KEY POINTS
Inborn errors of metabolism (IEMs) are rare disorders, most of them only present after delivery.
Several IEMs may affect the fetus, producing congenital malformations and/or brain insults.
Prenatal diagnosis is usually possible following the birth of an affected child; in patients without a family history, even in the presence of severe malformations, a definitive diagnosis is usually only made after delivery.
Biochemical tests and molecular studies are indicated in selected cases.
The in utero metabolic microenvironment during embryogenesis (fetal metabolome) profoundly influences the entire range of developmental processes underlying fetal organogenesis.
Inborn errors of metabolism (IEMs) are inherited disorders with mostly single gene defects resulting in the development of enzymatic blocks within biochemical pathways, often due to the deficiency of an enzyme or cofactor. There may be secondary accumulation or formation of toxic intermediaries, as well as deficiency of critical end products necessary for cell function. The resulting changes influence the internal and external cellular microenvironment, as well as cellular homeostatic mechanisms.1 The association of IEMs with developmental malformations has long been recognized (Figure 12–1). Following initial reports of association of callosal dysgenesis with IEMs,2 widespread developmental abnormalities in the morphogenesis of the brain have been described.3 A logical extension of these observed associations is the exploration of the possibilities of detection and diagnosis during the prenatal period. A majority of these conditions are usually diagnosed in postnatal life in the index case. During subsequent pregnancies, early detection and diagnosis carry the potential for early therapeutic interventions in the fetus or carefully considered decisions to terminate the pregnancy in the event of the diagnosis of an incurable disorder with no hope for a meaningful quality of life.
A variety of interactions between the planes of genome–proteome and metabolome regulate developmental processes that ultimately influence the formation and maturation of all organ systems, including the fetal brain. In early fetal life, interference with formation of the telencephalic vesicles (holoprosencephaly), dysgenesis of the corpus callosum, absence of the septi pellucidi, cerebellar dysgenesis, and abnormalities in ventricular shape (colpocephaly and single ventricle) may be visualized through targeted neurosonography. As the brain grows in complexity, abnormalities may extend to involve the gray matter (atrophy of the cortical ribbon and basal ganglia), white matter (thinning out or loss of volume, demyelination, or dysmyelination of white matter), encephaloclastic lesions (porencephalic cysts), and neuronal migration defects (pachygyria) and can be identified with the aid of high-resolution fetal magnetic resonance imaging (MRI). Neuronal loss or cell death (neurotoxic or apoptotic) is followed by wallerian (secondary axonal) degeneration, leading to atrophy and volume loss in the gray and white matter. These changes result in ventriculomegaly and a prominence of the extra-axial fluid spaces. Porencephalic cysts are seen secondary to ischemic injury following vascular occlusion and focal neuronal necrosis. Secondary processes such as failure or interference with myelination by glial cells will result in demyelination or dysmyelination.4
IEMs involve different biochemical/metabolic pathways, substrates, intermediary compounds, and end products. There is a large body of literature usually in the form of case reports linking individual disorders to the malformations of the nervous system during embryogenesis. For instance, disorders involving folic acid metabolism and folate deficiency states result in neural tube defects, and defects in the glycine cleavage pathway have a well-documented association with agenesis of the corpus callosum. Defects in cholesterol metabolism interfere with the development of telencephalic vesicles and appear to lead to holoprosencephaly and its variants. The cerebellum appears to be peculiarly vulnerable to the effects of metabolic perturbations. Defects of cerebellar development are associated with a variety of IEMs, such as congenital lactic acidosis, mitochondrial disorders, and disorders of glycosylation.3
Current technological advances in fetal ultrasonography and MRI permit visualization of the fetal brain in considerably greater detail than previously possible and enable detection of developmental malformations. Furthermore, these techniques permit a noninvasive tool to monitor serial changes over time. Recognition of specific patterns and associations with inborn errors of metabolism guide the neurologist and the metabolic specialist in targeting appropriate investigations, critical for diagnosis, treatment, and counseling.
