Fig. 6.1
Representations of well-characterized oxidation-specific epitopes and antibodies. Oxidation-specific antibodies recognize protein and lipid epitopes on oxidized LDL (OxLDL). These epitopes include malondialdehyde (MDA), phosphocholine-containing oxidized phospholipids (PC-OxPL), and 4-hydroxynonenal (HNE)
6.2 Antibodies and Peptides to Oxidation-Specific Epitopes
Table 6.1 summarizes the murine and human OSE autoantibodies and peptides with potential in clinical diagnostic imaging applications.
Table 6.1
Properties of murine and human OSE autoantibodies and peptides proposed for imaging applications
Antibody | Source | Epitopes bound | Conjugated labels |
MDA2 | Murine monoclonal IgG | MDA-LDL | 125I, 99Tc |
MDA-modified proteins | Gd micelles | ||
Mn micelles | |||
Mn dendrimers | |||
LUSPIO micelles | |||
LSPIO micelles | |||
Rhodamine micelles | |||
E06 | Natural murine monoclonal IgM | PC on oxidized phospholipids | Gd micelles |
Mn micelles | |||
LUSPIO micelles | |||
LSPIO micelles | |||
Rhodamine micelles | |||
IK17 (scFv) | Human monoclonal IgG | MDA-LDL | Gd micelles |
Copper OxLDL | Mn micelles | ||
LUSPIO micelles | |||
LSPIO micelles | |||
Rhodamine micelles | |||
IK17 (scFab) | Human monoclonal IgG | MDA-LDL | Gd micelles |
Copper OxLDL | Rhodamine micelles | ||
Mimotope | Characteristic | Epitopes mimicked | Antibodies bound |
P1 | 12-mer linear peptide | MAA | LR04 |
MDA-LDL | IK17 | ||
Copper OxLDL | Human IgG autoantibodies | ||
Human IgM autoantibodies | |||
P2 | 7-mer cyclic peptide | MAA | LR04 |
MDA-LDL | IK17 | ||
Copper OxLDL | Human IgG autoantibodies | ||
Human IgM autoantibodies |
MDA2 was one of the first monoclonal antibodies (IgG2a type) isolated and generated against OSEs that are formed during the oxidative modification of LDL [35]. MDA2 was generated from hybridomas derived from mice immunized with homologous murine malondialdehyde(MDA)-modified LDL. MDA2 is a hapten-specific antibody that recognizes MDA-modified proteins, such as MDA-LDL and other modified proteins present within atherosclerotic lesions, without any binding to native LDL, high-density lipoprotein, or very low-density lipoproteins [10, 36, 37].
The “natural” monoclonal antibody E06 (IgM type) antibody was discovered and characterized from splenic lymphocytes of apoE−/−mice [38, 39]. E06 binds to the phosphocholine (PC) head group of oxidized, but not native, phospholipids [40]. E06 effectively blocks the uptake of OxLDL and apoptotic cells by mouse macrophages [41] and protects mice against atherosclerosis [42], thereby demonstrating potential therapeutic benefit [14]. Interestingly, the variable region of E06 is structurally identical to “natural” murine T15 IgA antibodies that bind unoxidized PC of pneumococcus PC and protect mice against lethal infection [43]. It is believed that this class of autoantibodies may have evolved and been preserved through natural selection in response to defense against PC-containing organisms such as pneumococci and clearance of apoptotic cells [3].
IK17 Fab, the first fully human OSE monoclonal antibody, was generated from lymphocytes of a patient with coronary artery disease [44]. Total RNA was isolated from the patient’s peripheral blood mononuclear cells and was used to synthesize cDNA. Reverse transcription-polymerase chain reactions were performed, and the polymerase chain reaction products pooled according to isotype. The isotypes were then cloned into the phage display vector, pComb3H, to generate 2 phage display libraries, VH/Vκ and VH/Vλ. The resultant phagemid DNA was transformed into XL-1 blue Escherichia coli, rescued with a helper phage, and panned against MDA-LDL. The phagemid DNA was then prepared from infected bacteria and manipulated to express IK17 by removing gene III, which is fused to the C-terminus of the heavy chain gene and is essential for anchoring the Fab on the phage surface. IK17 binds to an advanced MDA-like epitope, malondialdehyde-acetaldehyde (MDA-MAA), which is expressed during extensive oxidative modification and in advanced human atherosclerotic lesions and vulnerable and ruptured plaques [11, 44–48]. IK17 has been shown to inhibit the uptake of OxLDL and apoptotic cells by macrophages similar to E06 [44]. For diagnostic imaging, both the Fab fragment and the lower-molecular-weight single-chain Fv (scFv) fragment have been used [44–47]. Overexpression of scFv IK17 with adenovirus or infusion of IK17 Fab results in reduced progression of atherosclerosis in susceptible mouse models [48]. The discovery of this human autoantibody has clinical implications not only from a diagnostic standpoint (reduced immunological concerns relative to the clinical use of murine antibodies) but also as a therapeutic agent.
