Fig. 14.1
Leukocyte SPECT/CT, performed at 4 h p.i., shows pathologic activity at the prosthesis proximal part, the presence of a chronic fistulization in the upper thigh (arrow), and an abscess collection near the distal part of the femoral shaft (dashed arrow), indicating a chronic infected hip prosthesis. Combining SPECT/CT fusion images enhances the exact localization and, in particular in this case, the extent of the inflamed tissues, probably more accurately than SPECT alone. Subsequently, the orthopedic surgeon can be prepared for an extended treatment strategy. Finally, note the presence of metal artifacts, generated on the low-dose CT (With kind permission from Springer Science + Business Media. Gemmel et al. [67]. Fig. 10)
14.2.2 Infected Vascular Graft
The reported rate of vascular graft infections ranges from 0.5 to 5 % and is associated with a high risk of mortality and morbidity (such as limb loss); so early diagnosis of these infections is of the utmost importance for the optimal timely management, which usually necessitates surgical intervention. In view of the remarkable consequences of a false-positive diagnosis, for the diagnosis of vascular graft infection, not only a high sensitivity but also a high specificity is needed. Imaging of vascular graft infection by labeled WBC SPECT has been tested previously, but the results were discouraging, as the test suffers from a chance of false-positive results caused by the inability to exactly localize the site of inflammation. Adding CT to SPECT imaging increases the diagnostic accuracy: on CT scans, air bubbles could be found around the infected graft in almost half of the cases; however, this finding is also highly nonspecific, because in 50 % of grafts these bubbles are present for weeks to months after graft implantation [32]. Also, CT is often false-negative in chronic low-grade infections, and it is challenging to differentiate between acute infection, hematoma, and lymphocele on CT scans. Based on these limitations, PET/CT with a sensitivity exceeding 90 %, the specificity of 64 %, positive and negative predictive values of 88 and 96 %, has been preferred over SPECT, CT, or combined SPECT/CT imaging [32].
14.3 Imaging of Noninfectious Inflammatory Disease
14.3.1 Rheumatoid Arthritis
Rheumatoid arthritis is an inflammatory arthropathy, for which a remarkable number of new therapeutic strategies, including biological agents such as anti-TNF antibodies, have emerged. Regarding the importance of the individualized use of disease-modifying antirheumatic drugs, such as methotrexate and biological agents, monitoring of response to treatment is one of the most challenging issues in front of rheumatologists, a measurement which cannot always be easily done by clinical criteria [25]. Another aspect of the diagnostic approach to RA is also the need to stratify patients into groups with a low risk or a high risk of rapid disease progression and severe destructive arthropathy. Imaging approaches have been employed to address these issues. Ultrasonography, MRI, and a number of scintigraphic approaches (including nanocolloids and 3-phase bone scintigraphy and 18F-FDG PET) have been used to detect synovitis, as well as increased synovial blood flow in affected joints. Selection of the best imaging approach to address the clinical question depends on different factors: ultrasonography is widely available, sensitive, inexpensive, convenient, and with no ionizing radiation, so it is increasingly becoming the first-line modality of screening for suspected RA or other inflammatory arthropathy patients. It may also show bone erosions in small joints (finger and toe joints) with high sensitivity [19]. MRI, although is the gold standard imaging modality for the detection of synovitis and sensitive in the detection of bone erosions, is expensive and less convenient for patients. Plain radiography is not sensitive enough to detect early lesions in RA.
Bone scintigraphy has been employed to image disease activity with planar imaging clearly less sensitive than SPECT, so minimal changes in bone metabolism may be missed [2]. For example, multipinhole SPECT of the hands has been employed to detect early stages of the rheumatoid disease with the sensitivity equal to or even more than MRI in some cases [16]. 99mTc-nanocolloids scintigraphy has also shown high diagnostic accuracy, but with limited clinical application due to advantages of competing modalities (ultrasound, MRI, and bone scintigraphy), mainly their broad availability. PET with 18F-FDG or 11C-choline has been studied for imaging of synovial inflammation and measurement of cell proliferation and has been proved to have high diagnostic accuracy [17]. Although scintigraphy with either 99mTc-nanocolloids or bisphosphonates could be employed in the diagnosis of RA at its early stages, its role in the evaluation of response to treatment requires repeated imaging as often as every 6–12 weeks. However, radiation exposure associated with nuclear medicine techniques puts ultrasonography and MRI at an advantage [14].