When dealing with rare and infrequently diagnosed diseases, fetal imaging has two different goals. In families at risk, the examination should be conducted with the goal of identification of specific, predefined patterns; the examiner should be familiar with these patterns prior to the evaluation in order to target the examination accurately (Tables 12–1 and 12–2). When technical problems impair full visualization, it should be included in the report and a follow-up examination scheduled.
Inborn Error of Metabolism | Neural Tube Defects | Holoprosencephaly | Cerebellar Malformations | Hypoplastic Temporal Lobes |
---|---|---|---|---|
Energy metabolism | ||||
Respiratory chain enzyme deficiency | + | |||
Fatty acid oxidation | ||||
Glutaric acidemia 2 | + | + | ||
Folic acid metabolism | ||||
Methylenetetrahydrofolate reductase deficiency | + | |||
Organic aciduria | ||||
Glutaric aciduria 1 | + | |||
Ethylmalonic aciduria | + | |||
Cholesterol metabolism | ||||
Smith-Lemli-Opitz | + | |||
Glycoprotein metabolism | ||||
Congenital disorder of glycosylation type 1a | + | |||
Trace element metabolism | ||||
Menkes kinky hair | + |
Inborn Error of Metabolism | Pachygyria | Polymicrogyria | Cortical Heterotopia | Cerebellar Dysplasia | Olivary Nuclei Dysplasia | Dysgenetic Corpus Callosum |
---|---|---|---|---|---|---|
Peroxisomal disorders | ||||||
Zellweger | + | + | + | + | + | + |
Infantile Refsum | + | + | ||||
Pseudoneonatal adrenoleukodystrophy | + | + | + | |||
Bifunctional enzyme deficiency | + | + | + | + | + | |
Chondrodysplasia punctata | + | |||||
Energy metabolism | ||||||
Pyruvate dehydrogenase deficiency | + | + | + | + | + | |
Fumarase deficiency | + | + | + | + | ||
Fatty acid oxidation defects | ||||||
Carnitine palmitoyl transferase 2 | + | |||||
Glutaric acidemia 2 | + | + | + | |||
Aminoacidurias | ||||||
Maternal phenylketonuria | + | |||||
Nonketotic hyperglycinemia | + | + | ||||
Organic aciduria | ||||||
3-Hydroxyisobutyric aciduria | + | + | ||||
Cholesterol metabolism | ||||||
Smith-Lemli-Opitz | + | + | + | + | + | |
Trace elements | ||||||
Menkes kinky hair syndrome | + | + | + | |||
Glycoprotein metabolism | ||||||
Congenital disorder of glycosylation 1a | + |
When one of the findings characteristic of IEMs is diagnosed during an ultrasound (US) or MRI examination, a differential diagnosis of the diseases known to be associated with this particular finding should be offered, followed by a repeat examination in search of other common anomalies known to be potentially present in the different entities (Table 12–3).
Fetal Ultrasonographic Features | Comments on Significance in Relationship to Inborn Errors of Metabolism |
---|---|
Intrauterine growth retardation | Nonspecific, wide differential, represents global effects of metabolic perturbation on the fetus |
Fetal akinesia/hypokinesia | Indicative of hypotonia, weakness in utero |
Anomalies in head size: microcephaly and macrocephaly | Indicates poor cerebral growth, can be severe in Amish microcephaly, macrocrania a feature of glutaric aciduria type I |
Forebrain development differentiation, midline anomalies | Holoprosencephaly is a feature of Smith-Lemli-Opitz syndrome |
Ventriculomegaly | Nonspecific feature indicates raised intracranial pressure, volume loss in the white matter |
Callosal abnormalities | Callosal dysgenesis is a marker for several IEMs (eg, NKH, PDH deficiency) |
Post fossa abnormalities | Cerebellar atrophy that is progressive is a feature of defects in energy metabolism, cerebellar hypoplasia is associated with CDG type 1a |
Neural tube segmentation | Disorders of folate metabolism |
Cerebral atrophy and calcifications | Nonspecific, indicates progressive disease as a consequence of neuronal loss/drop out, seen in mitochondrial disorders |
Intracranial hemorrhage, effusions | Subdural hemorrhages and effusions associated with organic acidemias (eg, GA1) |
Stroke/encephaloclastic lesions (porencephaly), | Nonspecific association with defects in energy metabolism, sulfite oxidase deficiency |
Schizencephalic clefts lissencephaly/pachygyria | These are more difficult to detect on US alone, may need fetal MRI, and follow-up postnatal imaging studies |
Pyruvate dehydrogenase alpha 1 (PDHA1), pyruvate dehydrogenase complex E1 alpha (PDHCE1A), OMIM *608769.