Peptides are advantageous from a clinical and commercial point of view as they are less immunogenic and are more cost-effective to produce, relative to their antibody counterparts. However, in order for a peptide to maintain the efficacy of the native antibody, the functional group of the antibody needs to be fully understood and sequenced. Currently 12-mer linear (P1) and one 7-mer cyclic (P2) peptides have been identified using phage display libraries and screened using binding specificity to murine and human anti-MDA monoclonal antibodies [49]. Additionally, these peptides mimic MDA and related epitopes that are present on the surface of murine apoptotic cells. Immunization of mice with the cyclic P2 peptides resulted in the generation of MDA-LDL-specific autoantibodies. In human studies, mimotopes will provide standardized, chemically defined antigens and are a paradigm in the generation of potential antibody mimics for diagnostic imaging.
Overall, these findings suggest that the OSE-targeted antibodies and mimotopes may have significant potential in biotheranostic (biomarker, diagnostic imaging, and therapeutic) applications [14, 50]. Table 6.2 summarizes the characteristics of all OSE molecular probes.
Table 6.2
Compilations of imaging studies targeting oxidation-specific epitopes
Date | Authors | Modality | Label/dose | Platform | Antibodies | Animal models | Summary of key findings |
---|---|---|---|---|---|---|---|
1999 | Tsimikas et al [37] | Planar/ SPECT | 99Tc/ 90 μCi 125I 125I | Direct conjugation | In vivo imaging | In vivo imaging | MDA2 specifically images atherosclerotic plaques in vivo |
MDA2 | 7 WHHL rabbits | ||||||
2 NZW rabbits (controls) | |||||||
pKa | pKa | First demonstration that oxidation-specific epitopes can be imaged in live animals | |||||
MDA2 | 7 WHHL and 2 NZW rabbits | ||||||
Halb (control Ab to human albumin) | 5 WHHL and 2 NZW rabbits | ||||||
Biodistribution | Biodistribution | ||||||
MDA2 alone | 5 WHHL rabbits | ||||||
MDA2 with MDA-LDL | = 7 WHHL rabbits | ||||||
2001 | Autoradiography | 125I | Direct conjugation | In vivo imaging | In vivo imaging | In vivo uptake of radiolabeled MDA2 provides an accurate measure of atherosclerotic lesions rich in oxidized LDL and is highly sensitive to their regression and plaque stabilization. Autoantibodies to OxLDL correlate with atherosclerosis progression and regression | |
MDA2 | 16 WHHL rabbits | ||||||
Halb (control Ab to human albumin) | 8 NZW rabbits (controls) | ||||||
Progression/regression study | |||||||
81 LDLR−/− mice | |||||||
2008 | Briley-Saebo et al [47] | MRI | Gd/0.075–mol/kg | Micelles | In vivo imaging | In vivo imaging | MRI nanoparticles containing Gd-labeled MDA2, IK17, and E06 enhance the aorta of mice with atherosclerosis |
Gd/0.075–mol/kg | |||||||
Gd/ 0.075–mol/kg | MDA2 | 8 apoE−/− mice | Unlabeled MDA2 competitively inhibits enhancement with Gd-MDA2 | ||||
IK17 | 3 apoE−/− mice | Targeted micelles co-localized with macrophages by confocal imaging | |||||
E06 | 8 apoE−/− mice | ||||||
IgG (control) | 3 apoE−/− mice | ||||||
pKa | pKa | ||||||
MDA2 | 30 apoE−/− and 14 WT mice | ||||||
IK17 | |||||||
E06 | |||||||
IgG (control) | |||||||
Biodistribution | Biodistribution | ||||||
MDA2 | Total of 30 apoE−/− mice | ||||||
IK17 | |||||||
E06 | |||||||
IgG (control) | |||||||
2011 | Briley-Saebo et al [45] | MRI | Fe/3.9 mg/kg | Lipid-coated, ultrasmall superparamagnetic iron oxide particles (LUSPIOs) | In vivo imaging | In vivo imaging | Specific signal accentuation within the aorta observed following administration of labeled MDA2, IK17, and E06 but not untargeted LUSPIOs |
Fe/3.9 mg/kg | MDA2 | 8 apoE−/− mice | Signal effect is competitively inhibited by unlabeled MDA2 | ||||
Fe/3.9 mg/kg | IK17 | 3 apoE−/− mice | LUSPIOs co-localize with intra-plaque macrophages, assessed by immunohistochemistry and confocal imaging | ||||
