy only
Extensive resection which minimizes neurologic dysfunction
Monitor for radiologic progression
Gross total resection improves patient overall survival
Monitor for clinical progression of neurologic symptoms
(Level III evidence)
Consider resection
Intraoperative cortical mapping to identify functionally critical areas (Level III evidence)
Intraoperative cortical mapping to identify functionally critical areas (Level III evidence)
Use of image-guided resection to achieve more extensive resection (Level I evidence)
Consider resection in conjunction with stereotactic brachytherapy
(Level III evidence)
Role of Surgery for Patients with Low-Grade Gliomas
Patients with low-grade gliomas often have a better prognosis than patients harboring higher-grade tumors [3–10, 12, 18–21]. In fact, the median survival for patients with low-grade gliomas is typically between 5 and 10 years [3–10, 12, 18–21]. Despite this more favorable prognosis, 50–75 % of patients with low-grade gliomas eventually die from their disease because of either tumor progression or degeneration to a higher malignant grade [1, 7, 8]. The optimal treatment for patients who harbor low-grade gliomas, however, remains unclear because many patients are followed radiographically and intervention is only pursued when clinical or radiographic progression becomes evident [35]. Moreover, the role of surgery in delaying recurrence and/or malignant degeneration as well as prolonging survival remains controversial [7, 35].
This lack of understanding of the effectiveness of surgery for patients with low-grade gliomas is because there are no randomized control trials or matched pair analyses. Keles et al. performed a literature review of the role of surgery for patients with low-grade gliomas and found 30 studies evaluating the role of surgery for patients with low-grade gliomas [1]. In this review, all but five studies were excluded because they included patients with grade I gliomas, relied upon intraoperative determination of extent of resection, and had small sample sizes, among others [36–39]. These five studies that met the inclusion criteria were also limited because extent of resection was determined by intraoperative gross visualization rather than neuro-imaging, and a distinction between gross total and near total resection was not made [36–39]. This review and others have found data supporting a role of surgery in delaying recurrence and prolonging survival controversial [1, 40].
Despite this, there are an increasing number of studies demonstrating a benefit for increasing extent of resection for patients with low-grade gliomas [7]. Wisoff et al. evaluated 518 pediatric patients with low-grade glioma, and found that extent of resection was significantly associated with improved progression-free survival [41]. The 5-year survival rate was 99 % with gross total resection, 95 % with 1.5 cm3 residual disease, 94 % with 1.5–2.9 cm3 residual, and 87 % with >3 cm3 residual [41]. The 5-year progression-free survival rate was 90 % with gross total resection and 45–65 % with any volume (1.5–3 cm3) of residual disease [41]. More recently, Smith et al. performed a retrospective volumetric analysis of extent of hemispheric LGG resection in 216 patients and found that patients with at least 90 % resection had 5- and 8-year survival rates of 97 % and 91 %, respectively, while patients with less than 90 % resection had 5- and 8-year survival rates of 76 % and 60 %, respectively [42]. We recently evaluated 170 patients with low-grade gliomas and found that gross-total resection was associated with increased overall survival as compared to patients who underwent subtotal resection [11]. Five-year overall survival after gross total, near total, and subtotal resection was 95 %, 80 %, 70 %, respectively, and 10-year survival was 76 %, 57 %, and 49 %, respectively [11]. Additionally, after gross total, near total, and subtotal resection, median time to tumor progression was 7.0, 4.0, and 3.5 years, respectively [11]. The median time to malignant degeneration after gross total, near total, and subtotal resection was 12.5, 5.8, and 7 years, respectively [11]. In a separate study, we found that gross total resection was independently associated with delayed malignant degeneration [7].