Pyruvate dehydrogenase alpha 1 deficiency is one of the most commont causes of congenital lactic acidosis. Mutations in genes coding for proteins involving the pyruvate dehydrogenase (PDH) complex (OMIM *300502 E1; EC 4.1.1.1) are associated with primary lactic acidosis presenting in the infantile period. The enzyme is a multienzyme complex with three components: pyruvate dehydrogenase (E1), dihydrolipoamide acetyltransferase (E2), and lipoamide dehydrogenase (E3).
The disorder is known to be associated with central nervous system (CNS) malformations in the prenatal period.5,6
The PDHCE1A complex catalyzes the first step involved in the conversion of pyruvate to acetyl coenzyme A (CoA). Mutations (occur de novo) in the gene coding for the alpha subunit of the PDHE1 component lead to an X-linked form of PDH deficiency.7,8,9,10,11
Infants present with either a metabolic form with severe lactic acidosis and encephalopathy at birth or in a neurologic form that may be detected prenatally on account of the associated anomalies. The phenotypic severity is linked to the underlying mutation and residual enzyme activity. Severe mutations tend to be lethal in male infants, whereas females present with brain abnormalities with minimal to no lactic acidosis. Milder mutations in males present with lactic acidosis and a neurologic phenotype, whereas females may be asymptomatic, often leading to a delay in diagnosis. The neuropathologic features associated include cerebral atrophy, cavitating lesions in the white matter and deep gray nuclei, callosal dysgenesis of varying severity, absence of the pyramids, heterotopias of the olivary complex, and abnormalities of the dentate nuclei.12
PDHCE1A is inherited as a mendelian X-linked condition. Both males and heterozygous females carrying one copy of the defective gene tend to be symptomatic.
Prenatal US examination could be useful in identification of ventriculomegaly, callosal dysgenesis, and posterior fossa abnormalities usually recognized in the newborn (Figure 12–2). MRI may bring a higher level of resolution to the abnormalities involving the brainstem and cerebellum (Figure 12–3). Lactate elevation in the brain can be demonstrated on magnetic resonance spectroscopy.13 Although enzyme activity in cultured fibroblasts is typically low, activity levels may be normal in heterozygous females; hence a reliable diagnosis requires a search for mutations in the gene coding for the PDHE1alpha subunit and should include DNA sequencing.14
Figure 12–2.
Axial sections on cranial ultrasound (US) in an infant with pyruvate dehydrogenase deficiency on day 1 of life. (A) Ventriculomegaly and posterior fossa cyst (arrowheads) can be identified. (B) Callosal dysgenesis (arrowhead) and unusual shape of the frontal horns are also identified. Note the abnormal sulcation in both figures.
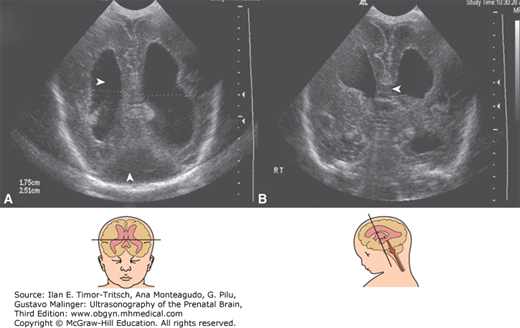
Figure 12–3.