E06 | 8 apoE−/− mice | LSPIOs are too large to enter plaques and cannot image lesion adequately | |||||
Untargeted (control) | 8 apoE−/− mice | ||||||
Lipid-coated, superparamagnetic iron oxide particles (LSPIOs) | In vivo imaging | In vivo imaging | |||||
MDA2 | 8 apoE−/− mice | ||||||
IK17 | 3 apoE−/− mice | ||||||
E06 | 8 apoE−/− mice | ||||||
Untargeted (control) | 8 apoE−/− mice | ||||||
LUSPIO and LSPIO | pKa | pKa | |||||
MDA2 | 8–10 apoE−/− and 8–10 WT mice in each group | ||||||
IK17 | |||||||
Untargeted (control) | |||||||
2011 | Vucic et al [54] | PET | 18F-FDG/1 mCi/kg | None | None | 6 NZW rabbits for each imaging modality | Pioglitazone decreased PET and DCE-MRI signal in the aorta associated with decreased macrophages and OxPL immunostaining |
DCE-MRI | None | ||||||
2012 | Briley-Saebo et al [46] | MRI | Mn/0.050 mol/kg | Micelle | In vivo imaging | In vivo imaging | Labeled OSE antibodies enhance the aorta in both apoE−/− and LDLR−/− mice. MDA2-Mn micelles are located within intra-plaque macrophages on confocal imaging |
MDA2 | 8 apoE−/− mice and 8 LDLR−/− mice | 97 % of MDA2-Mn micelles cleared within 1 week postinjection, compared to 72.9 % clearance for MDA2-Gd micelles | |||||
IK17 | 8 apoE−/− mice | ||||||
Untargeted (control) | 8 apoE−/− mice and 8 LDLR−/− mice | ||||||
Gd/0.050 mol/kg | MDA2 | 8 apoE−/− mice | |||||
Untargeted (control) | 8 apoE−/− mice | ||||||
Mn/0.050 mol/kg | pKa | pKa | |||||
MDA2 | 3 apoE−/− and 3 WT mice in each group | ||||||
IK17 | Biodistribution | ||||||
Untargeted (control) | 45 apoE−/− mice (N = 3 for each antibody/time point) | ||||||
Mn/0.050 mol/kg | Biodistribution | ||||||
MDA2 | |||||||
IK17 | |||||||
Untargeted (control) | |||||||
2012 | Vucic et al [55] | PET | 18F-FDG/1 mCi/kg | None | None | 6 NZW rabbits for each imaging modality | LXR agonist, but not atorvastatin, was associated with decreased PET and DCE-MRI signal and decreased macrophage and OxPL immunoreactivity |
DCE-MRI | None | ||||||
2014 | Nguyen et al [56] | MRI | Mn/0.050 mol/kg | G8 dendrimers | In vivo imaging | In vivo imaging | G8 dendrimers contain a large payload of Mn compared to micelles (768 ions on a dendrimer vs. 50 in a micelle) |
MDA2 | 4 apoE−/− mice | Mn G8 dendrimers circulate with a half-life of 2.9 h, compared to Mn micelles, which have a half-life of 16.4 h | |||||
Untargeted (control) | 3 apoE−/− mice | Labeled MDA2, but not untargeted G8 dendrimers, enhance the aorta apoE−/− mice on MRI | |||||
pKa | pKa | ||||||
MDA2 | 3 apoE−/− and 3 WT mice in each group | ||||||
Untargeted (control) | |||||||
Biodistribution | Biodistribution | ||||||
MDA2 | 18 apoE−/− mice (N = 3 for each antibody/time point) | ||||||
Untargeted (control) |
6.3 The Role of OSE in Atherogenesis and Disease Progression
The direct correlation between OSE generation and deposition in the arterial wall and the presence of atherosclerosis and plaque progression has been demonstrated using direct extraction of modified LDL from the vessel wall [35, 57] and with immunostaining techniques in a variety of atherosclerotic animal models, including different strains of mice and rabbits, as well as monkeys and humans [11–13, 35–37, 39, 43–46, 48, 51–55, 58–60]. In general, these studies document the strong presence of oxidized lipids in early and late atherosclerotic lesions and in lesion progression. Furthermore, in dietary regression studies in LDLR−/− mice and cynomolgus monkeys, the deposition of OSE was greatly reduced in the arterial wall during atherosclerosis regression [53, 58]. Reduced OSE by immunostaining within the atheroma correlated strongly with features of plaque stabilization, including reduced macrophage content and increased collagen and smooth muscle cell content.