14.3.2 Sarcoidosis
SPECT/CT with 67Ga-citrate has been used for imaging of sarcoidosis, in fact for determination of disease activity, monitoring of response to treatment, detection of sites of disease previously unknown, and unsuspected metabolically active disease [20–28, 34]. However, recent studies have shown that 67Ga offers no advantages over 18F-FDG PET/CT for imaging of inflammatory diseases; therefore, Ga scintigraphy has been largely replaced by PET/CT for such a purpose [26].
In the diagnosis of primary sarcoidosis, high 67Ga uptake could be seen in lymph nodes and other involved organs [20–25]. The lambda sign is seen on 67Ga scans in the setting of thoracic sarcoidosis. Bilateral hilar and right paratracheal lymph nodes are typically involved which can resemble the lambda symbol. The panda sign is seen as a result of 67Ga citrate accumulation in both regions of chronic and regions of acute inflammation. When the normal accumulation of the radionuclide in the nasopharynx is combined with increased symmetric accumulation in the parotid and lacrimal glands, the image shows a striking similarity to the mottled coloring of the giant panda. In response to treatment, uptake of 67Ga decreases, allowing for assessment of treatment effects and for early adaptation of therapy if no response is seen (Fig. 14.2).
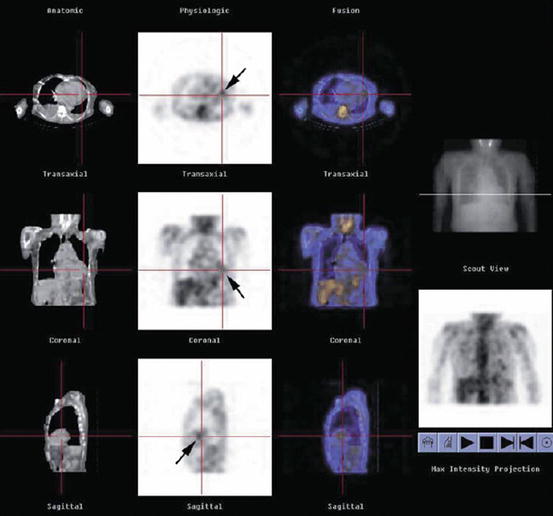
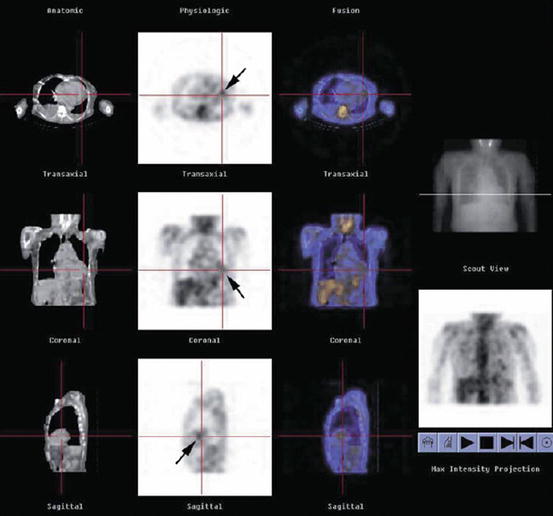
Fig. 14.2
A 60-year-old female patient with cardiac sarcoidosis. Single-photon emission computed tomography (SPECT)/CT (low-dose CT, SPECT, and fusion image) showing an abnormal uptake (arrows) near cardiac apex (With kind permission from Springer Science + Business Media. Momose et al. [68])
14.3.3 Inflammatory Bowel Disease (IBD)
Ulcerative colitis and Crohn’s disease are the two main subtypes of IBD, the diagnosis of which depends on direct endoscopic visualization of the GI mucosa and the histological evaluation of the tissue samples. Radiological and scintigraphic methods are mainly employed as an adjunct to endoscopy [2]. Although disease activity is usually measured clinically by clinical activity scores, endoscopy, or barium enteroclysis, this diagnostic approach has major disadvantages, as clinical activity scores are indirect and endoscopy and barium enteroclysis are both invasive techniques, which in case of severe disease predispose the patient to procedure-related complications, like colon perforation. Similarly, barium may be contraindicated in cases of severe inflammation because of the risk of perforation and subsequent peritonitis. Therefore, noninvasive imaging procedures are needed to adequately follow patients with IBD [2, 6].