There is a growing body of evidence that surgery is an effective treatment for patients with low-grade gliomas, despite an absence of randomized control trials. For patients who present with large tumors, significant neurological deficits, and medically intractable seizures, immediate surgical intervention is generally the preferred treatment option [7, 43]. Extensive resection without causing an iatrogenic deficit can lead to improvements in overall survival, progression-free survival, and malignant transformation-free survival [7–9, 44]. However, for incidentally discovered small tumors without mass effect or symptoms, observation with surveillance imaging is a viable option given the slow growth and natural history of low-grade gliomas (Table 18.1) [45].
Intra-Cavitary Radiopharmaceutical Therapy or Stereotactic Brachytherapy
The standard of care for patients with high-grade gliomas is extensive resection, followed by radiation and temozolomide chemotherapy [46]. Regardless of treatment, tumor recurrence typically occurs close to the tumor margins [20]. At the tumor margin, there is an increased tumor cell density [20]. Beyond the periphery, there is a sharp fall-off in cell numbers as the distance from the resection cavity increases [20]. Extensive surgical resection of gliomas can prolong progression-free and overall survival by decreasing tumor burden and possibly increasing adjuvant therapy efficacy as it has with other types of solid tumors [47]. Moreover, the use of adjuvant therapies have been developed to help reduce the tumor burden at the tumor periphery. One of these developments is SBT (Table 18.2).
Table 18.2
Summary of benefits and risks of stereotactic brachytherapy use in gliomas
Stereotactic brachytherapy benefits | Stereotactic brachytherapy risks |
---|---|
Minimizes surgical intervention in functionally critical tissue | Prospective, randomized controlled trials have failed to demonstrate a survival advantage |
Avoids systemic toxicity of chemotherapy | Invasive procedure when compared to external beam radiation therapy |
Avoids neuro-cognitive effects in children who undergo EBRT | Limited to tumors <5 cm in diameter and spherical in shape |
Retrospective series demonstrate both safety and efficacy | Complications: Radiation-induced necrosis and neurological dysfunction |
It is generally considered beneficial to provide radiation to the gliomatous areas around the surgical cavity, especially for high-grade gliomas [14, 48, 49]. The techniques to deliver this radiation include stereotactic radiation therapy and SBT [14, 48, 49]. SBT involves the delivery of radiation by stereotactically implanting radioactive sources near or within tumors [14, 48, 49]. SBT can be applied peri- or postoperatively by either permanent isotope seeds or by temporary catheters containing radioisotopes [14, 48, 49]. There are a variety of different isotopes that can be administered for radiation therapy including Iodine-125, Iodine-131, and Iridium-192, among others [14, 48, 49]. The exposure times of these seeds range from a few days to several months and vary whether temporary implants or permanent seeds are used [14, 48, 49]. In the central nervous system, the most common radioactive source used for SBT are Iodine-125 seeds (125I) which emit low-energy radiation of 0.028–0.035 meV and have a steep falloff dose that reduces peripheral normal tissue exposure [14, 15].
SBT has been used as adjuvant treatment following surgical resection for both primary and recurrent low- and high-grade gliomas and has been used for tumors in eloquent regions that are considered unresectable [14, 48, 49]. The theoretical benefit of SBT is that it allows for precise surgical implantation of radioisotopes within the tumor or tumor cavity, thus providing local radiation to adjacent tissue while sparing surrounding tissue [15]. Additionally, SBT avoids the systemic side effects of chemotherapy and the potential neuro-cognitive effects of external beam radiation therapy (EBRT) [50]. However, while numerous retrospective studies have demonstrated SBT to be safe and efficacious in gliomas [15, 51–56], three small single institution prospective randomized trials failed to demonstrate a survival advantage [57–59]. Therefore, the efficacy of this treatment option requires further investigation as well as potential advances in imaging and implant technology before it will be widely used.