In humans with sudden cardiac death, there is evidence of differential expression of OSEs and apolipoprotein (a) [apo(a)] in different stages of plaque progression and plaque rupture [11]. In the normal coronary artery, OSEs and apo(a) could not be detected using the current immunostaining techniques. In early lesions, only nominal OSEs and apo(a) deposition was observed. Interestingly, as the lesions progressed, apoB and MDA epitopes did not significantly increase. However, OxPL and IK17 epitope expression increased linearly with advancing plaque stage. These OSEs were mainly associated with foam cell-rich areas, lipid pools, and necrotic cores that are associated with advanced, unstable, and/or ruptured plaques. Correlations have also been observed with iron deposition and IK17 epitopes in human abdominal aortic lesions in patients with the Hp2-2 genotype associated with increased oxidative stress [60]. Finally, in humans undergoing coronary, carotid, renal, and peripheral procedures, direct evidence of the presence of specific OxPL and typo, as identified by liquid chromatography and tandem mass spectrometry, was documented in distal protection devices (Fig. 6.2) [12, 13].
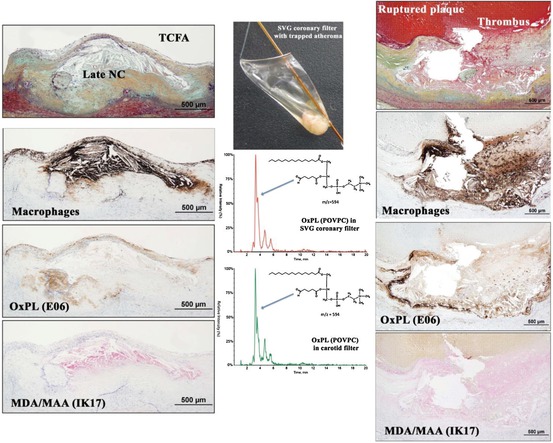
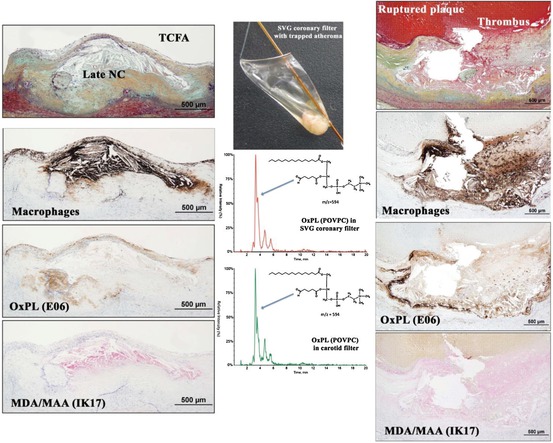
Fig. 6.2
The presence of macrophages and oxidation-specific epitopes in thin cap fibroatheroma (TCFA, left panels) and ruptured coronary plaques (right panels) detected by immunohistochemistry and coronary and carotid distal protection devices (middle panels) detected by LC-MS/MS (Reprinted with permission from van Dijk et al. [11])
6.4 Molecular Imaging Probes for In Vivo OSE Detection
Table 6.3 summarizes the imaging studies using OSE-specific antibodies.