Labeled leukocyte imaging (preferably with 99mTc instead of 111In due to better image quality) has been extensively employed for evaluation of IBD, mainly to detect involved bowel segments and localization of the disease as well as early diagnosis of resistance to therapy. WBC scan is unlikely to miss severe inflammation, and the sensitivity and specificity of labeled WBC scanning for the correct detection of involved bowel segment have been reported to be between 80 and 90 % in adults and children [1, 3–5, 7]. WBC scanning may also help to diagnose therapy resistance within days after the onset of therapy [7]. WBC scanning requires no bowel preparation, causes no patient discomfort and offers less radiation as compared to the competitive imaging modalities: the effective radiation dose for 99mTc-HMPAO WBC imaging is 3 mSv, for barium small bowel follow-through is 6 mSv, and for barium enema is 8.5 mSv [8].
Horsthuis et al. have demonstrated that WBC scanning is more sensitive than CT, but equivalent to ultrasonography and MRI in IBD imaging, with sensitivities ranging from 84 to 93 % and specificities ranging from 84 to 95 % [3]. Mainly based on the absence of radiation exposure, ultrasonography and MRI have been suggested as primary imaging modalities for IBD; however, it has been emphasized that ultrasonography is operator dependent, and usually not all bowel segments can be imaged by ultrasound technique [2]. For labeled leukocyte imaging, SPECT/CT should be performed for the correct localization of involved bowel segments. Controversy still exists about the optimal imaging time after infusion of labeled WBCs to the patient. Early scanning (30–60 min) has been recommended by some authors in order to avoid false-positive results caused by intestinal migration of the radionuclide, whereas late scanning (3 h) has been favored by others because of higher sensitivity [8]. Slightly lower specificity but higher sensitivity (85 % vs 100 %) and accuracy (85 % vs 95 %) of late scanning as compared to early scanning has been reported [12].
99mTc-Pentavalent dimercaptosuccinic acid [DMSA (V)] has been reported to be an accurate scintigraphic modality and has been suggested as a complementary technique to colonoscopy for the follow-up of patients and assessment of IBD activity [9, 10, 13]. Scintigraphic imaging of IBD with 111In-anti E-selectin monoclonal antibodies and 99mTc-labeled anti-granulocyte monoclonal antibodies has been insufficiently studied and warrants further investigation [8].
14.4 Fever of Unknown Origin (FUO)
Although a number of definitions are found for FUO, the most commonly acceptable definition is a temperature of at least 38.3 °C (101 °F) for more than 3 weeks, with the underlying cause being uncertain after 1 week of inpatient work-up. The potential causes of FUO include a broad spectrum of diseases, mostly infectious diseases and autoimmune disorders. One third of the cases are due to an occult malignancy.