Procedure
SBT is based on the principle that radiation will induce necrosis in a targeted tumor volume and that necrotic tissue will then be removed by macrophages [60]. Stereotactic computed tomography and magnetic resonance images (MRI) are co-registered for treatment planning and dosimetry calculations [61]. The typical cumulative, prescribed dose ranges from 50–65 Gy over 3–5 days [14, 48, 49]. In interstitial radiotherapy, 125I seeds are administered via stereotactically implanted catheters intraoperatively [15, 62]. Dose rates vary and individual institutions vary over whether a low dose, which allows build up of damage in tumor cells, or a high dose rate, which decreases total treatment time, is administered [14]. A potential barrier to the success of radioactive seed brachytherapy may be the inhomogeneous distribution of doses [14, 48, 49]. Tumor areas adjacent to seeds receive a very high dose that may contribute to toxicity, whereas the areas in between seeds may have cold spots and the tumor is potentially under-dosed in these regions [63].
Recently, the advent of GliaSite brachytherapy allows for administration of 125I on an outpatient basis [64–66]. With GliaSite, an expandable balloon catheter can be implanted intracranially near the tumor or within a resection cavity thereby providing a conformal fit (Fig. 18.1) [64–66]. Liquid 125I is then used as the source of radiation and the balloon is inflated through an accessible subcutaneous port; this administration provides the opportunity for treatment to be administered after several weeks of postoperative recovery [66, 67]. As the liquid radioisotope distributes uniformly throughout the balloon, there is a uniform delivery of radiation to the region surrounding the balloon [64–66]. This minimizes the potential for “hot” or “cold” radiation regions and also allows for a predictable distribution of radiation dose, which decreases with distance from the balloon surface [64–66]. Whereas stereotactic implantation of radiation seed catheters can be placed in the midst of actual gross tumor, the GliaSite technique is only useful when a surgical cavity has been created by resection of all or most of the tumor [64–66]. There also continues to be interest in developing targeted delivery of radiation dose by using radioisotopes bound to molecules, which preferentially bind to glioma cells [64–66]. In addition to 125I, I-131 labeled TM-601, a synthetic chlorotoxin, has also been demonstrated to effectively deliver radiation [68]. Efficacy has not been demonstrated, but this general approach remains promising and continues to be a subject of investigation.
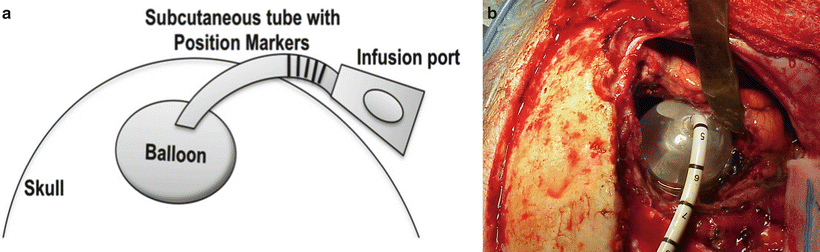
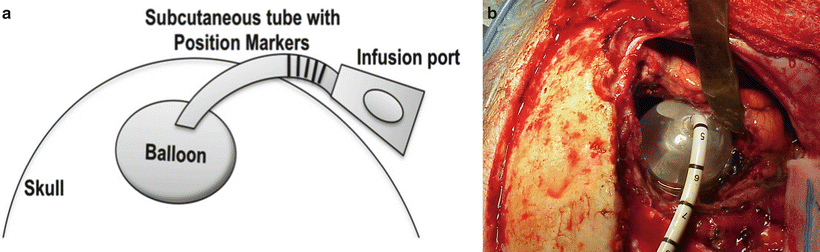
Fig. 18.1
GliaSite radiation therapy system device. (a) Schematic shows device components: balloon, subcutaneous tube, and infusion port. (b) Intraoperative photograph of device implantation after tumor resection
Indications
Therapeutic management of gliomas includes some combination of stereotactic biopsy and radiologic monitoring, surgical resection, and chemo-radiation therapy [3–5, 16]. Although surgical resection is the gold standard, tumors located in eloquent or critical areas of the brain are not always amenable to extensive debulking [49]. In children with inoperable low-grade gliomas, SBT is a treatment option that avoids the hematologic and otologic toxicity of chemotherapy and the cognitive and neuropsychological deficits associated with EBRT [50, 69]. In these children, retrospective studies have found SBT to be safe and have an efficacy that is comparable to conventional chemo-radiation with 5 and 10-year survival rates of 97 % and 92 %, respectively [51, 52, 69]. Additionally, in adults with low-grade gliomas, the combination of SBT and microsurgery reduces the high complication rate of injury to eloquent brain regions while effectively irradiating the tumor in several retrospective studies [56, 70, 71]. For high-grade gliomas, SBT has been utilized for both operated and inoperable gliomas in combination with adjuvant therapies and has been shown to be safely tolerated [55, 72]. Several studies have retrospectively studied the safety and feasibility of SBT in high-grade gliomas using GliaSite in combination with resected brain tumors [66, 67].