Table 6.3
Characteristics of various magnetic resonance nanoparticles targeting oxidation-specific epitopes
Formulation | Size (nm) | Relaxivity 60 MHz, 40 °C s−1 mM−1 | Blood half-life (h) | %ID Liver 24 h p.i. apoE−/− mice | Enhancement in the arterial wall at the optimal time point p.i. apoE−/− mice | Optimal time point for imaging and dose | |
---|---|---|---|---|---|---|---|
apoE−/− mice | WT mice | ||||||
Gd Micelles | r1 | %NENH, 9.4 T | 72 h | ||||
MDA2 | 22 ± 2 | 9.3 | 14.3* | 1.7 | 18 | 125 | 0.075-mmol Gd/kg |
IK17 | 16 ± 3 | 10.5 | 16.5* | 1.8 | 18 | 138** | |
E06 | 16 ± 3 | 10.8 | 20.1* | 1.7 | 32 | 231** | |
IgG | 16 ± 4 | 10.4 | 1.4 | 1.3 | 18 | −0.6 | |
Untargeted | 14 ± 2 | 11.6 | 1.5 | 1.5 | 12 | 15 | |
Mn Micelles | r1 | %NENH, 9.4 T | 48 h | ||||
MDA2 | 18 ± 3 | 4.1 | 16.4*,+ | NA | 5 | 141** | 0.05-mmol Mn/kg |
IK17 | 12 ± 2 | 3.8 | 12.3*,+ | NA | 5 | 135** | |
E06 | NA | NA | NA | NA | NA | NA | |
IgG | NA | NA | NA | NA | NA | NA | |
untargeted | 10 ± 2 | 4.1 | 3.4*,+ | NA | 4 | 12 | |
MDA2- intracellular | 45 | ||||||
Mn dendrimers | r1 | %NENH, 3 T | 48 h | ||||
MDA2 | 13 ± 1 | 3.5 | 1.9* | 0.9 | 11 | 62** | |
IK17 | NA | NA | NA | NA | NA | NA | 0.05-mmol Mn/kg |
E06 | NA | NA | NA | NA | NA | NA | |
IgG | NA | NA | NA | NA | NA | NA | |
Untargeted | 12 ± 1 | 3.4 | 0.8 | 0.8 | 1 | 16 | |
Iron Oxides | r2 | ΔR2 *(%) , 9.4 T | 24 h | ||||
MDA2 | 14 ± 3 | 37 | 9.01* | 1.55 | 31 | 56** | 3.9 mg Fe/kg |
IK17 | 12 ± 2 | 35 | 9.12 | NA | 31 | 58** | |
E06 | 16 ± 4 | 38 | 9.32 | NA | 30 | 62** | |
IgG | NA | NA | NA | NA | NA | NA | |
Untargeted | 10 ± 3 | 35 | 1.52 | 1.41 | 25 | 0.5 |
For nuclear imaging techniques such as PET and SPECT, probe design is focused on specificity, pharmacokinetics, biodistribution, and radioactive tracer half-life. Typically, an antibody or peptide is directly labeled with a radioactive tracer, and PET or SPECT imaging is performed once the antibody or peptide has cleared circulation, so that background signal from blood does not interfere with accurate imaging. When designing a probe for these modalities, it is critical that the antibody or peptide has a blood clearance that is faster than the half-life associated with the radioactive tracer. Additionally, the antibody must exhibit specificity to the target tissue. In the case of OSE imaging, the antibody or peptide can bind to and accumulate in the extracellular space and also can be taken up by the scavenger receptors associated with intra-plaque macrophages, but with limited uptake in tissue associated with the reticular endothelial system (RES, such as spleen and liver) and mononuclear phagocytic system (MPS, such as lymph, lung, and bone).
Preclinical studies using 125I-MDA2 were performed in atherosclerotic mice and rabbits, and imaging was performed 24 h after intravenous injection of the radiolabeled antibody [37, 44, 51], showing accumulation of 125I-MDA2 in atherosclerotic lesions, as confirmed by autoradiography, ex vivo imaging, lipid staining, and immunohistochemistry. The uptake of 125I-MDA2, evaluated as the percent of the injected dose determined by autoradiography, was highest in the aorta compared to all other tissues examined. Additionally, the uptake of 125I-MDA2 in the arterial wall was directly proportional to plaque burden, as measured by en face % plaque area as well as aortic weight which is an excellent surrogate of plaque burden, as measured by autoradiography (Fig. 6.3) [51].