Although usually anatomic imaging is the first diagnostic approach to the patient with FUO, it suffers from remarkable limitations:
It covers just a limited area of the body, which is already selected by the referring physician, and always there is a risk that the source of fever is not included in the imaged field. Such a limitation is not present in most of nuclear medicine imaging techniques that are used for this purpose (e.g., 67Ga scintigraphy, 18F-FDG PET), as they are performed as whole body imaging examinations. However, SPECT/CT has the same limitation, which is mostly due to the CT component. On the other hand, anatomic imaging modalities detect anatomic changes in tissues. These changes usually are not developed, unless the underlying disease progresses beyond a specific level of tissue distortion and derangement. Functional imaging of nuclear medicine does not suffer from such a limitation and is able to detect the disease processes in an earlier stage. In addition, changes caused by previous cured diseases, radiation, or surgical interventions (scars, fibrosis) may result in false-positive interpretation of the anatomical images, a problem which is significantly less common in functional imaging (like SPECT/CT).
67Ga-citrate is one of the most common agents used for the scintigraphic imaging of FUO. Whole body imaging is a great advantage of the technique, which is added to the ability of spot imaging of the areas of interest with SPECT acquisition. These areas of interest are chosen by the nuclear physician, based on the history and findings of the whole body scan. The proven efficiency of 67Ga-citrate scintigraphy in the detection of inflammation is the avidity of 67Ga-citrate for inflammatory molecules in the inflamed tissues. However, 67Ga-citrate scintigraphy suffers from some limitations such as delayed clearance of radiotracer from the background, which requires delayed image acquisition to achieve acceptable target-to-background ratio. High γ-energy emitted by 67Ga also impairs image quality by lower resolution resulting from the use of high-energy collimators. On the other hand, 67Ga-citrate scintigraphy is time consuming and has a relatively high radiation burden. Meller et al. have shown that 67Ga scintigraphy has a diagnostic yield nearly equivalent to that of 18F-FDG coincidence PET [30–32]. The alternative scintigraphic modality is labeled WBC scan, which may be used to identify infectious foci in patients with FUO. However, WBCs accumulate mainly in the foci of bacterial infections, and the scan usually has a low sensitivity in the detection of the other potential causes of FUO, such malignancies and autoimmune diseases, with a remarkable number of false-negative scans in FUO. Therefore, the clinical value of labeled WBCs for detection of the underlying etiology of FUO is limited. Considering the limitations of 67Ga scintigraphy and labeled WBC scan, 18F-FDG PET is the procedure of choice for the imaging evaluation of FUO, as main underlying causes of FUO (infection, autoimmune disease, and malignancy) can be detected with a high sensitivity by a single imaging. Different radiopharmaceuticals used for inflammation imaging have been listed on Table 14.1.
Table 14.1
Radiopharmaceuticals used for inflammation imaging
Radiopharmaceutical
Mechanism of action
99mTc/111In-labeled autologous WBCs (white blood cells)
Active migration of WBCs to the sites of infection/inflammation
67Ga-citrate
Increased perfusion of the inflamed tissues, local binding to lactoferrin, extravasation due to increased vascular permeability
99mTc-labeled bisphosphonates
Increased delivery and diffusion to the sites of infection/inflammation due to increased perfusion and extravasation (early angiographic and blood pool phases) and increased bone uptake due to higher osteoblastic activity (late phase)
99mTc-labeled nanocolloids
Uptake in macrophages (inflammation, bone marrow, liver, spleen), increased extravasation
99mTc/111In-labeled proteins (IgG, albumin)
Extravasation (increased perfusion and vessel permeability)
14.5 Brain Imaging
Although functional imaging provides invaluable diagnostic information in many patients with neurological disorders, currently CT and MRI have substituted functional imaging. The most important indications of functional imaging with brain SPECT/CT are assessment of brain tumors, particularly for differentiation of postradiation necrosis versus residual/recurrent tumor, differential diagnosis of Parkinson disease and dementia, detection of seizure focus, detection of brain death, and evaluation of substance abuse.