MRI T1-weighted images of the same infant as in Figure 12–2 on day 1 of life. (A) Median section confirms callosal and posterior fossa abnormalities (arrowheads) with large extra-axial spaces due to a reduction in brain mass. (B) Axial section shows bilateral ventriculomegaly (arrowhead). Note the presence of diffuse white matter abnormalities and pachygyria.
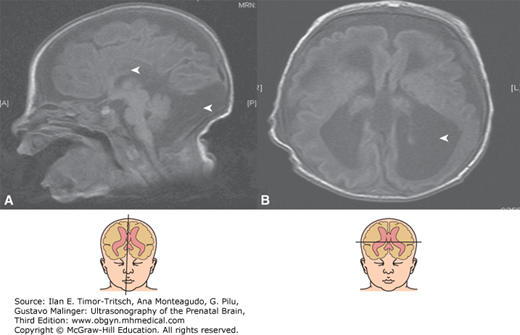
The principal differential diagnoses include mitochondrial disorders of the respiratory chain, which can also present with congenital lactic acidosis. With PDHCE1A deficiency, lactate/pyruvate ratios are normal in the cerebrospinal fluid (CSF), a distinctive feature in comparison to disorders of the respiratory chain.
When there is a history of a previous child with a neonatal presentation of PDH deficiency, a targeted exam should be obtained from 18 weeks’ gestation to monitor the development of the corpus callosum. Sonograms should be obtained every 2 to 3 weeks to monitor development of ventriculomegaly or periventricular cysts.
The changes in the brain in the form of ventriculomegaly and brain atrophy can be detected as early as 28 postmenstrual weeks.15 The occurrence of structural brain anomalies is influenced by gender and the severity of the mutation. Ultrasonography may be useful in the detection of structural anomalies by the end of the second trimester.
MRI of a fetus whose mother had a history of two prior infants affected with pyruvate dehydrogenase deficiency demonstrated ventriculomegaly, increased extra-axial CSF, and posterior fossa abnormalities (Figure 12–4). Magnetic resonance spectroscopy was normal. The diagnosis of PDH deficiency was made after delivery with the detection of severe lactic acidosis in the immediate neonatal period.16
Figure 12–4.
Single shot fast spin echo (SSFSE) fetal magnetic resonance imaging (MRI) during the third trimester of a patient with pyruvate dehydrogenase deficiency. (A) Sagittal section shows callosal and pontocerebellar abnormalities (arrowhead). (B) Axial section shows ventriculomegaly, particularly of the posterior horns (arrowhead).
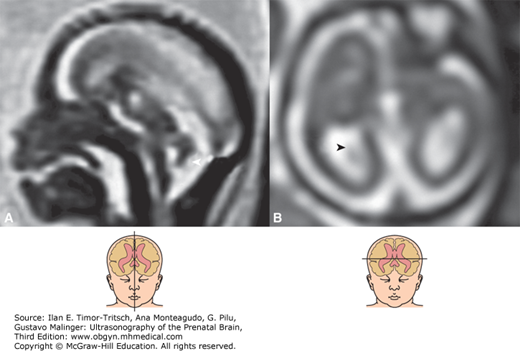
Management of a high-risk pregnancy should be made in coordination with a metabolic geneticist. Following delivery, the infant will need to be placed on a ketogenic diet, with symptomatic treatment for the management of lactic acidosis and seizures. In the absence of curative treatments, when brain malformations and/or extensive destructive lesions are identified, in utero termination of pregnancy, when legally possible, can be considered.
Some forms of the PDH deficiency are thiamine responsive, and thiamine supplements are helpful; lactic acidosis may be treated with the introduction of a ketogenic diet and the concomitant use of dichloroacetate. The ketogenic diet has been used successfully in the rescue of a zebrafish model for PDH deficiency.17 When there is established involvement of the prenatal brain, the prognosis is poor, and the children either die of fulminant lactic acidosis or are left with severe brain damage.