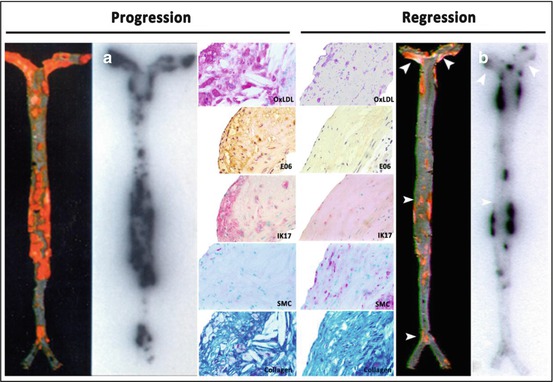
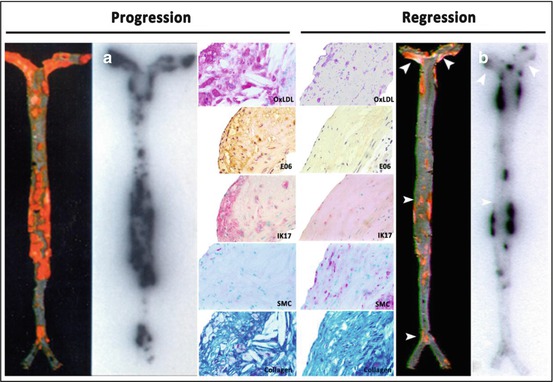
Fig. 6.3
Autoradiographic and immunohistochemistry representations of 125I-MDA2 accumulation in Sudan-stained aortas of LDLR−/−mice undergoing progression and regression diets. Representative en face mouse aortas stained with Sudan red and corresponding autoradiographs of 125I-MDA2 accumulation in mouse aortas following intravenous injection, in progression (a) and regression. (b) groups. Arrowheads display discordance between lipid stained plaque and 125I-MDA2 accumulation during dietary regression. Representative aortic atherosclerotic lesions from LDLR−/−mice from progression (left middle panel) and regression (right middle panel) groups. Immunostaining for OxLDL, SMCs, and IK17 appears pink/purple. Collagen is stained with Masson Trichrome and appears bright blue. E06 staining appears as brown product of peroxidase reaction (Reprinted with permission from Tsimikas et al. [51] and Torzewski et al. [53])
This initial approach also provided novel insights into the changes in OSE during plaque progression and regression induced by dietary changes in mice, rabbits, and monkeys in a series of experimental studies [51–53, 58]. It was observed that during atherosclerosis progression induced by western diet (high fat, high cholesterol), OSE deposition in the vessel wall is relatively proportional to plaque burden, with evidence of strong expression of several unique OSE, along with macrophage markers and reduced smooth muscle cell and collagen content. However, when the western diets were removed and the animal placed on a normal chow diet (typically ~5 % fat and no added cholesterol), atherosclerosis progression was either reduced or regression induced. However, despite the fact that many lesions were unchanged in size, there was evidence of significant loss of OSE and macrophages, along with substantial gain in smooth muscle cells and collagen (Fig. 6.3) [53], accepted markers of plaque stabilization [61]. Furthermore, there was evidence that with loss of OxPL in the vessel wall, OxPL/apoB in plasma were increased [58], suggesting, but not proving, a reverse flux of OxPL from the vessel wall to the circulation. Finally, reduced immunostaining for OSE with concomitantly reduced 125I-MDA2 uptake was noted in aged (~4 years old) Watanabe heritable hyperlipidemic rabbits with extensive (essentially 100 % of aortic surface area) atherosclerosis but with reduced OSE staining due to the presence of fibrotic and calcified lesions [53]. A clinical equivalent of this would be an obstructive, heavily calcified fibrotic lesion with reduced OSE content that would have a lower propensity to rupture [62]. If translated to clinical imaging, these observations suggest that loss of OSE may be a very early event in plaque stabilization, before any significant change in plaque size. Ultimately, linking such changes to clinical events would provide proof that this is a clinically relevant pathophysiological pathway for plaque surveillance.