14.5.1 Parkinson Disease
Parkinson disease is one of the best examples in which functional imaging has been shown to be superior to anatomic imaging regarding clinical diagnostic efficacy. It provides invaluable information not obtainable by the other imaging modalities, which significantly influence decision making and can potentially change the therapeutic plan. 123I-ioflupane with commercial name of DAT is the most commonly used and the most widely studied radiopharmaceutical for the assessment patients suspected for Parkinson disease. It is actually one of the best radiotracers to visualize the striatum using SPECT, showing the distribution of the dopamine transporters in the striatum. After reorientation of head to avoid false impressions of asymmetry due to head tilting, images are visually interpreted [35–37].
In a normal scan the striatum is seen as symmetric, comma‐shaped regions, with both caudate and putamen showing high uptake compared to background activity. With increasing age, a reduction in the target-to-background ratio is seen (5–8 % decrease in ratio per decade). In early Parkinson disease, there is usually an asymmetrical pattern of reduced DaT binding initially in the dorsal putamen contralateral to the clinically most symptomatic body side. The radiotracer binding gradually progresses anteriorly and ipsilaterally as the disease progresses. Although the activity in the caudate nucleus declines with advancing disease, it is relatively preserved in early disease. In the atypical parkinsonian syndromes, there usually is a more symmetrical decrease in DaT binding, and more involvement of caudate is detected. However, the overlap between scintigraphic features is sufficiently high not to reliably differentiate between different parkinsonian syndromes. In Lewy body dementia, DaT binding is reduced bilaterally, mainly in the putamen but also in the caudate. The reduction of radiotracer binding is generally with less asymmetry than seen in Parkinson disease.
14.5.2 Stroke
Flow imaging with radiopharmaceuticals, which reflect regional cerebral blood flow (rCBF), has been used for early detection of stroke. Two 99mTc-labeled radiopharmaceuticals for brain flow imaging are available: hexamethylpropyleneamineoxime ([99mTc-HMPAO] Ceretec) and ethylcysteinate dimer ([99mTc-ECD] bicisate, Neurolite). Although absolute rCBF measurement using Xenon as the radiotracer is possible, such a measurement with Technetium agents has not been fully implemented, and the region of interest (ROI) analysis of rCBF has become the preferred method of quantification with these radiopharmaceuticals [38–45].
In the very early hours of stroke, rCBF is reduced, while tissue oxygen metabolism is maintained. This flow-metabolism mismatch is called “misery perfusion.” In later stages, ischemia progresses and damage to blood vessels might lead to excessive or “luxury perfusion.” This sequence of scintigraphic findings has been used to determine which patients may benefit from thrombolytic therapy. Some studies have shown that SPECT is able to detect the ischemic events even before MRI and the ischemic area is larger in SPECT images as compared to MRI. Such a difference is partly explained by the higher sensitivity of SPECT in detecting the penumbra area, the area that benefits from early reperfusion interventions and is at the highest risk for expansion of irreversible injury. The best candidates for fibrinolytic therapy would be those patients presenting immediately after the onset of clinical symptoms and showing an area of reduced tracer uptake (hypoperfusion) on the SPECT images. In fact, a normal brain SPECT image or just an area of hypoperfusion implies a favorable prognosis as a result of effective collateral circulation. By contrast, an area of no radiopharmaceutical uptake (cold area or absent perfusion) is more likely to be associated with hemorrhagic complications if fibrinolysis is employed. For SPECT-driven decision making, brain SPECT should be done between the first 3 and 6 h after the onset of stroke symptoms, which is usually not possible in routine clinical practice.
SPECT also has been shown to be effective in the study of transient ischemic attacks (TIA). A pharmacologic stress using acetazolamide (Diamox) injection has been employed to unmask the underlying vasculopathies limiting the vasodilatory response to Diamox. In fact, abnormal vessels do not show dilatory response to Diamox, and the blood flow is shunted away from them, leading to a kind of steal phenomenon. The final outcome in scintigraphic imaging is a significant reduction in radiotracer uptake of the cerebral regions being fed by the affected blood vessels. The rationale for the imaging is comparable to what is employed in myocardial perfusion imaging using dipyridamole.