SLO syndrome, RSH syndrome, Rutledge lethal multiple congenital anomaly syndrome, polydactyly, sex reversal, renal hypoplasia, unilobar lung, lethal acrodysgenital syndrome, OMIM 270400
Smith-Lemli-Opitz (SLO) syndrome is a common birth defect (1:20,000–1:40,000) associated with malformations within multiple systems, craniofacial dysmorphic features, limb defects, and abnormalities of the heart, lungs, kidney, and genitalia18,19,20 (Figure 12–5).
Although two forms of the disorder are described, a severe form with neonatal presentation and a milder form, these likely represent two ends of a pathologic spectrum. The CNS involvement is highly variable, with microcephaly, hypoplasia of the frontal lobes, holoprosencephaly, callosal dysgenesis, and cerebellar hypoplasia typical of the severe forms of the disorder. However, considerable clinical heterogeneity exists, and milder forms can be more difficult to diagnose in the prenatal period on the basis of CNS features alone.21
The occurrence of multiple malformations involving the face, limbs (polydactyly and syndactyly), genital abnormalities (hypospadias, ambiguous genitalia, micropenis, hypoplastic scrotum, and bifid scrotum), and renal anomalies (agenesis, renal cysts, and hydronephrosis), along with CNS abnormalities, are well recognized features of SLO syndrome.
This condition is caused by a defect in the enzyme 7-dehydrocholesterol reductase (DHCR7; OMIM *602858, EC 1.3.1.21), involved in the pathway for cholesterol biosynthesis. The DHCR7 gene maps to 11q12-q13 locus. Plasma cholesterol levels are typically low, whereas the levels of the precursor 7-dehydrocholesterol are elevated.22
The disorder is autosomal recessive in its inheritance. Each sibling of an affected individual has a 25% chance of being affected, a 50% chance of being an asymptomatic carrier, and a 25% chance of being unaffected and not a carrier.
Maternal serum and urinary dihydroxysteroid ratios in combination with fetal anomalies detectable on US greatly enhance the likelihood of establishing a prenatal diagnosis.23,24 Identification of mutations in the gene encoding the enzyme sterol delta-7-reductase are diagnostic; common mutations can currently be identified through a polymerase chain reaction (PCR) assay.25,26
The findings of SLO syndrome can be mimicked by other conditions, both genetic and metabolic; however, rarely is the combination of more than two of the above described anomalies and the biochemical defect replicated in other conditions. Other genetic considerations are trisomies 13 and 18, Meckel syndrome, and Simpson-Golabi syndrome. Metabolic conditions that can be considered with similar sterol synthesis defects include β-sitosterolemia (abnormal sterol biosynthesis, normal to elevated cholesterol levels, episodic hemolysis, tuberous xanthomatosis, and early atherosclerosis), CHILD syndrome (congenital hemidysplasia, ichthyosiform nevus, and limb defects), desmosterolosis (macrocephaly, hypoplastic nasal bridge, thick alveolar ridges, gingival nodules, cleft palate, total anomalous pulmonary venous drainage, ambiguous genitalia, short limbs, and generalized osteosclerosis), mevalonic aciduria (normal to slightly reduced cholesterol levels, developmental delay, dysmorphic facial features, central cataracts, anemia, and hepatosplenomegaly), and X-linked dominant chondrodysplasia punctata (alopecia, cataracts, ichthyosis, punctate calcification of bones, and rhizomelic limb shortening).
A confirmed diagnosis of SLO syndrome in one child would suggest that the biological parents are likely carriers; consequently, future pregnancies carry a definite risk of recurrence. In such situations, the subsequent pregnancies should be followed from the first trimester so that abnormalities in the cleavage of the forebrain are not missed.