Previous Studies
Previous studies (Table 18.3) have demonstrated the safety and efficacy of brachytherapy for patients with gliomas. Gutin et al. studied 63 patients who were administered brachytherapy (125I) in addition to EBRT, procarbazine, lomustine, and vincristine from 1982 to 1990. The median survival for patients with anaplastic gliomas was 157 weeks for those with implants and 165 weeks for those without implants, while the median survival for patients with GB was 88 weeks for those with implants and 67 weeks for those without implants [73]. Wen and colleagues treated 56 patients with GB from 1987 to 1993 [74]. The patients in this study underwent surgery, limited field EBRT, and brachytherapy with 125I [74]. The median survival for patients undergoing brachytherapy was 18 months compared with 11 months for a matched brachytherapy control group with similar clinical and radiologic features [74]. Thirty six patients (64 %), however, underwent reoperation for symptomatic radiation necrosis [74]. Welsh et al. studied 20 patients from eight institutions who underwent Gliasite brachytherapy boost [66]. These patients underwent extensive debulking followed by EBRT [66]. The average survival for this study population was 11.4 months, which was 3 months longer than historical controls [66]. Three patients (14 %) in this series experienced central nervous system toxicity [66].
Table 18.3
Summary of previous studies on the use of brachytherapy
Author | Years | Number of patients | Glioma type | Brachytherapy type | EBRT dose (cGy) | Median survival (months) | Complications |
---|---|---|---|---|---|---|---|
Gobitti et al.a | 2006–2008 | 15 | Recurrent WHO IV | Gliasite | 4,500 | 13 | Radiation necrosis (20 %), death (13 %) |
Suchorska et al. | 1982–2006 | 95 | Recurrent WHO grade II | Iodine-125 | 20 | 3.3 % permanent morbidity | |
Chen et al.a | 1999–2002 | 18 | WHO IV | Iodine-125 | 4,000 | 26.3 | Radiation necrosis (61 %) |
Welsh et al. | 2000–2004 | 20 | WHO IV | Gliasite | 5,000 | 11.4 | Radiation necrosis (14 %) |
Reardon et al. | 1996–2003 | 43 | Recurrent WHO III-IV | Iodine-131 | 4,600 | 14.8 | Radiation necrosis (12 %), hematologic toxicity (23 %) |
Akabani et al. | 1996–2003 | 18 | WHO III-IV | Iodine-131 | 4,800 | 18.2 | Radiation necrosis (39 %) |
Selker et al.b | 1987–1994 | 133 | WHO III-IV | Iodine-125 | 6,000 | 15.7 | Radiation necrosis (15.3 %) |
Koot et al. | 1998–1995 | 66 | WHO IV | Iodine-125 (45), Iridium-192 (21) | 5,000 | 16 | Radiation necrosis (5 %), Vascular ischemia (14 %) |
Johannsen et al. | 1992–1996 | 44 | WHO III-IV
![]() Stay updated, free articles. Join our Telegram channel![]() Full access? Get Clinical Tree![]() ![]() ![]() |