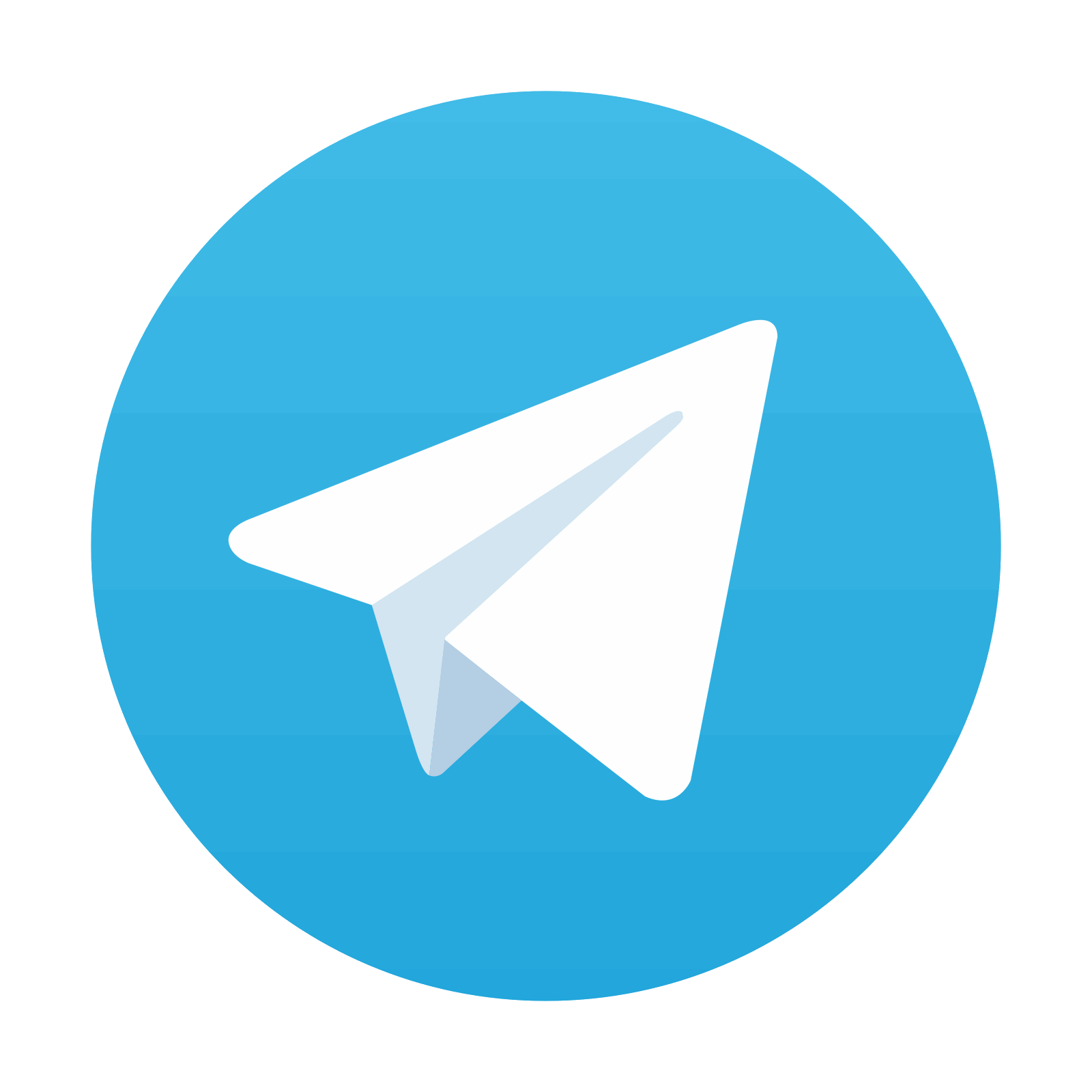
Stay updated, free articles. Join our Telegram channel

Full access? Get Clinical Tree
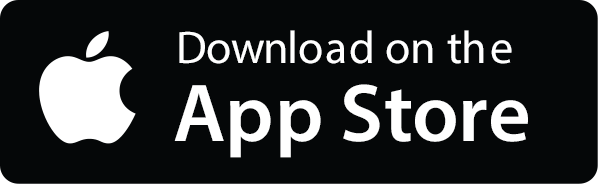
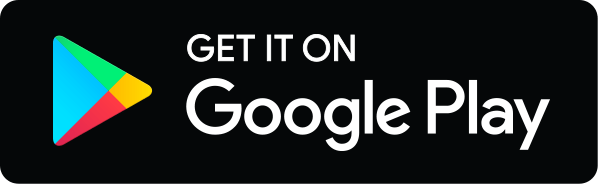