There is marked phenotypic variability in the presentation of SLO syndrome. A few reports have emphasized the clinical significance of the association of intrauterine growth retardation and nuchal edema detected on US examinations prenatally to be highly suggestive of SLO syndrome; however, milder cases may be missed despite the use of biochemical screening and prenatal ultrasonography.21,24 In a single large series examining the antenatal expression of the disorder, intrauterine growth retardation was the only consistent feature, often in combination with other anomalies such as nuchal edema and cerebral, renal, or limb malformations. A considerable proportion (~15%) of the prenatal US studies were reported as normal; an early diagnosis of multiple malformations was possible in only 10%23 (Figure 12–6). A combination of biochemical sterol analysis in the amniotic fluid and ultrasonographic examinations may be more helpful in milder cases.
Figure 12–6.
Prenatal US images of the same patient as in Figure 12–5 at 25 weeks of pregnancy. (A) Dysgenesis of the corpus callosum; the corpus callosum is thick and short (arrows), the splenium is missing and replaced by a cyst of the cavum interpositum (asterisk). (B) Cerebellar hypoplasia; the transverse cerebellar diameter is unusually small (2.06 mm = 19 weeks, 5 days). (C) Color Doppler of the heart showing atrioventricular canal. Other findings, not shown, included intrauterine growth retardation, microcephaly, pulmonic stenosis, and cleft palate.
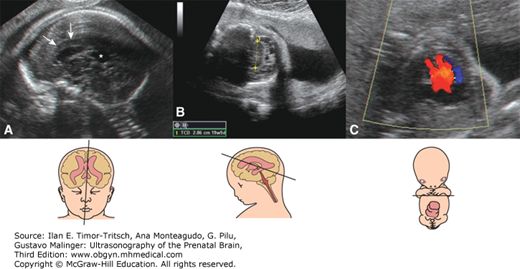
When severe, SLO syndrome is identified in utero; where legally possible, medical termination of pregnancy can be considered as an option.
The prognosis is variable, with severe malformations resulting in a shortened life span, whereas milder cases may be compatible with a normal life span. Dietary cholesterol supplementation, as well as the use of simvastatin, a 3-hydroxy-3-methylglutaryl (HMG) CoA inhibitor, improves the biochemical profile,27 but the effects on behavior remain unclear.
Glutaric acidemia type I, glutaryl CoA dehydrogenase deficiency, OMIM 231670
Glutaric aciduria type I is an autosomal recessive disorder resulting from an inherited defect in the glutaryl-CoA dehydrogenase enzyme (GCDH; enzyme commission number, EC 1.3.99.7; OMIM*231670). The disorder causes an acute devastating neurologic syndrome in infants that is characterized by sudden-onset hypotonia, dystonia, and encephalopathy often in conjunction with a febrile illness. Survivors often have dystonic movements, seizures, and developmental delay.
Neuropathologic features are fairly characteristic for this disorder: macrocrania and increased brain size and weight, subdural effusions and hematomas, a pattern of frontotemporal hypoplasia associated with incomplete opercularization, and atrophy of the caudate and putamina bilaterally.28
Pathologic changes are confined to the nervous system; macrocephaly at birth is usually a marker.
In a majority of patients, the principal pathologic changes follow the occurrence of encephalopathic crises often in postnatal life. A combination of the neurotoxic effects of intracerebral accumulation of intermediaries such as glutaric acid and 3-hydroxyglutaric acid and intrinsic vulnerability of striatal medium-sized γ-amino butyric acid neurons to neurotoxins and metabolic stress underlie the pathogenic effects.
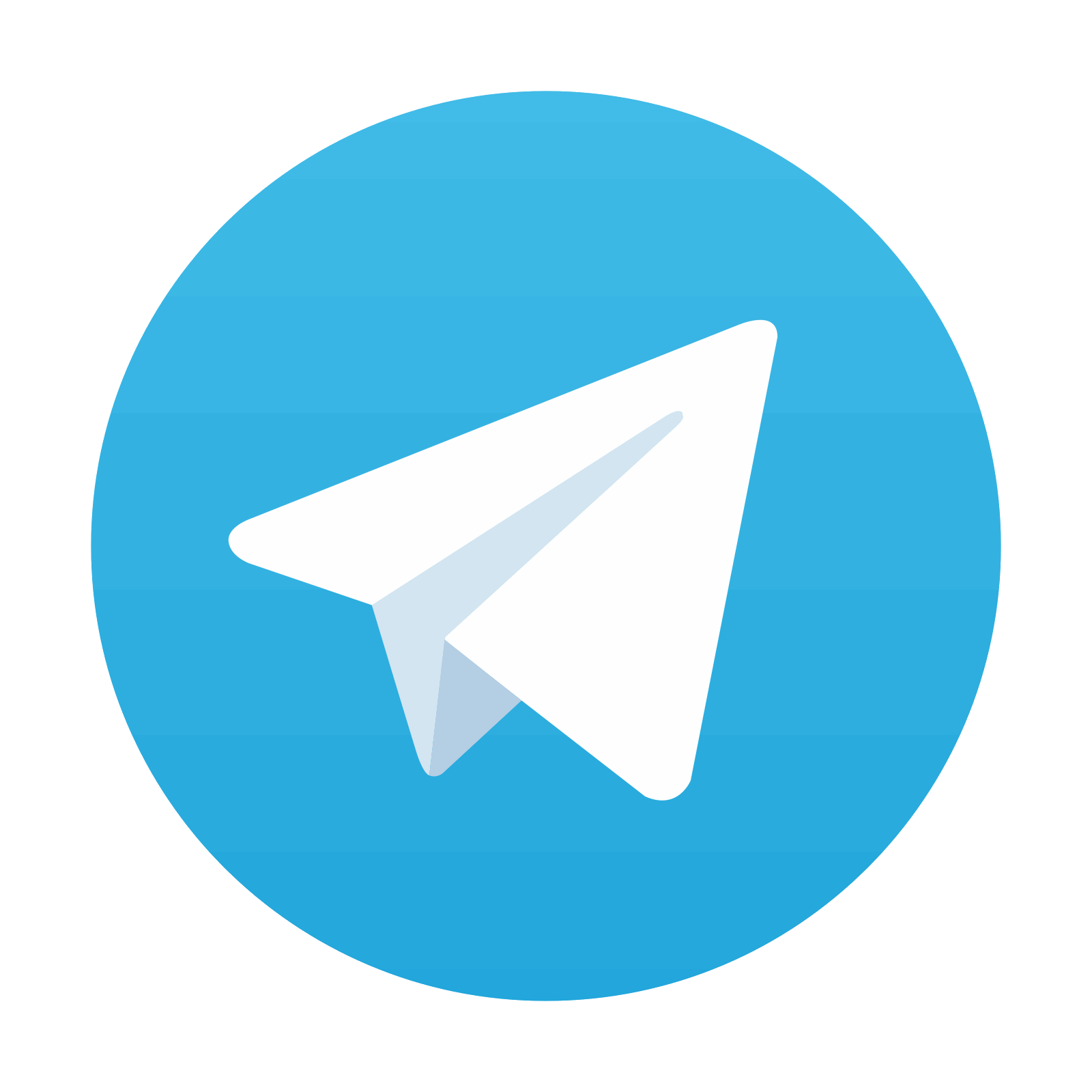
Stay updated, free articles. Join our Telegram channel

Full access? Get Clinical Tree
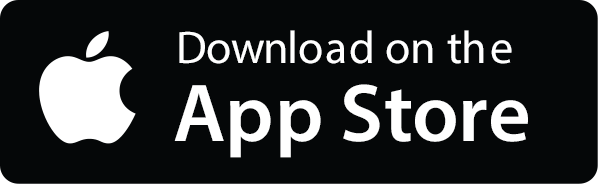
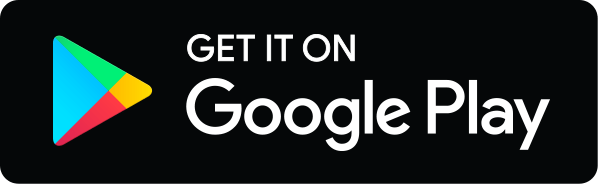